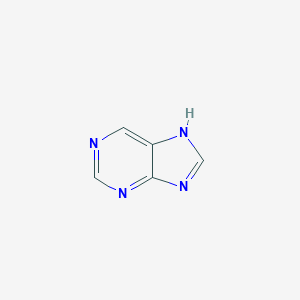
Purine
Übersicht
Beschreibung
Purine is a heterocyclic aromatic organic compound composed of a fused pyrimidine ring (six-membered) and an imidazole ring (five-membered) . This bicyclic structure forms the backbone of critical biomolecules, including adenine and guanine—key constituents of DNA and RNA. Beyond nucleic acids, purines are integral to cellular energy metabolism (e.g., ATP, GTP), signal transduction (e.g., cAMP), and enzyme cofactors (e.g., NAD, FAD) .
This compound metabolism is tightly regulated, with imbalances linked to pathologies such as gout, Lesch-Nyhan syndrome, and cancer . Over 10 this compound antimetabolites, including 6-mercaptothis compound and 6-thioguanine, are FDA-approved for cancer therapy. These compounds mimic endogenous purines, disrupting nucleotide synthesis and DNA replication in rapidly dividing cells .
Vorbereitungsmethoden
Synthetische Wege und Reaktionsbedingungen: Purin kann durch verschiedene Verfahren synthetisiert werden, darunter der De-novo-Syntheseweg und der Salvage-Weg. Die De-novo-Synthese beinhaltet die Bildung von Purinnukleotiden aus einfachen Molekülen wie Phosphoribosylpyrophosphat, Glycin, Glutamin und Kohlendioxid . Dieser Weg findet in der Leber statt und beinhaltet eine Reihe von enzymatischen Reaktionen, die den Purinring an einer Ribose-Einheit aufbauen .
Industrielle Produktionsverfahren: Die industrielle Produktion von Purin beinhaltet häufig die Verwendung von Ionenaustauschchromatographie zur Trennung und Reinigung von Purinbasen . Dieses Verfahren eignet sich besonders zur Isolierung von Purin aus komplexen Gemischen, da es aufgrund seiner Fähigkeit, Verbindungen basierend auf ihren Ladungseigenschaften zu trennen, angewendet werden kann .
Analyse Chemischer Reaktionen
Chemical Modifications
Purines can undergo various chemical modifications that enhance their biological activity or alter their properties:
-
Alkylation : The introduction of alkyl groups can modify the pharmacological properties of purines.
-
Halogenation : This involves the substitution of hydrogen atoms with halogen atoms, which can affect the reactivity and stability of purines.
-
Amination : The addition of amino groups can lead to the formation of different purine derivatives with potential therapeutic applications.
Table 2: Common Chemical Modifications of Purines
Modification Type | Example Reaction | Resulting Product |
---|---|---|
Alkylation | Alkyl halide + this compound | Alkylated this compound derivative |
Halogenation | Halogen + this compound | Halogenated this compound derivative |
Amination | Amine + this compound | Aminated this compound derivative |
Biological Relevance
Purines are not only structural components of nucleic acids but also serve as signaling molecules and energy carriers (e.g., ATP). Their metabolism is tightly regulated due to their involvement in critical cellular processes such as DNA repair and cell division.
This compound Metabolism and Disease
Alterations in this compound metabolism have been linked to various diseases, including cancer. For example, studies indicate that this compound metabolites can influence DNA repair mechanisms, contributing to therapy resistance in certain cancer types .
Recent Advances in this compound Research
Recent studies have focused on innovative synthetic methods for producing purines and their derivatives. Techniques involving new catalysts and reaction conditions have been explored to improve yields and selectivity in synthesizing bioactive purines .
Innovative Synthetic Routes
Recent advancements include:
-
The use of diaminomaleonitrile as a precursor for synthesizing various purines.
-
Employing urea derivatives and imidazole as starting materials for more efficient synthesis pathways.
These methods not only enhance the efficiency of this compound synthesis but also open avenues for creating novel compounds with potential therapeutic applications.
