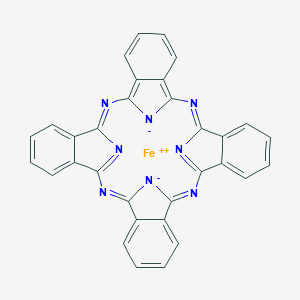
Phthalocyanine Iron(II)
Übersicht
Beschreibung
Iron(II) phthalocyanine (FePc) is a macrocyclic complex consisting of a central iron(II) ion coordinated to four nitrogen atoms within a conjugated 18-π-electron phthalocyanine ligand. This planar structure enables unique electronic and catalytic properties, including strong absorption in the visible spectrum, redox versatility, and stability under harsh conditions . FePc is widely studied for applications in electrocatalysis (e.g., oxygen reduction reaction, ORR), organic electronics, and photodynamic therapy . Its catalytic activity arises from the Fe(II/III) redox couple, which facilitates electron transfer in reactions like ORR and olefination .
Wirkmechanismus
Target of Action
Iron(II) phthalocyanine (FePc) primarily targets the oxygen reduction reaction (ORR) . This reaction is crucial for the energy efficiency of fuel cells and metal-air batteries . FePc is also known to catalyze intramolecular C(sp3)–H bond amination with alkyl azides .
Mode of Action
FePc interacts with its targets through its unique FeN4 active site . The FeN4 site usually exhibits poor O2 adsorption and activation due to its plane-symmetric structure . An axial fe–o coordination strategy can improve its o2 adsorption, activation, and thus the orr performance . The Fe–O coordination evokes electronic localization among the axial direction of O–FeN4 sites to enhance O2 adsorption and activation .
Biochemical Pathways
The primary biochemical pathway affected by FePc is the oxygen reduction reaction (ORR) . This reaction directly determines the energy efficiency of fuel cells and metal-air batteries . FePc enhances the ORR by improving O2 adsorption and activation .
Pharmacokinetics
Without axial ligands, FePc aggregates leading to solids of low solubility . A soluble iron(ii)-phthalocyanine derivative with bulky substituents can prevent aggregation and improve solubility .
Result of Action
The primary result of FePc’s action is the enhancement of the oxygen reduction reaction (ORR) . The catalyst obtained from FePc exhibits fast kinetics for O2 adsorption and activation . It also shows a remarkable half-wave potential of 0.90V . In addition, FePc can catalyze intramolecular C(sp3)–H bond amination with alkyl azides .
Action Environment
The action of FePc can be influenced by environmental factors such as the presence of axial ligands and the type of carbon support used . For instance, without axial ligands, FePc forms solids with low solubility . When coordinated with an oxidized carbon, fepc exhibits improved o2 adsorption and activation . Furthermore, the type of carbon support (e.g., carbon nanotube or black pearl) can affect the electrocatalytic activity of FePc .
Biochemische Analyse
Biochemical Properties
Iron(II) phthalocyanine plays a crucial role in biochemical reactions, particularly in redox processes. It interacts with various enzymes and proteins, acting as a catalyst in oxidation-reduction reactions. One notable interaction is with cytochrome P450 enzymes, where Iron(II) phthalocyanine mimics the heme group, facilitating electron transfer and catalytic activity. Additionally, it interacts with oxygenases and peroxidases, enhancing their catalytic efficiency in oxidative reactions .
Cellular Effects
Iron(II) phthalocyanine influences various cellular processes, including cell signaling pathways, gene expression, and cellular metabolism. It has been shown to affect the activity of reactive oxygen species (ROS) within cells, thereby modulating oxidative stress responses. This compound can also influence the expression of genes involved in antioxidant defense mechanisms, such as superoxide dismutase and catalase. Furthermore, Iron(II) phthalocyanine impacts cellular metabolism by altering the activity of key metabolic enzymes, leading to changes in energy production and utilization .
