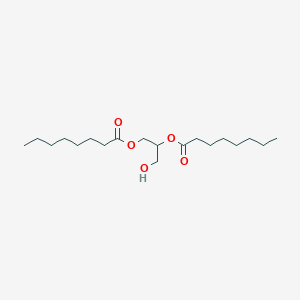
1,2-Dioctanoylglycerol
Übersicht
Beschreibung
1,2-Dioctanoylglycerol (1,2-DiC8) is a synthetic diacylglycerol (DAG) with two octanoyl (C8) fatty acid chains esterified at the sn-1 and sn-2 positions of the glycerol backbone. As a cell-permeable analog of endogenous DAGs, it is widely used to study lipid-mediated signaling pathways, particularly protein kinase C (PKC) activation . Its short acyl chains enhance solubility compared to long-chain DAGs, making it a practical tool for in vitro and cellular studies . Research highlights its role in modulating phospholipase A2 (PLA2) activity , MAPK/ERK signaling in lipolysis , and mitotic progression in plant cells .
Vorbereitungsmethoden
Chemical Acylation Strategies
Acylation of Glycerol Derivatives
The cornerstone of 1,2-dioctanoylglycerol synthesis involves regioselective acylation of glycerol or its derivatives. A widely adopted method employs octanoyl chloride as the acylating agent, reacting with 1,2-diol precursors under anhydrous conditions. For example, (S)-1,2-propanediol is acylated with excess octanoyl chloride in the presence of a base such as pyridine to neutralize HCl byproducts . The reaction typically proceeds at room temperature or under reflux in nonpolar solvents like benzene or dichloromethane.
Key Reaction Parameters:
Reagent | Solvent | Temperature | Yield (%) | Reference |
---|---|---|---|---|
Octanoyl chloride | Benzene | Reflux | 85–90 | |
Octanoyl chloride | CH₂Cl₂ | 25°C | 75–80 |
The stereospecific sn-1,2 configuration is preserved by using chiral starting materials or through kinetic control, favoring acylation at the primary hydroxyl groups.
Protection-Deprotection Approaches
To prevent unwanted acylation at the sn-3 position, protective groups are employed. For instance, 1,2-diols like 1,2-propanediol are first protected as benzyl ethers or acetonides before acylation. After acylation, the protecting group is removed via catalytic hydrogenolysis (for benzyl ethers) or acid hydrolysis (for acetonides) .
Example Workflow:
-
Acetonide Formation: 1,2-Propanediol is treated with 2,2-dimethoxypropane under acidic conditions to form a 1,2-acetonide.
-
Acylation: The acetonide is reacted with octanoyl chloride in dichloromethane.
-
Deprotection: The acetonide is cleaved using p-toluenesulfonic acid in methanol, yielding this compound.
Data Table:
Protective Group | Deprotection Method | Purity Post-Deprotection (%) |
---|---|---|
Benzyl ether | H₂/Pd | 92 |
Acetonide | Acid hydrolysis | 88 |
Enzymatic Synthesis
Lipase-Catalyzed Esterification
Although less common in industrial settings, lipases such as Candida antarctica lipase B (CAL-B) enable regioselective acylation of glycerol in non-aqueous media. This method offers milder conditions and avoids racemization. Glycerol is incubated with vinyl octanoate or octanoic acid in tert-butanol, with the enzyme immobilized on a solid support .
Optimized Conditions:
Parameter | Value |
---|---|
Temperature | 40°C |
Solvent | tert-Butanol |
Enzyme Loading | 10% (w/w) |
Conversion Rate | 70–75% |
Purification and Analytical Validation
Chromatographic Techniques
Crude synthesis mixtures often contain regioisomers (e.g., 1,3-dioctanoylglycerol) and unreacted starting materials. Reversed-phase HPLC with C18 columns and evaporative light scattering detection (ELSD) resolves these impurities. Mobile phases typically comprise acetonitrile/water gradients (70:30 to 100:0 over 20 min) .