Wissenschaftliche Forschungsanwendungen
Biological Functions of Purine
Purines are vital for numerous cellular functions:
- Nucleic Acid Structure : Adenine and guanine are integral to the structure of DNA and RNA.
- Energy Metabolism : ATP (adenosine triphosphate) and GTP (guanosine triphosphate) are key energy carriers in cellular processes.
- Signal Transduction : cAMP (cyclic adenosine monophosphate) acts as a secondary messenger in various signaling pathways.
Medicinal Applications
This compound derivatives have been extensively studied for their therapeutic potential. Below are some notable applications:
Chemotherapeutics
Purines and their analogs have been developed as chemotherapeutic agents due to their ability to interfere with nucleic acid synthesis:
- Fludarabine : An analog used in treating chronic lymphocytic leukemia (CLL) by inhibiting DNA synthesis.
- Thiopurines : Such as azathioprine, used in autoimmune diseases and organ transplantation by suppressing immune responses .
Antiviral Agents
Certain this compound derivatives exhibit antiviral properties:
- Acyclovir : A guanine analog effective against herpes viruses by inhibiting viral DNA polymerase.
- Ganciclovir : Another guanine derivative used to treat cytomegalovirus infections .
Anticancer Agents
Purines play a role in cancer treatment through various mechanisms:
- Cyclin-dependent kinase (CDK) inhibitors : These this compound-based compounds promote apoptosis in cancer cells .
- Purinergic receptor modulators : Targeting adenosine receptors can influence tumor growth and metastasis .
Research Applications
Purines are utilized in various research fields, including:
Biochemical Probes
Purines serve as probes for studying biochemical pathways:
- Fluorescent adenosine derivatives help elucidate mechanisms of signal transduction and metabolism .
- 8-bromoguanosine is used to investigate nucleic acid biophysics.
Enzyme Inhibition Studies
Purines act as substrates or inhibitors for enzymes involved in this compound metabolism:
- Research on this compound nucleoside phosphorylase (PNP) has implications for drug development targeting metabolic disorders .
Synthesis and Derivatives
Recent advancements in synthetic methods have expanded the accessibility of this compound derivatives:
Uric Acid and Disease Management
Elevated levels of uric acid, a product of this compound metabolism, have been linked to various diseases:
- Gout : Characterized by high serum uric acid levels, leading to inflammation.
- Cardiovascular Diseases : Hyperuricemia is associated with increased risk factors for heart disease .
Dietary Purines
Research has shown that dietary intake of purines impacts health:
Wirkmechanismus
Purines exert their effects through various mechanisms:
Molecular Targets: Purines interact with specific receptors, such as adenosine receptors, which are involved in cell signaling pathways.
Pathways Involved: Purines play a role in energy metabolism, signal transduction, and the regulation of cellular processes.
Vergleich Mit ähnlichen Verbindungen
Purine derivatives and structurally analogous compounds exhibit distinct chemical, functional, and pharmacological properties. Below is a systematic comparison:
Pyrimidines
Property | This compound | Pyrimidine |
---|---|---|
Structure | Bicyclic (pyrimidine + imidazole) | Monocyclic (six-membered) |
Nucleobases | Adenine, Guanine | Cytosine, Thymine, Uracil |
Metabolic Role | Energy carriers, signaling molecules | Primarily nucleic acid synthesis |
Catabolic End Product | Uric acid | Beta-amino acids, ammonia |
Pyrimidines lack the imidazole ring, reducing their structural complexity and metabolic versatility compared to purines. While both classes are essential for nucleic acid synthesis, purines dominate energy transfer and signaling pathways .
Uric Acid
Uric acid, a this compound catabolite, shares a heterocyclic core with this compound but differs in oxidation state and functional groups:
- Structure : A trioxythis compound with keto groups at positions 2, 6, and 6.
- Function : Antioxidant in humans but pathogenic at high concentrations (e.g., gout, kidney stones) .
- However, its structural similarity to caffeine (a methylxanthine) allows cross-reactivity in assays targeting this compound-binding proteins .
