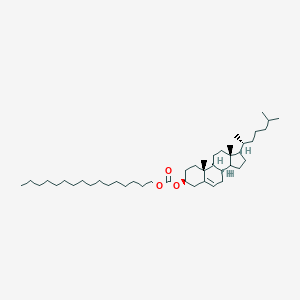
Cholesteryl hexadecyl carbonate
Übersicht
Beschreibung
Cholesteryl hexadecyl carbonate is a chemical compound with the molecular formula C44H78O3 . It is a type of cholesteryl ester .
Synthesis Analysis
The synthesis of cholesteryl esters like cholesteryl hexadecyl carbonate involves complex chemical procedures . These procedures often involve the use of cholesterol-based compounds and specific synthetic procedures to obtain new cholesterol derivatives .Molecular Structure Analysis
The molecular structure of cholesteryl hexadecyl carbonate is based on the structure of cholesterol, with additional components that give it its unique properties .Chemical Reactions Analysis
Cholesteryl esters participate in various chemical reactions. One of the challenges in analyzing these compounds is determining the locations of carbon-carbon double bonds within unsaturated fatty acyl chains . Techniques such as the Paternὸ-Büchi (PB) reaction can be used to determine these locations .Wissenschaftliche Forschungsanwendungen
Dielectric Studies
Dielectric studies provide an understanding of the electrical properties of materials interacting with an applied field . The dielectric and thermodynamic properties of cholesteryl oleyl carbonate (COC) liquid crystal are studied at 9.0 GHz using the cavity perturbation technique . The observed values of frequency shift and width of the resonance profile are analysed using the Slater perturbation equations for dielectric permittivity and dielectric loss .
Thermodynamic Analysis
The thermodynamic parameters including change in activation energy, enthalpy and entropy are calculated for heating and cooling cycles . The response of COC for heating and cooling cycles at 9.0 GHz shows fruitful results for both dielectric and thermodynamic parameters at transition temperatures .
Liquid Crystal Research
Liquid crystals (LCs) are ordered liquids, a state of matter which lies between the crystalline and the ordinary (isotropic) liquid phases . Because of their good tunability with microwave frequencies, liquid crystals are popular compounds for use in the fabrication of tunable filters, phase shifters, reflect-arrays, and microcapsules .
Optical Switching
The LC shows optical switching characteristics which are used in thermochromic devices, pressure sensors, electrical signal indicators, and radiation detectors .
Broadband Reflection
Cholesteric liquid crystal (ChLC) materials with broadband reflection are witnessing a significant surge in interest due to their unique ability to self-organize into a helical supra-molecular architecture and their excellent selective reflection of light based on the Bragg relationship .
Self-Assembled Nanostructures
By building self-organized nanostructures with pitch gradient or non-uniform pitch distribution, extensive work has already been performed to obtain ChLC films with a broad reflection band .
Wirkmechanismus
Target of Action
The primary target of Cholesteryl Hexadecyl Carbonate is Cholesteryl Ester Transfer Protein (CETP) . CETP is a crucial protein that regulates plasma cholesterol levels by transferring cholesteryl esters (CEs) among lipoproteins . It plays a key role in reverse cholesterol transport (RCT) by mediating the transfer of cholesteryl esters (CEs) and triglycerides (TGs) between high-density lipoproteins (HDLs) and apolipoprotein B-100 (apoB-100) containing lipoproteins in plasma .
Mode of Action
Cholesteryl Hexadecyl Carbonate interacts with its target, CETP, and inhibits its function . The inhibition of CETP activity leads to a decrease in the transfer of cholesteryl esters from HDL to low-density lipoproteins (LDL), thereby reducing the amount of cholesterol esters transferred into the bloodstream . This action ultimately slows the progression of atherosclerotic cardiovascular disease (ASCVD) .
Biochemical Pathways
The action of Cholesteryl Hexadecyl Carbonate affects the cholesterol homeostasis pathway . By inhibiting CETP, it disrupts the normal transfer of cholesteryl esters and triglycerides between lipoproteins, which is a key process in the regulation of cholesterol levels in the body . This disruption can lead to changes in the concentrations of LDL and HDL cholesterol in the plasma, which are important factors in the development of ASCVD .
Pharmacokinetics
Related lipid nanoparticles have been shown to have a plasmatic half-life of approximately 30 minutes . These nanoparticles displayed a similar nanoparticle-driven biodistribution, indicative of their integrity during the first hours after injection . More research is needed to fully understand the ADME properties of Cholesteryl Hexadecyl Carbonate and their impact on its bioavailability.
Result of Action
The inhibition of CETP by Cholesteryl Hexadecyl Carbonate results in a decrease in the transfer of cholesteryl esters from HDL to LDL . This can lead to a reduction in the amount of cholesterol esters transferred into the bloodstream, which can slow the progression of ASCVD . .
Action Environment
The action, efficacy, and stability of Cholesteryl Hexadecyl Carbonate can be influenced by various environmental factors. For instance, the presence of other lipids and proteins in the plasma can affect the interaction of Cholesteryl Hexadecyl Carbonate with its target, CETP . Additionally, factors such as pH and temperature could potentially influence the stability and activity of Cholesteryl Hexadecyl Carbonate.
