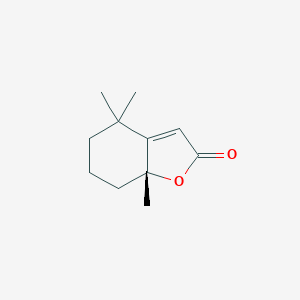
Dihydroactinidiolide
Übersicht
Beschreibung
Dihydroactinidiolide is a volatile terpene with a sweet, tea-like odor. It is used as a fragrance and occurs naturally in black tea, fenugreek, fire ants, mangoes, and tobacco . It has also been prepared synthetically . Dihydroactinidiolide is a pheromone for a variety of insects .
Synthesis Analysis
The total synthesis of Dihydroactinidiolide has been achieved using Asymmetric Catalytic Hetero-Diels-Alder Methodology . The key step in the syntheses is the copper(II)-bisoxazoline-catalyzed hetero-Diels-Alder reaction of a cyclic diene with ethyl glyoxylate .Molecular Structure Analysis
Dihydroactinidiolide has a molecular formula of C11H16O2 . It is a bicyclic lactone . The structure contains a carbonyl group that can react with nucleophilic structures in macromolecules .Chemical Reactions Analysis
The formation of Dihydroactinidiolide by thermal degradation of β-carotene has been studied . A comparison of yields of Dihydroactinidiolide in commercial β-carotene and β-carotene derived from crude palm oil was investigated .Physical And Chemical Properties Analysis
Dihydroactinidiolide has a molar mass of 180.24 g/mol . It has a density of 1.1±0.1 g/cm3, a boiling point of 296.1±9.0 °C at 760 mmHg, and a vapour pressure of 0.0±0.6 mmHg at 25°C .Wissenschaftliche Forschungsanwendungen
Growth Inhibition
Dihydroactinidiolide has been isolated from Eleocharis coloradoensis, along with p-coumaric acid, ferulic acid, and luteolin, and shown to be a potent phytotoxin at low concentration . It acts as a potent growth inhibitor, making it a subject of interest in the study of plant growth and development .
Regulation of Gene Expression and Photoacclimation
Dihydroactinidiolide is a high light-induced β-Carotene derivative that can regulate gene expression and photoacclimation in Arabidopsis . This compound is produced during high light stress and induces changes in the expression of 1O2-responsive genes . The β-cyclocitral-dependent gene reprogramming was associated with an increased tolerance of the plants to photooxidative stress .
Bioactive Molecule in Animals
Dihydroactinidiolide is known to be a bioactive molecule in animals. It is a component of pheromones in insects, such as red fire ants, and in mammals such as the Cat and the Red Fox .
Cytotoxic Effects Against Cancer Cell Lines
Dihydroactinidiolide has been found to exhibit cytotoxic effects against cancer cell lines . This makes it a potential candidate for further research in cancer treatment.
Seed Germination and Seedling Growth Inhibition
Dihydroactinidiolide was identified as a major component of ethyl acetate extracts of cyanobacteria or aquatic macrophytes which inhibit seed germination and seedling growth . This property could be useful in controlling the growth of certain plants.
Potential Use in Agriculture
While not directly related to Dihydroactinidiolide, carotenoid-derived nanoparticles, which Dihydroactinidiolide is a derivative of, have diverse applications in biomedical, electrochemical, and environment but their use in agriculture, i.e., elicitation and nano-fertilizer is limited . More areas for nanoparticle application can be explored for future research .
Wirkmechanismus
Target of Action
Dihydroactinidiolide (DA) is a volatile terpene that has been found to interact with a variety of targets. It is known to be a bioactive molecule in animals, acting as a component of pheromones in insects such as red fire ants . In plants, it has been suggested to play a role in the regulation of gene expression and photoacclimation .
Mode of Action
It is known that da can react with nucleophilic structures in macromolecules due to its carbonyl group, providing this compound with a high potential reactivity . This suggests that DA may interact with its targets through chemical reactions, leading to changes in the targets’ function or behavior.