This compound Antimetabolites
FDA-approved this compound antimetabolites mimic natural purines to inhibit DNA synthesis:
Compound | Modification | Mechanism | Clinical Use |
---|---|---|---|
6-Mercaptothis compound | Sulfhydryl substitution at C6 | Inhibits de novo this compound synthesis | Leukemia, lymphoma |
6-Thioguanine | Sulfur-for-oxygen at C6 | Incorporates into DNA, causing strand breaks | Acute myeloid leukemia |
Fludarabine | Fluorinated arabinose sugar | Inhibits DNA polymerase and ribonucleotide reductase | Chronic lymphocytic leukemia |
These analogs exploit structural similarities to disrupt this compound metabolism but diverge in pharmacokinetics and toxicity profiles .
Xanthine and Hypoxanthine
- Xanthine: An intermediate in this compound degradation with two keto groups (positions 2 and 6).
- Hypoxanthine : Formed during ATP catabolism, it salvages this compound nucleotides via hypoxanthine-guanine phosphoribosyltransferase (HGPRT). Structural flexibility allows it to pair with adenine, thymine, and uracil under mutagenic conditions .
Cancer Mutagenesis and this compound Stretches
Genomic analyses reveal that cancer-associated mutations preferentially avoid this compound-rich regions (poly-purine stretches). These sequences stabilize DNA secondary structures (e.g., G-quadruplexes) and regulate chromatin organization, suggesting this compound stretches are under negative selection in oncogenesis .
Enzymatic this compound Reduction in Food
In beer production, an enzymatic cocktail (adenine deaminase, guanine deaminase, xanthine oxidase) reduces this compound content by 40–60%, mitigating hyperuricemia risk. Uric acid, a byproduct, is further degraded to allantoin .
Antimalarial Drug Design
Structural studies of Plasmodium falciparum this compound phosphoribosyltransferase (HGXPRTase) bound to transition-state inhibitors (e.g., immucillin-H) reveal conserved catalytic residues with human HGPRT. However, distinct hydrogen-bonding networks enable selective inhibitor design .
Biologische Aktivität
Purines are a class of organic compounds that play crucial roles in various biological processes, including energy metabolism, cellular signaling, and the synthesis of nucleic acids. This article explores the biological activity of purines, focusing on their mechanisms, physiological roles, and implications in health and disease.
Overview of Purine Structure and Function
Purines consist of a fused double-ring structure containing nitrogen atoms. The most notable purines include adenine and guanine, which are integral components of nucleotides (the building blocks of DNA and RNA). Additionally, purines such as adenosine triphosphate (ATP) serve as primary energy carriers in cells.
Key Functions of Purines:
- Energy Metabolism : ATP is essential for energy transfer within cells.
- Cell Signaling : Purines act as signaling molecules through purinergic receptors, influencing various physiological processes.
- Nucleotide Synthesis : Purines are precursors for DNA and RNA synthesis.
1. Purinergic Signaling
Purinergic signaling refers to the role of purines in cell communication. ATP and adenosine interact with specific receptors (e.g., P2X and P1 receptors), triggering intracellular signaling pathways that regulate numerous functions, including:
- Immune Response : Activation of immune cells through ATP signaling enhances inflammatory responses .
- Neuronal Function : Adenosine modulates neurotransmitter release and neuronal excitability, impacting conditions like epilepsy .
2. Antioxidant Properties
Purines exhibit antioxidant properties that help protect cells from oxidative stress. Uric acid, the end product of this compound metabolism in humans, acts as a potent antioxidant, scavenging free radicals and reducing oxidative damage .
3. Role in Cancer
Purines are implicated in cancer biology. Tumor cells often exhibit altered this compound metabolism to support rapid proliferation. For instance, the de novo synthesis pathway is upregulated in these cells to meet increased this compound demands . Additionally, this compound analogs are utilized as chemotherapeutic agents due to their ability to inhibit DNA synthesis in rapidly dividing cancer cells .