Molecular Mechanism
At the molecular level, Iron(II) phthalocyanine exerts its effects through several mechanisms. It binds to biomolecules, including proteins and nucleic acids, through coordination interactions with its central iron ion. This binding can result in enzyme inhibition or activation, depending on the specific target. For example, Iron(II) phthalocyanine can inhibit the activity of certain oxidases by competing with their natural substrates. Additionally, it can induce changes in gene expression by interacting with transcription factors and other regulatory proteins .
Temporal Effects in Laboratory Settings
In laboratory settings, the effects of Iron(II) phthalocyanine can change over time due to its stability and degradation properties. Studies have shown that this compound is relatively stable under physiological conditions but can undergo degradation in the presence of strong oxidizing agents. Long-term exposure to Iron(II) phthalocyanine has been observed to cause cumulative effects on cellular function, including alterations in cell proliferation and apoptosis rates. These temporal effects are crucial for understanding the long-term implications of using this compound in biomedical applications .
Dosage Effects in Animal Models
The effects of Iron(II) phthalocyanine vary with different dosages in animal models. At low doses, it has been found to enhance antioxidant defenses and improve metabolic efficiency. At high doses, Iron(II) phthalocyanine can exhibit toxic effects, including oxidative damage to cellular components and disruption of normal cellular functions. Threshold effects have been observed, where a specific dosage range is required to achieve the desired therapeutic effects without causing adverse reactions .
Metabolic Pathways
Iron(II) phthalocyanine is involved in several metabolic pathways, primarily those related to oxidative metabolism. It interacts with enzymes such as cytochrome P450 and peroxidases, influencing the metabolic flux of substrates through these pathways. Additionally, Iron(II) phthalocyanine can affect the levels of metabolites involved in redox reactions, such as NADH and FADH2, thereby modulating the overall metabolic state of the cell .
Transport and Distribution
Within cells and tissues, Iron(II) phthalocyanine is transported and distributed through interactions with various transporters and binding proteins. It can bind to albumin and other plasma proteins, facilitating its distribution throughout the body. Additionally, specific transporters on cell membranes can mediate the uptake of Iron(II) phthalocyanine into cells, where it can accumulate in specific compartments. The localization and accumulation of this compound are influenced by its chemical properties and the presence of binding partners .
Subcellular Localization
Iron(II) phthalocyanine exhibits specific subcellular localization patterns, which can affect its activity and function. It is often found in the mitochondria, where it can influence mitochondrial respiration and energy production. Additionally, Iron(II) phthalocyanine can localize to the nucleus, where it may interact with DNA and nuclear proteins, affecting gene expression and chromatin structure. The subcellular localization of Iron(II) phthalocyanine is determined by targeting signals and post-translational modifications that direct it to specific compartments .
Biologische Aktivität
Phthalocyanine Iron(II) (FePc) is a versatile compound that has garnered significant attention in various fields, including catalysis, electrochemistry, and biomedical applications. This article delves into its biological activity, focusing on its electrocatalytic properties, interactions with biological systems, and potential therapeutic uses.
Overview of Phthalocyanine Iron(II)
Phthalocyanines are macrocyclic compounds similar to porphyrins, consisting of a central metal ion coordinated to a large, planar organic ligand. In the case of FePc, iron serves as the central metal. The unique electronic properties of FePc make it suitable for diverse applications, particularly in catalysis and as a sensor.
Electrocatalytic Activity
FePc has been extensively studied for its electrocatalytic properties, particularly in the context of the oxygen reduction reaction (ORR). Research indicates that the catalytic activity of FePc can be enhanced through various modifications:
- Support Materials : The performance of FePc is significantly influenced by the support materials used. For instance, studies have shown that FePc adsorbed on pyridine-functionalized single-wall carbon nanotubes (py-SWCNT) exhibits higher ORR activity compared to those on regular SWCNTs. This enhancement is attributed to the axial ligand's influence on the electronic properties of FePc and its interaction with the support material .