Biochemical Pathways
DA is a derivative of β-carotene, produced through the secondary oxidation of β-ionone . It has been found to accumulate in Arabidopsis leaves under high light stress , suggesting that it may be involved in the plant’s response to light stress.
Pharmacokinetics
It has been suggested that da has suitable adme properties which prove its druglikeness
Result of Action
DA has been found to exhibit various effects at the molecular and cellular level. For example, it has been found to exhibit cytotoxic effects against cancer cell lines . In plants, it has been suggested to regulate gene expression and photoacclimation . In animals, it acts as a component of pheromones, influencing the behavior of other animals .
Action Environment
The action of DA can be influenced by environmental factors. For example, it has been found to accumulate in Arabidopsis leaves under high light stress , suggesting that light conditions can influence its production and action. Furthermore, the action of DA as a pheromone in insects suggests that it may be influenced by the presence of other animals .
Safety and Hazards
Zukünftige Richtungen
Dihydroactinidiolide has been identified as a major component of ethyl acetate extracts of cyanobacteria or aquatic macrophytes, which inhibit seed germination and seedling growth . It also has been identified in wheat glumes, which acts as a germination inhibitor . The current findings demand for further in vitro and in vivo studies to develop Dihydroactinidiolide as a multi-target lead against Alzheimer’s disease .
Eigenschaften
IUPAC Name |
4,4,7a-trimethyl-6,7-dihydro-5H-1-benzofuran-2-one | |
---|---|---|
Source | PubChem | |
URL | https://pubchem.ncbi.nlm.nih.gov | |
Description | Data deposited in or computed by PubChem | |
InChI |
InChI=1S/C11H16O2/c1-10(2)5-4-6-11(3)8(10)7-9(12)13-11/h7H,4-6H2,1-3H3 | |
Source | PubChem | |
URL | https://pubchem.ncbi.nlm.nih.gov | |
Description | Data deposited in or computed by PubChem | |
InChI Key |
IMKHDCBNRDRUEB-UHFFFAOYSA-N | |
Source | PubChem | |
URL | https://pubchem.ncbi.nlm.nih.gov | |
Description | Data deposited in or computed by PubChem | |
Canonical SMILES |
CC1(CCCC2(C1=CC(=O)O2)C)C | |
Source | PubChem | |
URL | https://pubchem.ncbi.nlm.nih.gov | |
Description | Data deposited in or computed by PubChem | |
Molecular Formula |
C11H16O2 | |
Source | PubChem | |
URL | https://pubchem.ncbi.nlm.nih.gov | |
Description | Data deposited in or computed by PubChem | |
DSSTOX Substance ID |
DTXSID00864588 | |
Record name | 2(4H)-Benzofuranone, 5,6,7,7a-tetrahydro-4,4,7a-trimethyl- | |
Source | EPA DSSTox | |
URL | https://comptox.epa.gov/dashboard/DTXSID00864588 | |
Description | DSSTox provides a high quality public chemistry resource for supporting improved predictive toxicology. | |
Molecular Weight |
180.24 g/mol | |
Source | PubChem | |
URL | https://pubchem.ncbi.nlm.nih.gov | |
Description | Data deposited in or computed by PubChem | |
Physical Description |
Clear, colourless liquid; musky or coumarin-like aroma | |
Record name | (+\/-)-(2,6,6,-Trimethyl-2-hydroxycyclohexylidene)acetic acid gamma-lactone | |
Source | Joint FAO/WHO Expert Committee on Food Additives (JECFA) | |
URL | https://www.fao.org/food/food-safety-quality/scientific-advice/jecfa/jecfa-flav/details/en/c/1155/ | |
Description | The flavoring agent databse provides the most recent specifications for flavorings evaluated by JECFA. | |
Explanation | Permission from WHO is not required for the use of WHO materials issued under the Creative Commons Attribution-NonCommercial-ShareAlike 3.0 Intergovernmental Organization (CC BY-NC-SA 3.0 IGO) licence. | |
Boiling Point |
295.00 to 296.00 °C. @ 760.00 mm Hg | |
Record name | Dihydroactinidiolide | |
Source | Human Metabolome Database (HMDB) | |
URL | http://www.hmdb.ca/metabolites/HMDB0035240 | |
Description | The Human Metabolome Database (HMDB) is a freely available electronic database containing detailed information about small molecule metabolites found in the human body. | |
Explanation | HMDB is offered to the public as a freely available resource. Use and re-distribution of the data, in whole or in part, for commercial purposes requires explicit permission of the authors and explicit acknowledgment of the source material (HMDB) and the original publication (see the HMDB citing page). We ask that users who download significant portions of the database cite the HMDB paper in any resulting publications. | |