Case Study 1: Neuronal Differentiation
A study on rat PC6-3 cells demonstrated that this compound metabolism is critical during neuronal differentiation. The research highlighted an increase in this compound levels post-differentiation, suggesting that adequate this compound availability is vital for proper neuronal function .
Case Study 2: this compound Metabolism Disorders
Lesch-Nyhan syndrome is an inherited disorder caused by a deficiency in hypoxanthine-guanine phosphoribosyltransferase (HGprt), leading to abnormal this compound metabolism. Patients exhibit neurological symptoms and increased uric acid levels due to disrupted recycling pathways .
Research Findings
Recent studies have elucidated various aspects of this compound biology:
Q & A
Q. What are the validated methodologies for quantifying purine levels in biological samples, and how are they optimized for reproducibility?
Basic Research Question
Quantification of purines (e.g., adenine, guanine, hypoxanthine) typically employs high-performance liquid chromatography (HPLC) with UV detection or liquid chromatography–mass spectrometry (LC-MS) for enhanced sensitivity . Key optimization steps include:
- Sample Preparation : Deproteinization using perchloric acid or ultrafiltration to prevent interference.
- Chromatographic Conditions : Use of ion-pairing agents (e.g., tetrabutylammonium phosphate) to improve peak resolution.
- Validation : Calibration curves with internal standards (e.g., ¹³C-labeled purines) to account for matrix effects .
Reproducibility is ensured through triplicate runs and adherence to protocols from journals like the Beilstein Journal of Organic Chemistry, which mandate detailed experimental descriptions for replication .
Q. How do researchers address discrepancies between in vivo and in vitro models of this compound metabolism?
Advanced Research Question
Conflicting data often arise from differences in metabolic compartmentalization (e.g., cytosolic vs. mitochondrial pathways). Methodological strategies include:
- Model Selection : Use of hepatocyte cultures for in vitro studies to mirror hepatic this compound synthesis, complemented by knockout mouse models (e.g., Hprt-deficient mice) for in vivo validation .
- Data Normalization : Adjust for extracellular this compound uptake in vitro using isotope tracing (e.g., ¹⁵N-labeled adenine) .
- Cross-Validation : Meta-analysis of existing datasets via scoping reviews to identify confounding variables (e.g., pH, oxygen tension) .
Q. What analytical frameworks are recommended for resolving contradictions in this compound catabolism studies?
Advanced Research Question
Systematic resolution involves:
- Source Analysis : Compare experimental conditions (e.g., enzyme sources, buffer compositions) across studies. For example, xanthine oxidase activity varies significantly with pH (optimal at 7.4) and substrate purity .
- Controlled Replication : Reproduce conflicting experiments under standardized protocols, as emphasized by guidelines for scientific integrity .
- Statistical Harmonization : Apply multivariate regression to isolate variables (e.g., dietary this compound intake in animal studies) contributing to divergent outcomes .
Q. How can multi-omics approaches (genomic, transcriptomic, metabolomic) be integrated to study this compound-related disorders?
Advanced Research Question
Integration strategies include:
- Hypothesis-Driven Design : Use PICOT (Population, Intervention, Comparison, Outcome, Time) frameworks to align omics layers. For example, linking APRT gene mutations (genomic) with urinary 2,8-dihydroxyadenine excretion (metabolomic) in adenine phosphoribosyltransferase deficiency .
- Data Fusion Tools : Platforms like XCMS Online for LC-MS metabolomics coupled with RNA-seq data to identify regulatory networks .
- Validation Pipelines : Confirm omics findings with targeted assays (e.g., qPCR for gene expression, enzymatic activity assays) .
Q. What protocols ensure this compound stability during long-term storage and experimental assays?
Basic Research Question
Stability protocols involve:
- Storage Conditions : -80°C storage in inert buffers (e.g., Tris-EDTA) to prevent oxidation. Lyophilization is avoided for labile purines like uric acid .
- Inhibitor Use : Add adenosine deaminase inhibitors (e.g., erythro-9-(2-hydroxy-3-nonyl)adenine) to prevent enzymatic degradation during tissue homogenization .