- Substituent Effects : The presence of electronegative substituents on the phthalocyanine ring can also improve catalytic performance. Perchlorinated derivatives of FePc demonstrate superior ORR activity due to their modified redox potentials .
Table 1: Comparison of ORR Activities
Catalyst Type | Support Material | ORR Activity (mA/cm²) | Tafel Slope (mV/decade) |
---|---|---|---|
FePc | SWCNT | 0.45 | 70 |
16(Cl)FePc | SWCNT | 0.55 | 65 |
FePc | py-SWCNT | 0.60 | 60 |
16(Cl)FePc | py-SWCNT | 0.75 | 55 |
Cytotoxicity and Biocompatibility
Recent studies have explored the cytotoxic effects and biocompatibility of FePc in various biological contexts:
- Nanoparticle Formulations : Iron(II) phthalocyanine has been incorporated into mesoporous titania nanoparticles aimed at targeting breast cancer cells. These nanoparticles demonstrated low toxicity and good biocompatibility in vivo while exhibiting effective photothermal therapy under near-infrared (NIR) laser irradiation .
- Cell Viability Assays : The cytotoxicity of FePc was assessed using CCK-8 assays, which indicated that formulations containing FePc had minimal adverse effects on normal cells while effectively targeting cancerous cells .
Photothermal Therapy
FePc's ability to absorb NIR light allows it to generate heat upon irradiation, making it a candidate for photothermal therapy:
- Mechanism : Upon exposure to NIR light, FePc generates localized heat that can induce apoptosis in cancer cells without damaging surrounding healthy tissues. This property is particularly advantageous in targeted cancer therapies .
Case Studies
- Electrochemical Sensors : A study demonstrated the use of a glass carbon electrode modified with Fe(II) tetra-tert-butyl phthalocyanine for nitrite detection in food products. The sensor exhibited high selectivity and sensitivity towards nitrite ions, showcasing FePc's potential in food safety applications .
- Graphene-Based Applications : Research involving the adsorption of FePc on defective graphene revealed enhanced electronic properties and stability, suggesting potential applications in sensor technology and catalysis .
Wissenschaftliche Forschungsanwendungen
Catalytic Applications
Oxygen Reduction Reaction (ORR)
FePc has emerged as a potential catalyst for the oxygen reduction reaction, which is crucial for energy conversion technologies such as fuel cells and metal-air batteries. Research indicates that the performance of FePc in ORR can be enhanced through coordination with oxidized carbon substrates, improving its catalytic activity. The thermodynamic properties and topological indices associated with FePc provide insights into optimizing its performance in these applications .
Synthetic Catalysis
FePc has been utilized in synthetic organic chemistry as a catalyst for various reactions. For instance, it has been employed in the olefination of aldehydes using diazoacetonitrile, showcasing its efficacy in facilitating complex organic transformations . This application highlights FePc's role in advancing green chemistry by enabling reactions under milder conditions.
Imaging and Diagnostic Applications
Photoacoustic Imaging
Due to its strong near-infrared (NIR) absorption properties, FePc is being explored as a contrast agent for photoacoustic imaging. Studies have demonstrated that nanoparticles loaded with FePc can effectively target cancer cells, providing dual-modal imaging capabilities that enhance tumor visualization . The biocompatibility and safety profile of FePc make it an attractive candidate for clinical applications in cancer diagnostics.
Photothermal Therapy
FePc's ability to generate heat upon NIR irradiation positions it as a promising agent for photothermal therapy (PTT). Research has shown that FePc-loaded nanoparticles can significantly inhibit tumor growth in vivo, demonstrating its potential as a therapeutic agent in cancer treatment . The combination of imaging and therapeutic functions in a single agent underscores the versatility of FePc in modern medical applications.
Material Science Applications
Graphene-Based Composites
FePc has been investigated for its adsorption properties on graphene and defective graphene surfaces. These studies utilize density functional theory to understand the interactions between FePc and graphene materials, paving the way for innovative applications in electronic devices and sensors . The incorporation of FePc into graphene composites may enhance their electrical conductivity and catalytic properties.