Solubility |
Insoluble in water; soluble in nonpolar solvents, soluble (in ethanol) | |
Record name | (+\/-)-(2,6,6,-Trimethyl-2-hydroxycyclohexylidene)acetic acid gamma-lactone | |
Source | Joint FAO/WHO Expert Committee on Food Additives (JECFA) | |
URL | https://www.fao.org/food/food-safety-quality/scientific-advice/jecfa/jecfa-flav/details/en/c/1155/ | |
Description | The flavoring agent databse provides the most recent specifications for flavorings evaluated by JECFA. | |
Explanation | Permission from WHO is not required for the use of WHO materials issued under the Creative Commons Attribution-NonCommercial-ShareAlike 3.0 Intergovernmental Organization (CC BY-NC-SA 3.0 IGO) licence. | |
Density |
1.051-1.058 | |
Record name | (+\/-)-(2,6,6,-Trimethyl-2-hydroxycyclohexylidene)acetic acid gamma-lactone | |
Source | Joint FAO/WHO Expert Committee on Food Additives (JECFA) | |
URL | https://www.fao.org/food/food-safety-quality/scientific-advice/jecfa/jecfa-flav/details/en/c/1155/ | |
Description | The flavoring agent databse provides the most recent specifications for flavorings evaluated by JECFA. | |
Explanation | Permission from WHO is not required for the use of WHO materials issued under the Creative Commons Attribution-NonCommercial-ShareAlike 3.0 Intergovernmental Organization (CC BY-NC-SA 3.0 IGO) licence. | |
CAS RN |
15356-74-8, 17092-92-1 | |
Record name | 5,6,7,7a-Tetrahydro-4,4,7a-trimethyl-2(4H)-benzofuranone | |
Source | CAS Common Chemistry | |
URL | https://commonchemistry.cas.org/detail?cas_rn=15356-74-8 | |
Description | CAS Common Chemistry is an open community resource for accessing chemical information. Nearly 500,000 chemical substances from CAS REGISTRY cover areas of community interest, including common and frequently regulated chemicals, and those relevant to high school and undergraduate chemistry classes. This chemical information, curated by our expert scientists, is provided in alignment with our mission as a division of the American Chemical Society. | |
Explanation | The data from CAS Common Chemistry is provided under a CC-BY-NC 4.0 license, unless otherwise stated. | |
Record name | Dihydroactinolide | |
Source | ChemIDplus | |
URL | https://pubchem.ncbi.nlm.nih.gov/substance/?source=chemidplus&sourceid=0015356748 | |
Description | ChemIDplus is a free, web search system that provides access to the structure and nomenclature authority files used for the identification of chemical substances cited in National Library of Medicine (NLM) databases, including the TOXNET system. | |
Record name | DIHYDROACTINIDIOLIDE | |
Source | DTP/NCI | |
URL | https://dtp.cancer.gov/dtpstandard/servlet/dwindex?searchtype=NSC&outputformat=html&searchlist=357087 | |
Description | The NCI Development Therapeutics Program (DTP) provides services and resources to the academic and private-sector research communities worldwide to facilitate the discovery and development of new cancer therapeutic agents. | |
Explanation | Unless otherwise indicated, all text within NCI products is free of copyright and may be reused without our permission. Credit the National Cancer Institute as the source. | |
Record name | 2(4H)-Benzofuranone, 5,6,7,7a-tetrahydro-4,4,7a-trimethyl- | |
Source | EPA Chemicals under the TSCA | |
URL | https://www.epa.gov/chemicals-under-tsca | |
Description | EPA Chemicals under the Toxic Substances Control Act (TSCA) collection contains information on chemicals and their regulations under TSCA, including non-confidential content from the TSCA Chemical Substance Inventory and Chemical Data Reporting. | |
Record name | 2(4H)-Benzofuranone, 5,6,7,7a-tetrahydro-4,4,7a-trimethyl- | |
Source | EPA DSSTox | |
URL | https://comptox.epa.gov/dashboard/DTXSID00864588 | |
Description | DSSTox provides a high quality public chemistry resource for supporting improved predictive toxicology. | |
Record name | 5,6,7,7a-tetrahydro-4,4,7a-trimethylbenzofuran-2(4H)-one | |
Source | European Chemicals Agency (ECHA) | |
URL | https://echa.europa.eu/substance-information/-/substanceinfo/100.035.794 | |