- Analytical Validation : Periodic stability testing via LC-MS to detect degradation products (e.g., allantoin from uric acid) .
Q. How should researchers design experiments to investigate this compound salvage pathway redundancy across species?
Advanced Research Question
Comparative experimental design requires:
- Phylogenetic Analysis : Select model organisms (e.g., Drosophila vs. mammals) with divergent salvage pathways.
- Knockout/Rescue Studies : Disrupt key enzymes (e.g., Hprt in mice) and assess compensatory mechanisms via metabolomic profiling .
- Computational Modeling : Use flux balance analysis (FBA) to predict pathway utilization under varying this compound availability .
Q. What statistical methods are most robust for analyzing this compound flux data in dynamic metabolic studies?
Advanced Research Question
Robust analysis employs:
- Kinetic Modeling : Compartmental models (e.g., Michaelis-Menten for enzyme kinetics) to quantify this compound turnover rates .
- Time-Series Analysis : Autoregressive integrated moving average (ARIMA) models for longitudinal metabolomics data .
- Error Propagation : Monte Carlo simulations to account for analytical variability in LC-MS measurements .
Q. How do researchers ethically validate this compound-related biomarkers in clinical studies?
Basic Research Question
Ethical validation follows:
- Informed Consent : Explicit participant agreement for biomarker analysis, per guidelines from clinical journals .
- Blinded Analysis : Separate teams for data collection and interpretation to reduce bias .
- External Audits : Third-party verification of biomarker reproducibility, as recommended by the Journal of Clinical Practice and Research .
Eigenschaften
IUPAC Name |
7H-purine | |
---|---|---|
Source | PubChem | |
URL | https://pubchem.ncbi.nlm.nih.gov | |
Description | Data deposited in or computed by PubChem | |
InChI |
InChI=1S/C5H4N4/c1-4-5(8-2-6-1)9-3-7-4/h1-3H,(H,6,7,8,9) | |
Source | PubChem | |
URL | https://pubchem.ncbi.nlm.nih.gov | |
Description | Data deposited in or computed by PubChem | |
InChI Key |
KDCGOANMDULRCW-UHFFFAOYSA-N | |
Source | PubChem | |
URL | https://pubchem.ncbi.nlm.nih.gov | |
Description | Data deposited in or computed by PubChem | |
Canonical SMILES |
C1=C2C(=NC=N1)N=CN2 | |
Source | PubChem | |
URL | https://pubchem.ncbi.nlm.nih.gov | |
Description | Data deposited in or computed by PubChem | |
Molecular Formula |
C5H4N4 | |
Source | PubChem | |
URL | https://pubchem.ncbi.nlm.nih.gov | |
Description | Data deposited in or computed by PubChem | |
DSSTOX Substance ID |
DTXSID5074470 | |
Record name | Purine | |
Source | EPA DSSTox | |
URL | https://comptox.epa.gov/dashboard/DTXSID5074470 | |
Description | DSSTox provides a high quality public chemistry resource for supporting improved predictive toxicology. | |
Molecular Weight |
120.11 g/mol | |
Source | PubChem | |
URL | https://pubchem.ncbi.nlm.nih.gov | |
Description | Data deposited in or computed by PubChem | |
Physical Description |
Solid | |
Record name | Purine | |
Source | Human Metabolome Database (HMDB) | |
URL | http://www.hmdb.ca/metabolites/HMDB0001366 | |
Description | The Human Metabolome Database (HMDB) is a freely available electronic database containing detailed information about small molecule metabolites found in the human body. | |
Explanation | HMDB is offered to the public as a freely available resource. Use and re-distribution of the data, in whole or in part, for commercial purposes requires explicit permission of the authors and explicit acknowledgment of the source material (HMDB) and the original publication (see the HMDB citing page). We ask that users who download significant portions of the database cite the HMDB paper in any resulting publications. | |
Solubility |
500.0 mg/mL | |
Record name | Purine | |
Source | Human Metabolome Database (HMDB) | |
URL | http://www.hmdb.ca/metabolites/HMDB0001366 | |
Description | The Human Metabolome Database (HMDB) is a freely available electronic database containing detailed information about small molecule metabolites found in the human body. | |