Thermodynamic Properties and Structural Analysis
Recent research employing topological descriptors has provided insights into the structural characteristics of FePc. By analyzing various topological indices, scientists can correlate these properties with thermodynamic behavior, aiding in the design of more efficient materials for catalysis and other applications .
Table 1: Summary of Applications
Analyse Chemischer Reaktionen
Olefination of Aldehydes
One of the notable reactions catalyzed by Phthalocyanine Iron(II) is the olefination of aldehydes using diazoacetonitrile. This reaction has been shown to yield alkenyl nitriles efficiently, with yields ranging from 75% to 97%. The mechanism involves the transformation of phosphazine to ylide, which subsequently reacts with aldehydes to form olefins via the Wittig reaction.
Table 1: Olefination Reaction Conditions and Yields
Entry | Catalyst | Solvent | PPh₃ (equiv) | Temperature (°C) | Yield (%) |
---|---|---|---|---|---|
1 | PcFe(II) | Toluene | 1.1 | 80 | 80 |
2 | PcFe(III)Cl | Toluene | 1.1 | 80 | 77 |
3 | PcCo(II) | Toluene | 1.1 | 80 | Trace |
4 | TPPFe(III)Cl | Toluene | 1.1 | 80 | 15 |
5 | TPPCo(II) | Toluene | 1.1 | 80 | 46 |
The results indicate that Phthalocyanine Iron(II) exhibits superior catalytic activity compared to other metal complexes under similar conditions .
Electrocatalytic Reactions
Phthalocyanine Iron(II) has also been investigated for its electrocatalytic properties, particularly in the reduction of oxygen in acidic media. When dispersed on carbon substrates, FePc demonstrates significant catalytic activity, outperforming platinum catalysts at low overpotentials. This reaction's efficiency is attributed to the unique electronic structure of FePc and its ability to facilitate electron transfer processes.
Table 2: Electrocatalytic Performance of FePc
Catalyst | Medium | Activity |
---|---|---|
FePc/C | Acidic (0.5 M H₂SO₄) | High activity without methanol interference |
Pt/C | Acidic (0.5 M H₂SO₄) | Lower activity at similar conditions |
The stability of FePc during these reactions suggests its potential for practical applications in fuel cells and batteries .
Oxidative C−C Cross-Coupling Reactions
Another significant application of Phthalocyanine Iron(II) is in oxidative C−C cross-coupling reactions between tertiary anilines and phenols. Using air as the sole oxidant, this reaction can yield high selectivity and efficiency under mild conditions.
Table 3: Oxidative Cross-Coupling Reaction Yields
Entry | Catalyst | Yield (%) |
---|---|---|
1 | FePc | 66 |
2 | FePcF16 | Higher than FePc |
The performance of the hexadecafluorinated derivative (FePcF16) indicates that electronic modifications can enhance catalytic activity significantly .
Mechanistic Insights
The mechanisms underlying these reactions often involve complex interactions between the iron center and the ligands within the phthalocyanine structure. For instance, in the olefination process, the formation of a phosphorus ylide from phosphazine is crucial for subsequent reactions with aldehydes, demonstrating a clear pathway for product formation.
Transition Metal Catalysis
The transition between ferrous (Fe²⁺) and ferric (Fe³⁺) states in Phthalocyanine Iron(II) can be influenced by external factors such as gas exposure (e.g., NO₂). This transition affects the magnetic properties and reactivity of the complex, highlighting the importance of oxidation states in catalytic performance .