HPLC Parameters:
Column | Flow Rate (mL/min) | Detection | Retention Time (min) |
---|---|---|---|
C18 (250 mm) | 1.0 | ELSD | 12.3 (1,2-isomer) |
Structural Confirmation
Nuclear Magnetic Resonance (NMR) is indispensable for verifying the sn-1,2 regiochemistry. Key signals include:
-
¹H NMR (CDCl₃): δ 4.15–4.25 (m, sn-1,2 glycerol protons), δ 2.30–2.35 (t, octanoyl CH₂).
-
¹³C NMR (CDCl₃): δ 173.5 (ester carbonyl), δ 63.2 (sn-3 glycerol carbon).
Comparative Analysis of Synthetic Routes
Method | Yield (%) | Purity (%) | Regioselectivity | Scalability |
---|---|---|---|---|
Chemical Acylation | 85–90 | 95 | High | Industrial |
Enzymatic Synthesis | 70–75 | 90 | Moderate | Lab-scale |
Chemical acylation remains the gold standard for large-scale production, while enzymatic methods are favored for specialty applications requiring minimal side reactions.
Condition | Degradation Products | Half-Life (Days) |
---|---|---|
25°C, dry argon | None | >180 |
25°C, ambient air | 1,3-dioctanoylglycerol | 30 |
4°C, aqueous buffer | Octanoic acid, glycerol | 7 |
Industrial-Scale Production Challenges
Cost vs. Purity Trade-offs
Bulk synthesis prioritizes cost-effective catalysts (e.g., H₂SO₄) over regioselectivity, necessitating rigorous post-synthesis purification. In contrast, small-scale research batches use high-purity reagents and chiral catalysts, achieving >98% regiochemical fidelity .
Environmental Impact
Traditional methods generate HCl waste, requiring neutralization. Emerging green chemistry approaches utilize enzyme cascades or recyclable solvents like cyclopentyl methyl ether (CPME).
Emerging Innovations
Continuous Flow Synthesis
Microreactor technology enables continuous acylation, reducing reaction times from hours to minutes. Preliminary data show:
Reactor Type | Residence Time (min) | Yield (%) |
---|---|---|
Microfluidic | 10 | 82 |
Batch | 120 | 85 |
Solid-Phase Synthesis
Immobilized glycerol analogs on resin beads allow stepwise acylation, though yields remain suboptimal (50–60%) .
Analyse Chemischer Reaktionen
Types of Reactions
1,2-Dioctanoylglycerol undergoes various chemical reactions, including:
Oxidation: It can be oxidized to form corresponding carboxylic acids.
Reduction: Reduction reactions can convert it into alcohols.
Substitution: It can undergo nucleophilic substitution reactions, where the ester groups are replaced by other functional groups.
Common Reagents and Conditions
Oxidation: Common oxidizing agents include potassium permanganate (KMnO4) and chromium trioxide (CrO3).
Reduction: Reducing agents such as lithium aluminum hydride (LiAlH4) or sodium borohydride (NaBH4) are used.
Substitution: Nucleophiles like amines or thiols can be used under basic conditions.
Major Products Formed
Oxidation: Octanoic acid and glycerol derivatives.
Reduction: Octanol and glycerol derivatives.
Substitution: Various substituted glycerol derivatives depending on the nucleophile used.
Wissenschaftliche Forschungsanwendungen
Protein Kinase C Activation
One of the primary applications of 1,2-dioctanoylglycerol is its role as a potent activator of protein kinase C. PKC is a family of enzymes that play vital roles in numerous cellular processes, including cell proliferation, differentiation, and apoptosis. By mimicking the action of naturally occurring diacylglycerols, this compound allows researchers to investigate the downstream effects of PKC activation in various cell types and model systems .
Investigating Cellular Signaling Pathways
Research has shown that this compound can influence intracellular calcium levels and interact with other signaling pathways beyond PKC. This broadens its utility as a research tool for studying various aspects of cell signaling mechanisms.
Mitotic Progression Studies
Studies have demonstrated that the timing and concentration of this compound treatment can significantly affect mitotic progression in cells. For instance, treatment during specific phases of mitosis can either accelerate or retard cell division processes. This characteristic allows researchers to explore regulatory checkpoints in the cell cycle .