Explanation | HMDB is offered to the public as a freely available resource. Use and re-distribution of the data, in whole or in part, for commercial purposes requires explicit permission of the authors and explicit acknowledgment of the source material (HMDB) and the original publication (see the HMDB citing page). We ask that users who download significant portions of the database cite the HMDB paper in any resulting publications. | |
CAS No. |
120-73-0 | |
Record name | Purine | |
Source | CAS Common Chemistry | |
URL | https://commonchemistry.cas.org/detail?cas_rn=120-73-0 | |
Description | CAS Common Chemistry is an open community resource for accessing chemical information. Nearly 500,000 chemical substances from CAS REGISTRY cover areas of community interest, including common and frequently regulated chemicals, and those relevant to high school and undergraduate chemistry classes. This chemical information, curated by our expert scientists, is provided in alignment with our mission as a division of the American Chemical Society. | |
Explanation | The data from CAS Common Chemistry is provided under a CC-BY-NC 4.0 license, unless otherwise stated. | |
Record name | 1H-Purine | |
Source | ChemIDplus | |
URL | https://pubchem.ncbi.nlm.nih.gov/substance/?source=chemidplus&sourceid=0000120730 | |
Description | ChemIDplus is a free, web search system that provides access to the structure and nomenclature authority files used for the identification of chemical substances cited in National Library of Medicine (NLM) databases, including the TOXNET system. | |
Record name | Purine | |
Source | DTP/NCI | |
URL | https://dtp.cancer.gov/dtpstandard/servlet/dwindex?searchtype=NSC&outputformat=html&searchlist=753 | |
Description | The NCI Development Therapeutics Program (DTP) provides services and resources to the academic and private-sector research communities worldwide to facilitate the discovery and development of new cancer therapeutic agents. | |
Explanation | Unless otherwise indicated, all text within NCI products is free of copyright and may be reused without our permission. Credit the National Cancer Institute as the source. | |
Record name | Purine | |
Source | EPA DSSTox | |
URL | https://comptox.epa.gov/dashboard/DTXSID5074470 | |
Description | DSSTox provides a high quality public chemistry resource for supporting improved predictive toxicology. | |
Record name | Purine | |
Source | European Chemicals Agency (ECHA) | |
URL | https://echa.europa.eu/substance-information/-/substanceinfo/100.004.020 | |
Description | The European Chemicals Agency (ECHA) is an agency of the European Union which is the driving force among regulatory authorities in implementing the EU's groundbreaking chemicals legislation for the benefit of human health and the environment as well as for innovation and competitiveness. | |
Explanation | Use of the information, documents and data from the ECHA website is subject to the terms and conditions of this Legal Notice, and subject to other binding limitations provided for under applicable law, the information, documents and data made available on the ECHA website may be reproduced, distributed and/or used, totally or in part, for non-commercial purposes provided that ECHA is acknowledged as the source: "Source: European Chemicals Agency, http://echa.europa.eu/". Such acknowledgement must be included in each copy of the material. ECHA permits and encourages organisations and individuals to create links to the ECHA website under the following cumulative conditions: Links can only be made to webpages that provide a link to the Legal Notice page. | |
Record name | PURINE | |
Source | FDA Global Substance Registration System (GSRS) | |
URL | https://gsrs.ncats.nih.gov/ginas/app/beta/substances/W60KTZ3IZY | |
Description | The FDA Global Substance Registration System (GSRS) enables the efficient and accurate exchange of information on what substances are in regulated products. Instead of relying on names, which vary across regulatory domains, countries, and regions, the GSRS knowledge base makes it possible for substances to be defined by standardized, scientific descriptions. | |