Q & A
Basic Research Questions
Q. What are the optimal synthetic routes for Iron(II) Phthalocyanine, and how are they experimentally validated?
- Methodology : The synthesis typically involves cyclotetramerization of phthalonitrile precursors in the presence of iron salts under inert conditions. For substituted derivatives, precursors like trimethoxyphenoxy-phthalonitrile are used to achieve regioselective functionalization . Characterization employs UV-Vis spectroscopy (Q-band absorption at ~670 nm), FT-IR (C-N stretching at 1280–1320 cm⁻¹), and elemental analysis to confirm purity and ligand coordination. X-ray diffraction (XRD) is critical for structural validation but requires high-quality single crystals, which can be challenging due to aggregation tendencies .
Q. How do axial ligands influence the electronic properties of Iron(II) Phthalocyanine?
- Methodology : Introduce axial ligands (e.g., pyridine, cyanide) during synthesis and compare electronic spectra via UV-Vis-NIR. Density of States (DOS) calculations using DFT (e.g., Vienna Ab Initio Simulation Package, VASP) reveal ligand-induced shifts in frontier orbitals. Cyclic voltammetry (CV) assesses redox behavior, showing ligand-dependent changes in Fe²⁺/Fe³⁺ potentials .
Q. What spectroscopic techniques are most reliable for distinguishing Iron(II) from Iron(III) Phthalocyanine?
- Methodology : Use Mössbauer spectroscopy to identify oxidation states via quadrupole splitting (ΔEQ ≈ 0.8–1.2 mm/s for Fe²⁺ vs. 1.5–2.5 mm/s for Fe³⁺). X-ray photoelectron spectroscopy (XPS) provides binding energy differences (Fe 2p₃/₂: ~708 eV for Fe²⁺ vs. ~711 eV for Fe³⁺). Magnetic susceptibility measurements (SQUID) differentiate paramagnetic Fe²⁺ (high-spin, S=1) from Fe³⁺ (low-spin, S=½) .
Advanced Research Questions
Q. How do exchange-correlation functionals in DFT impact the accuracy of electronic structure predictions for Iron(II) Phthalocyanine?
- Methodology : Compare Generalized Gradient Approximation (GGA-PBE) vs. hybrid functionals (e.g., HSE06) using plane-wave codes (e.g., VASP ). GGA underestimates band gaps but captures delocalized π-electrons, while hybrid functionals improve ligand-field splitting energies at higher computational cost. Validate against experimental UV-Vis and XAS spectra .
Q. What causes discrepancies in reported magnetic moments for Iron(II) Phthalocyanine across studies?
- Methodology : Analyze sample purity (e.g., trace Fe³⁺ contamination via XPS) and crystallinity (XRD). Use CASSCF (Complete Active Space Self-Consistent Field) calculations to account for multi-reference effects in Fe²⁺ d⁶ configuration. Experimental SQUID data must be corrected for diamagnetic contributions from the phthalocyanine ring .
Q. How can the Projector Augmented Wave (PAW) method improve the modeling of Iron(II) Phthalocyanine’s spin states?
- Methodology : Implement PAW pseudopotentials in VASP to reduce core-electron approximation errors. Compare spin-polarized calculations (LSDA+U) with experimental Mössbauer data. PAW provides accurate electron density near the Fe nucleus, critical for predicting spin crossover behavior under external stimuli (e.g., pressure, temperature) .
Q. What strategies resolve contradictions in catalytic activity data for Iron(II) Phthalocyanine in oxygen reduction reactions (ORR)?
- Methodology : Standardize testing conditions (pH, electrolyte, rotating disk electrode settings). Use in-situ Raman spectroscopy to monitor Fe-N₄ active sites during ORR. DFT-based microkinetic modeling identifies rate-limiting steps (e.g., OOH* formation), while Pourbaix diagrams reconcile pH-dependent activity variations .
Q. Methodological Guidelines
- Experimental Design : For reproducibility, document solvent purity (e.g., anhydrous DMF), reaction time/temperature, and glovebox O₂/H₂O levels (<1 ppm) .
- Data Contradictions : Cross-validate computational results with multiple functionals (PBE, SCAN, HSE) and experimental datasets (e.g., EXAFS for bond lengths) .