Effects on Steroidogenesis
In studies involving porcine Leydig cells, this compound was shown to modulate steroidogenesis by inhibiting forskolin-stimulated testosterone production. This suggests potential applications in endocrinological research .
Cardiac Physiology Research
Research involving adult rat ventricular myocytes has indicated that this compound can inhibit L-type calcium currents independently of PKC activation. This finding highlights its potential role in cardiac physiology studies and drug development targeting cardiac function .
Comparative Analysis with Other Compounds
To better understand the unique properties and applications of this compound, it is useful to compare it with other diacylglycerols.
Compound Name | Activation of PKC | Effect on Calcium Levels | Applications |
---|---|---|---|
This compound | Yes | Yes | Cell signaling studies, mitotic progression |
Phorbol 12-myristate 13-acetate | Yes | Yes | Tumor promotion studies |
1,3-Dioctanoylglycerol | No | No | Control compound for comparative studies |
Case Study 1: Mitotic Progression
In an experiment involving stamen hair cells from Tradescantia virginiana, varying concentrations of this compound were applied at different stages of mitosis. Results indicated that treatment at late prophase led to rapid nuclear envelope breakdown and accelerated entry into anaphase compared to untreated controls .
Case Study 2: Steroidogenesis Modulation
A study examined the effects of this compound on testosterone production in porcine Leydig cells. The compound inhibited forskolin-stimulated steroidogenesis, indicating its potential use in exploring hormonal regulation mechanisms .
Wirkmechanismus
1,2-Dioctanoylglycerol activates protein kinase C by mimicking the natural diacylglycerols produced in cells. It binds to the regulatory domain of protein kinase C, causing a conformational change that activates the enzyme. This activation leads to the phosphorylation of various target proteins, which in turn regulate multiple cellular pathways, including those involved in cell growth, differentiation, and apoptosis .
Vergleich Mit ähnlichen Verbindungen
Comparison with Structurally Similar Compounds
1,3-Dioctanoylglycerol (1,3-DiC8)
1,3-DiC8, an isomer of 1,2-DiC8, differs in acyl chain positioning (sn-1 and sn-3). Despite structural similarity, it exhibits distinct biological properties:
- PKC Activation : 1,3-DiC8 is a poor PKC activator compared to 1,2-DiC8. In human platelets and neutrophils, 1,3-DiC8 primes PLA2 activation at levels comparable to 1,2-DiC8 (~150% of control in platelets), but its inability to robustly activate PKC suggests PKC-independent pathways in PLA2 priming .
- Mitotic Regulation : In Tradescantia stamen hair cells, 1,3-DiC8 fails to reverse quin2-induced metaphase arrest or alter mitotic timing, unlike 1,2-DiC8 .
1-Oleoyl-2-Acetyl-sn-Glycerol (OAG)
OAG, a mixed-chain DAG (C18:1 at sn-1, C2:0 at sn-2), shares PKC-activating properties with 1,2-DiC8 but differs in downstream effects:
- Arachidonic Acid (AA) Release : OAG increases AA release in platelets and neutrophils (117–134% of control), slightly less than 1,2-DiC8 (150%) .
- Cell Permeability : The acetyl chain in OAG enhances membrane permeability but reduces metabolic stability compared to 1,2-DiC8 .
Phorbol Esters (e.g., PMA)
Phorbol 12-myristate 13-acetate (PMA), a potent PKC agonist, outperforms 1,2-DiC8 in PLA2 priming (400% AA release in platelets vs. 150% for 1,2-DiC8) . However, 1,2-DiC8 uniquely activates PKC isoforms insensitive to PMA in adrenal glomerulosa cells, suggesting divergent signaling mechanisms .
Structural and Functional Analysis
Acyl Chain Length and Solubility
- Medium-Chain DAGs (C8–C10) : 1,2-DiC8 and dicaprylin (C8-DAG) exhibit higher solubility than long-chain DAGs, enabling efficient cellular uptake .
- Long-Chain DAGs (e.g., 1,2-Dioleoylglycerol): These mimic endogenous DAGs but require detergents for solubilization, limiting experimental utility .