Explanation | Unless otherwise noted, the contents of the FDA website (www.fda.gov), both text and graphics, are not copyrighted. They are in the public domain and may be republished, reprinted and otherwise used freely by anyone without the need to obtain permission from FDA. Credit to the U.S. Food and Drug Administration as the source is appreciated but not required. | |
Record name | Purine | |
Source | Human Metabolome Database (HMDB) | |
URL | http://www.hmdb.ca/metabolites/HMDB0001366 | |
Description | The Human Metabolome Database (HMDB) is a freely available electronic database containing detailed information about small molecule metabolites found in the human body. | |
Explanation | HMDB is offered to the public as a freely available resource. Use and re-distribution of the data, in whole or in part, for commercial purposes requires explicit permission of the authors and explicit acknowledgment of the source material (HMDB) and the original publication (see the HMDB citing page). We ask that users who download significant portions of the database cite the HMDB paper in any resulting publications. | |
Melting Point |
214 °C | |
Record name | Purine | |
Source | Human Metabolome Database (HMDB) | |
URL | http://www.hmdb.ca/metabolites/HMDB0001366 | |
Description | The Human Metabolome Database (HMDB) is a freely available electronic database containing detailed information about small molecule metabolites found in the human body. | |
Explanation | HMDB is offered to the public as a freely available resource. Use and re-distribution of the data, in whole or in part, for commercial purposes requires explicit permission of the authors and explicit acknowledgment of the source material (HMDB) and the original publication (see the HMDB citing page). We ask that users who download significant portions of the database cite the HMDB paper in any resulting publications. | |
Synthesis routes and methods I
Procedure details
Synthesis routes and methods II
Procedure details
Synthesis routes and methods III
Procedure details
Synthesis routes and methods IV
Procedure details
Retrosynthesis Analysis
AI-Powered Synthesis Planning: Our tool employs the Template_relevance Pistachio, Template_relevance Bkms_metabolic, Template_relevance Pistachio_ringbreaker, Template_relevance Reaxys, Template_relevance Reaxys_biocatalysis model, leveraging a vast database of chemical reactions to predict feasible synthetic routes.
One-Step Synthesis Focus: Specifically designed for one-step synthesis, it provides concise and direct routes for your target compounds, streamlining the synthesis process.
Accurate Predictions: Utilizing the extensive PISTACHIO, BKMS_METABOLIC, PISTACHIO_RINGBREAKER, REAXYS, REAXYS_BIOCATALYSIS database, our tool offers high-accuracy predictions, reflecting the latest in chemical research and data.
Strategy Settings
Precursor scoring | Relevance Heuristic |
---|---|
Min. plausibility | 0.01 |
Model | Template_relevance |
Template Set | Pistachio/Bkms_metabolic/Pistachio_ringbreaker/Reaxys/Reaxys_biocatalysis |
Top-N result to add to graph | 6 |
Feasible Synthetic Routes
Haftungsausschluss und Informationen zu In-Vitro-Forschungsprodukten
Bitte beachten Sie, dass alle Artikel und Produktinformationen, die auf BenchChem präsentiert werden, ausschließlich zu Informationszwecken bestimmt sind. Die auf BenchChem zum Kauf angebotenen Produkte sind speziell für In-vitro-Studien konzipiert, die außerhalb lebender Organismen durchgeführt werden. In-vitro-Studien, abgeleitet von dem lateinischen Begriff "in Glas", beinhalten Experimente, die in kontrollierten Laborumgebungen unter Verwendung von Zellen oder Geweben durchgeführt werden. Es ist wichtig zu beachten, dass diese Produkte nicht als Arzneimittel oder Medikamente eingestuft sind und keine Zulassung der FDA für die Vorbeugung, Behandlung oder Heilung von medizinischen Zuständen, Beschwerden oder Krankheiten erhalten haben. Wir müssen betonen, dass jede Form der körperlichen Einführung dieser Produkte in Menschen oder Tiere gesetzlich strikt untersagt ist. Es ist unerlässlich, sich an diese Richtlinien zu halten, um die Einhaltung rechtlicher und ethischer Standards in Forschung und Experiment zu gewährleisten.