- Literature Review : Prioritize peer-reviewed studies using databases like SciFinder, avoiding non-academic sources (e.g., BenchChem) .
Vergleich Mit ähnlichen Verbindungen
Iron(II) Phthalocyanine vs. Cobalt Phthalocyanine (CoPc)
- Ag/AgCl in 0.1 M KOH) compared to CoPc (-0.21 V), but higher selectivity for the 4-electron pathway (95% for FePc vs. 75% for CoPc), minimizing peroxide formation .
- Electronic Structure : The Fe(II) center in FePc adopts a high-spin configuration (isomer shift: 0.46–0.49 mm/s), enabling stronger axial ligand interactions (e.g., O₂ adsorption) compared to the low-spin Co(II) in CoPc .
- Stability : CoPc degrades faster under oxidative conditions due to Co leaching, while FePc retains 90% activity after 5,000 cycles in alkaline media .
Table 1 : Key ORR Performance Metrics in Alkaline Media
Property | FePc/CNT | CoPc/CNT | MnPc/Graphene |
---|---|---|---|
Onset Potential (V) | -0.15 | -0.21 | -0.18 |
Electron Transfer # | 3.9 | 3.2 | 3.5 |
H₂O₂ Yield (%) | 5 | 25 | 15 |
Iron(II) Phthalocyanine vs. Iron Porphyrazines
Iron porphyrazines (FePz), structurally analogous to FePc but with a smaller macrocyclic ring, show distinct differences:
- Redox Flexibility : FePc undergoes reversible Fe(II)/Fe(III) transitions at +0.32 V vs. RHE, while FePz exhibits irreversible oxidation due to ligand instability .
- Catalytic Efficiency: FePz derivatives show 30% lower turnover frequency (TOF) in cyclohexane oxidation compared to FePc, attributed to reduced π-conjugation and weaker substrate binding .
Iron(II) Phthalocyanine vs. Metalloporphyrins
- Spin State : FePc’s high-spin Fe(II) enhances O₂ adsorption and activation, whereas low-spin Fe in porphyrins (e.g., heme) limits catalytic versatility .
- Axial Coordination: FePc’s axial Fe–O coordination (e.g., with graphene oxide) improves ORR activity by 40% compared to non-coordinated Fe-porphyrins .
Support-Dependent Performance
FePc’s catalytic behavior varies significantly with the supporting material:
- Carbon Nanotubes (CNT): FePc/CNT achieves a mass activity of 1.2 A mg⁻¹ at 0.8 V, outperforming FePc/carbon spheres (0.8 A mg⁻¹) due to enhanced electron transfer .
- Defective Graphene : FePc adsorbed on graphene with Stone-Wales defects shows a 50% higher adsorption energy (-3.2 eV) than on pristine graphene, stabilizing intermediates in ORR .
Table 2 : Support-Dependent Properties of FePc
Support Type | BET Surface Area (m²/g) | Fe–N₄ Bond Length (Å) | ORR Activity (mA/cm²) |
---|---|---|---|
Carbon Nanotubes | 260 | 1.92 | 4.5 |
Graphene Oxide | 320 | 1.89 | 6.1 |
Carbon Spheres | 180 | 1.95 | 3.2 |
Hybrid Systems and Modified Derivatives
- RHE, surpassing unmodified FePc (+0.75 V) .
- π-π Stacked Composites : FePc/graphene oxide composites exhibit a specific capacitance of 450 F/g, 3× higher than standalone FePc, due to enhanced charge delocalization .