Description | The European Chemicals Agency (ECHA) is an agency of the European Union which is the driving force among regulatory authorities in implementing the EU's groundbreaking chemicals legislation for the benefit of human health and the environment as well as for innovation and competitiveness. | |
Explanation | Use of the information, documents and data from the ECHA website is subject to the terms and conditions of this Legal Notice, and subject to other binding limitations provided for under applicable law, the information, documents and data made available on the ECHA website may be reproduced, distributed and/or used, totally or in part, for non-commercial purposes provided that ECHA is acknowledged as the source: "Source: European Chemicals Agency, http://echa.europa.eu/". Such acknowledgement must be included in each copy of the material. ECHA permits and encourages organisations and individuals to create links to the ECHA website under the following cumulative conditions: Links can only be made to webpages that provide a link to the Legal Notice page. | |
Record name | Dihydroactinidiolide | |
Source | European Chemicals Agency (ECHA) | |
URL | https://echa.europa.eu/information-on-chemicals | |
Description | The European Chemicals Agency (ECHA) is an agency of the European Union which is the driving force among regulatory authorities in implementing the EU's groundbreaking chemicals legislation for the benefit of human health and the environment as well as for innovation and competitiveness. | |
Explanation | Use of the information, documents and data from the ECHA website is subject to the terms and conditions of this Legal Notice, and subject to other binding limitations provided for under applicable law, the information, documents and data made available on the ECHA website may be reproduced, distributed and/or used, totally or in part, for non-commercial purposes provided that ECHA is acknowledged as the source: "Source: European Chemicals Agency, http://echa.europa.eu/". Such acknowledgement must be included in each copy of the material. ECHA permits and encourages organisations and individuals to create links to the ECHA website under the following cumulative conditions: Links can only be made to webpages that provide a link to the Legal Notice page. | |
Record name | DIHYDROACTINIDIOLIDE, (±)- | |
Source | FDA Global Substance Registration System (GSRS) | |
URL | https://gsrs.ncats.nih.gov/ginas/app/beta/substances/BH8469LVA9 | |
Description | The FDA Global Substance Registration System (GSRS) enables the efficient and accurate exchange of information on what substances are in regulated products. Instead of relying on names, which vary across regulatory domains, countries, and regions, the GSRS knowledge base makes it possible for substances to be defined by standardized, scientific descriptions. | |
Explanation | Unless otherwise noted, the contents of the FDA website (www.fda.gov), both text and graphics, are not copyrighted. They are in the public domain and may be republished, reprinted and otherwise used freely by anyone without the need to obtain permission from FDA. Credit to the U.S. Food and Drug Administration as the source is appreciated but not required. | |
Record name | Dihydroactinidiolide | |
Source | Human Metabolome Database (HMDB) | |
URL | http://www.hmdb.ca/metabolites/HMDB0035240 | |
Description | The Human Metabolome Database (HMDB) is a freely available electronic database containing detailed information about small molecule metabolites found in the human body. | |
Explanation | HMDB is offered to the public as a freely available resource. Use and re-distribution of the data, in whole or in part, for commercial purposes requires explicit permission of the authors and explicit acknowledgment of the source material (HMDB) and the original publication (see the HMDB citing page). We ask that users who download significant portions of the database cite the HMDB paper in any resulting publications. | |
Retrosynthesis Analysis
AI-Powered Synthesis Planning: Our tool employs the Template_relevance Pistachio, Template_relevance Bkms_metabolic, Template_relevance Pistachio_ringbreaker, Template_relevance Reaxys, Template_relevance Reaxys_biocatalysis model, leveraging a vast database of chemical reactions to predict feasible synthetic routes.