Zukünftige Richtungen
Research on cholesteryl esters like cholesteryl hexadecyl carbonate is ongoing, with a focus on understanding their synthesis, structure-property relationships, and applications . Future research may also explore the development of more effective inhibitors that can regulate the activity of cholesteryl ester transfer proteins .
Eigenschaften
IUPAC Name |
[(3S,8S,9S,10R,13R,14S,17R)-10,13-dimethyl-17-[(2R)-6-methylheptan-2-yl]-2,3,4,7,8,9,11,12,14,15,16,17-dodecahydro-1H-cyclopenta[a]phenanthren-3-yl] hexadecyl carbonate | |
---|---|---|
Source | PubChem | |
URL | https://pubchem.ncbi.nlm.nih.gov | |
Description | Data deposited in or computed by PubChem | |
InChI |
InChI=1S/C44H78O3/c1-7-8-9-10-11-12-13-14-15-16-17-18-19-20-32-46-42(45)47-37-28-30-43(5)36(33-37)24-25-38-40-27-26-39(35(4)23-21-22-34(2)3)44(40,6)31-29-41(38)43/h24,34-35,37-41H,7-23,25-33H2,1-6H3/t35-,37+,38+,39-,40+,41+,43+,44-/m1/s1 | |
Source | PubChem | |
URL | https://pubchem.ncbi.nlm.nih.gov | |
Description | Data deposited in or computed by PubChem | |
InChI Key |
GCKXFAZCNWKZAW-BFGJSWSOSA-N | |
Source | PubChem | |
URL | https://pubchem.ncbi.nlm.nih.gov | |
Description | Data deposited in or computed by PubChem | |
Canonical SMILES |
CCCCCCCCCCCCCCCCOC(=O)OC1CCC2(C3CCC4(C(C3CC=C2C1)CCC4C(C)CCCC(C)C)C)C | |
Source | PubChem | |
URL | https://pubchem.ncbi.nlm.nih.gov | |
Description | Data deposited in or computed by PubChem | |
Isomeric SMILES |
CCCCCCCCCCCCCCCCOC(=O)O[C@H]1CC[C@@]2([C@H]3CC[C@]4([C@H]([C@@H]3CC=C2C1)CC[C@@H]4[C@H](C)CCCC(C)C)C)C | |
Source | PubChem | |
URL | https://pubchem.ncbi.nlm.nih.gov | |
Description | Data deposited in or computed by PubChem | |
Molecular Formula |
C44H78O3 | |
Source | PubChem | |
URL | https://pubchem.ncbi.nlm.nih.gov | |
Description | Data deposited in or computed by PubChem | |
Molecular Weight |
655.1 g/mol | |
Source | PubChem | |
URL | https://pubchem.ncbi.nlm.nih.gov | |
Description | Data deposited in or computed by PubChem | |
Product Name |
Cholesteryl hexadecyl carbonate |
Retrosynthesis Analysis
AI-Powered Synthesis Planning: Our tool employs the Template_relevance Pistachio, Template_relevance Bkms_metabolic, Template_relevance Pistachio_ringbreaker, Template_relevance Reaxys, Template_relevance Reaxys_biocatalysis model, leveraging a vast database of chemical reactions to predict feasible synthetic routes.
One-Step Synthesis Focus: Specifically designed for one-step synthesis, it provides concise and direct routes for your target compounds, streamlining the synthesis process.
Accurate Predictions: Utilizing the extensive PISTACHIO, BKMS_METABOLIC, PISTACHIO_RINGBREAKER, REAXYS, REAXYS_BIOCATALYSIS database, our tool offers high-accuracy predictions, reflecting the latest in chemical research and data.
Strategy Settings
Precursor scoring | Relevance Heuristic |
---|---|
Min. plausibility | 0.01 |
Model | Template_relevance |
Template Set | Pistachio/Bkms_metabolic/Pistachio_ringbreaker/Reaxys/Reaxys_biocatalysis |
Top-N result to add to graph | 6 |
Feasible Synthetic Routes
Haftungsausschluss und Informationen zu In-Vitro-Forschungsprodukten
Bitte beachten Sie, dass alle Artikel und Produktinformationen, die auf BenchChem präsentiert werden, ausschließlich zu Informationszwecken bestimmt sind. Die auf BenchChem zum Kauf angebotenen Produkte sind speziell für In-vitro-Studien konzipiert, die außerhalb lebender Organismen durchgeführt werden. In-vitro-Studien, abgeleitet von dem lateinischen Begriff "in Glas", beinhalten Experimente, die in kontrollierten Laborumgebungen unter Verwendung von Zellen oder Geweben durchgeführt werden. Es ist wichtig zu beachten, dass diese Produkte nicht als Arzneimittel oder Medikamente eingestuft sind und keine Zulassung der FDA für die Vorbeugung, Behandlung oder Heilung von medizinischen Zuständen, Beschwerden oder Krankheiten erhalten haben. Wir müssen betonen, dass jede Form der körperlichen Einführung dieser Produkte in Menschen oder Tiere gesetzlich strikt untersagt ist. Es ist unerlässlich, sich an diese Richtlinien zu halten, um die Einhaltung rechtlicher und ethischer Standards in Forschung und Experiment zu gewährleisten.