Eigenschaften
CAS-Nummer |
132-16-1 |
---|---|
Molekularformel |
C32H16FeN8 |
Molekulargewicht |
568.4 g/mol |
IUPAC-Name |
2,11,20,29,37,39-hexaza-38,40-diazanidanonacyclo[28.6.1.13,10.112,19.121,28.04,9.013,18.022,27.031,36]tetraconta-1,3,5,7,9,11,13,15,17,19(39),20,22,24,26,28,30(37),31,33,35-nonadecaene;iron(2+) |
InChI |
InChI=1S/C32H16N8.Fe/c1-2-10-18-17(9-1)25-33-26(18)38-28-21-13-5-6-14-22(21)30(35-28)40-32-24-16-8-7-15-23(24)31(36-32)39-29-20-12-4-3-11-19(20)27(34-29)37-25;/h1-16H;/q-2;+2 |
InChI-Schlüssel |
MIINHRNQLVVCEW-UHFFFAOYSA-N |
SMILES |
C1=CC=C2C(=C1)C3=NC4=NC(=NC5=C6C=CC=CC6=C([N-]5)N=C7C8=CC=CC=C8C(=N7)N=C2[N-]3)C9=CC=CC=C94.[Fe+2] |
Kanonische SMILES |
C1=CC=C2C(=C1)C3=NC4=NC(=NC5=C6C=CC=CC6=C([N-]5)N=C7C8=CC=CC=C8C(=N7)N=C2[N-]3)C9=CC=CC=C94.[Fe+2] |
Key on ui other cas no. |
132-16-1 |
Physikalische Beschreibung |
Dark purple crystals; Insoluble in water; [Alfa Aesar MSDS] |
Piktogramme |
Irritant; Environmental Hazard |
Verwandte CAS-Nummern |
36344-64-6 |
Synonyme |
ferrous phthalocyanine iron(II) phthalocyanine |
Herkunft des Produkts |
United States |
Retrosynthesis Analysis
AI-Powered Synthesis Planning: Our tool employs the Template_relevance Pistachio, Template_relevance Bkms_metabolic, Template_relevance Pistachio_ringbreaker, Template_relevance Reaxys, Template_relevance Reaxys_biocatalysis model, leveraging a vast database of chemical reactions to predict feasible synthetic routes.
One-Step Synthesis Focus: Specifically designed for one-step synthesis, it provides concise and direct routes for your target compounds, streamlining the synthesis process.
Accurate Predictions: Utilizing the extensive PISTACHIO, BKMS_METABOLIC, PISTACHIO_RINGBREAKER, REAXYS, REAXYS_BIOCATALYSIS database, our tool offers high-accuracy predictions, reflecting the latest in chemical research and data.
Strategy Settings
Precursor scoring | Relevance Heuristic |
---|---|
Min. plausibility | 0.01 |
Model | Template_relevance |
Template Set | Pistachio/Bkms_metabolic/Pistachio_ringbreaker/Reaxys/Reaxys_biocatalysis |
Top-N result to add to graph | 6 |
Feasible Synthetic Routes
Haftungsausschluss und Informationen zu In-Vitro-Forschungsprodukten
Bitte beachten Sie, dass alle Artikel und Produktinformationen, die auf BenchChem präsentiert werden, ausschließlich zu Informationszwecken bestimmt sind. Die auf BenchChem zum Kauf angebotenen Produkte sind speziell für In-vitro-Studien konzipiert, die außerhalb lebender Organismen durchgeführt werden. In-vitro-Studien, abgeleitet von dem lateinischen Begriff "in Glas", beinhalten Experimente, die in kontrollierten Laborumgebungen unter Verwendung von Zellen oder Geweben durchgeführt werden. Es ist wichtig zu beachten, dass diese Produkte nicht als Arzneimittel oder Medikamente eingestuft sind und keine Zulassung der FDA für die Vorbeugung, Behandlung oder Heilung von medizinischen Zuständen, Beschwerden oder Krankheiten erhalten haben. Wir müssen betonen, dass jede Form der körperlichen Einführung dieser Produkte in Menschen oder Tiere gesetzlich strikt untersagt ist. Es ist unerlässlich, sich an diese Richtlinien zu halten, um die Einhaltung rechtlicher und ethischer Standards in Forschung und Experiment zu gewährleisten.