One-Step Synthesis Focus: Specifically designed for one-step synthesis, it provides concise and direct routes for your target compounds, streamlining the synthesis process.
Accurate Predictions: Utilizing the extensive PISTACHIO, BKMS_METABOLIC, PISTACHIO_RINGBREAKER, REAXYS, REAXYS_BIOCATALYSIS database, our tool offers high-accuracy predictions, reflecting the latest in chemical research and data.
Strategy Settings
Precursor scoring | Relevance Heuristic |
---|---|
Min. plausibility | 0.01 |
Model | Template_relevance |
Template Set | Pistachio/Bkms_metabolic/Pistachio_ringbreaker/Reaxys/Reaxys_biocatalysis |
Top-N result to add to graph | 6 |
Feasible Synthetic Routes
Q & A
Q1: What is the molecular formula and weight of dihydroactinidiolide?
A1: Dihydroactinidiolide has a molecular formula of C11H16O2 and a molecular weight of 180.24 g/mol.
Q2: Are there any spectroscopic data available for dihydroactinidiolide?
A2: Yes, researchers have used various spectroscopic techniques to characterize dihydroactinidiolide. These include infrared spectroscopy (IR), gas chromatography-mass spectrometry (GC-MS), and nuclear magnetic resonance spectroscopy (NMR). [, , , , , ]
Q3: Can you describe common synthetic routes for dihydroactinidiolide?
A3: Dihydroactinidiolide can be synthesized through several pathways, often starting from readily available compounds. Some methods involve the use of β-ionone as a precursor, utilizing reagents like peroxyacetic acid and silicotungstic acid. [] Other approaches utilize citral, employing steps such as cyclization, cyanation, dehydration, and hydrolysis. [, ] Additionally, asymmetric synthesis routes have been developed using chiral starting materials like (R)-2-hydroxy-2-methylcyclohexanone and employing reactions like cerium enolate chemistry or copper(II)-bisoxazoline-catalyzed hetero-Diels-Alder reactions. [, , ]
Q4: What are the natural sources of dihydroactinidiolide?
A4: Dihydroactinidiolide is found naturally in various plants. Significant sources include:
- Tea: Black tea, green tea, yellow tea, and Pu-erh tea have been reported to contain dihydroactinidiolide. [, , , , , ]
- Other plants: Artemisia lactiflora, Osmanthus fragrans, Trollius chinensis, persimmon leaves (Diospyros kaki), and Fucus vesiculosus (brown algae) also contain dihydroactinidiolide. [, , , , , ]
- Fungi: Certain fungi, like Ganoderma applanatum, Hypomyces odoratus, Kuehneromyces mutabilis, and Trametes suaveolens, can produce dihydroactinidiolide through the degradation of β-carotene. []
Q5: How is dihydroactinidiolide formed in nature?
A5: Dihydroactinidiolide is often formed as a degradation product of β-carotene. This process can occur through various mechanisms:
- Thermal degradation: Heating β-carotene, such as during the roasting of green tea, can lead to the formation of dihydroactinidiolide. [, ]
- Oxidative degradation: Exposure of β-carotene to oxidizing agents can also result in dihydroactinidiolide formation. [, ]
- Enzymatic degradation: Certain microorganisms possess enzymes, such as carotenoid cleavage oxygenases, capable of breaking down β-carotene into dihydroactinidiolide and other compounds. [, , ]
Q6: Does dihydroactinidiolide have any known biological activities?
A6: Yes, dihydroactinidiolide has been reported to exhibit various biological activities:
- Antimetastatic activity: Research suggests that dihydroactinidiolide inhibits the migration and invasion of non-small cell lung cancer (NSCLC) cells in vitro, potentially by downregulating N-cadherin. []
- Allelopathic activity: Dihydroactinidiolide has been identified as an allelochemical, inhibiting the germination and growth of certain plant species, such as Chinese amaranth and barnyard grass. []
- Germination inhibitor: Studies have shown that dihydroactinidiolide can act as a germination inhibitor in wheat grains, potentially contributing to seed dormancy. [, ]
- Pheromone activity: In animals, dihydroactinidiolide is a known component of pheromones in insects and mammals. []
Q7: What are the potential applications of dihydroactinidiolide?
A7: Due to its aroma and biological properties, dihydroactinidiolide holds potential applications in various industries:
- Food industry: As a flavoring agent, dihydroactinidiolide contributes to the characteristic aroma of certain foods and beverages, particularly tea. [, , , , ]
- Fragrance industry: The pleasant, often fruity, odor of dihydroactinidiolide makes it valuable for perfumes and cosmetics. [, ]
- Agriculture: Its allelopathic properties suggest potential applications in developing bio-herbicides or weed control strategies. []
- Pharmaceuticals: The antimetastatic activity of dihydroactinidiolide warrants further investigation for potential anticancer therapies. []
Q8: What analytical techniques are used to identify and quantify dihydroactinidiolide?
A8: Several analytical techniques are employed:
- Gas Chromatography-Mass Spectrometry (GC-MS): This is the most common method for separating, identifying, and quantifying dihydroactinidiolide in complex mixtures. [, , , , , , , ]
- High-Performance Liquid Chromatography (HPLC): HPLC can also be used to separate and quantify dihydroactinidiolide, particularly when coupled with UV-Vis detection. []
Q9: How do researchers ensure the accuracy and reliability of dihydroactinidiolide analysis?
A9: Analytical method validation is crucial for ensuring accurate and reliable results. This often involves:
- Use of standards: Comparison with authentic dihydroactinidiolide standards helps in confirming the identity and quantifying the compound. []
- Method optimization: Careful selection of extraction methods, chromatographic columns, and detection parameters ensures optimal separation and detection sensitivity. [, ]
- Quality control: Implementing appropriate quality control measures, like using internal standards and performing replicate analyses, helps in minimizing errors and ensuring data reliability. []
Q10: What are some of the future research directions for dihydroactinidiolide?
A11:
Q11: How does the concentration of dihydroactinidiolide vary in different tea types and cultivars?
A12: The concentration of dihydroactinidiolide varies significantly depending on the tea type, cultivar, processing methods, and geographical origin. For instance, some studies show higher levels in aged Pu-erh tea compared to raw or ripe Pu-erh tea. [] Additionally, the cultivars used for Wuyi Rock tea demonstrate distinct volatile profiles, influencing the relative abundance of dihydroactinidiolide. []
Haftungsausschluss und Informationen zu In-Vitro-Forschungsprodukten
Bitte beachten Sie, dass alle Artikel und Produktinformationen, die auf BenchChem präsentiert werden, ausschließlich zu Informationszwecken bestimmt sind. Die auf BenchChem zum Kauf angebotenen Produkte sind speziell für In-vitro-Studien konzipiert, die außerhalb lebender Organismen durchgeführt werden. In-vitro-Studien, abgeleitet von dem lateinischen Begriff "in Glas", beinhalten Experimente, die in kontrollierten Laborumgebungen unter Verwendung von Zellen oder Geweben durchgeführt werden. Es ist wichtig zu beachten, dass diese Produkte nicht als Arzneimittel oder Medikamente eingestuft sind und keine Zulassung der FDA für die Vorbeugung, Behandlung oder Heilung von medizinischen Zuständen, Beschwerden oder Krankheiten erhalten haben. Wir müssen betonen, dass jede Form der körperlichen Einführung dieser Produkte in Menschen oder Tiere gesetzlich strikt untersagt ist. Es ist unerlässlich, sich an diese Richtlinien zu halten, um die Einhaltung rechtlicher und ethischer Standards in Forschung und Experiment zu gewährleisten.