Stereochemical Specificity
The sn-1,2 configuration is critical for PKC binding. 1,3-DiC8 and other isomers fail to activate PKC due to steric incompatibility with the C1 domain .
Comparative Data Tables
Table 2: Physicochemical Properties
Compound | Acyl Chains | Solubility | Metabolic Stability |
---|---|---|---|
1,2-DiC8 | C8/C8 | High | Moderate |
1,3-DiC8 | C8/C8 | High | Low |
C8-DAG (dicaprylin) | C8/C8 | High | High |
1,2-Dioleoylglycerol | C18:1/C18:1 | Low | High |
Biologische Aktivität
1,2-Dioctanoylglycerol (1,2-DAG), also known as sn-1,2-dioctanoylglycerol or DiC8, is a synthetic diacylglycerol compound that has garnered attention for its role as a potent activator of protein kinase C (PKC). This article explores the biological activity of 1,2-DAG, detailing its mechanisms of action, effects on cellular processes, and relevant research findings.
This compound has the chemical formula C19H36O5 and a molecular weight of 344.5 g/mol. It consists of two octanoyl (C8) fatty acid chains esterified to a glycerol backbone at the sn-1 and sn-2 positions. The compound is typically a clear, colorless oil or waxy solid that is soluble in dimethyl sulfoxide and ethanol, making it suitable for laboratory applications .
1,2-DAG functions primarily by activating PKC, an enzyme involved in various cellular signaling pathways. Upon binding to PKC, 1,2-DAG induces a conformational change in the enzyme that allows it to interact with phosphatidylserine and become activated. This activation triggers downstream signaling cascades that influence cell proliferation, differentiation, and survival .
Key Effects of 1,2-DAG Activation:
- Cell Proliferation : 1,2-DAG promotes cell division through PKC-mediated pathways.
- Differentiation : It influences the differentiation of various cell types.
- Apoptosis : The compound can modulate programmed cell death processes.
- Calcium Signaling : 1,2-DAG affects intracellular calcium levels independently of PKC activation .
Table 1: Summary of Biological Activities
Case Study: Neurotransmitter Release
In a study examining the effects of 1,2-DAG on neurotransmitter release, it was found that application of 50 µg/ml of dioctanoylglycerol resulted in a significant increase (35-50%) in labeled dopamine release from rat striatal synaptosomes. This effect demonstrates the compound's ability to modulate neurotransmitter dynamics through PKC activation .
Case Study: Cardiac Function
Another study focused on the impact of 1,2-DAG on cardiac myocytes revealed that extracellular application led to a concentration-dependent inhibition of L-type calcium current (ICa,L). The half-maximum inhibitory concentration was determined to be approximately 2.2 µM. This finding suggests that 1,2-DAG may play a role in regulating cardiac excitability and contractility .
Table 2: Comparison with Related Diacylglycerols
Compound Name | Structure Type | Unique Features |
---|---|---|
1,2-Dioleoyl-glycerol | Diacylglycerol | Contains unsaturated fatty acids; different biological properties |
Phorbol 12-myristate 13-acetate | Phorbol ester | Stronger tumor-promoting activity; more complex structure |
1,3-Dioctanoyl-glycerol | Diacylglycerol | Different acyl position; less potent PKC activator compared to 1,2-isomer |
The unique positioning of acyl groups at the sn-1 and sn-2 positions distinguishes this compound from its analogs regarding its biological activity and specificity towards PKC activation .
Q & A
Basic Research Questions
Q. What experimental methods validate the role of 1,2-Dioctanoylglycerol in protein kinase C (PKC) activation?
- Methodological Answer : this compound is a synthetic diacylglycerol (DAG) analog used to study PKC activation due to its solubility and membrane permeability. Experimental validation involves PKC translocation assays, where fluorescence microscopy tracks GFP-tagged PKC isoforms relocating to membranes upon treatment. Dose-response curves (e.g., 0.5–60 µg/mL) and time-lapse imaging are critical for assessing activation kinetics. Controls should include inactive isomers (e.g., 1,3-Dioctanoylglycerol) to confirm specificity .
Q. How is this compound detected and quantified in lipid mixtures?
- Methodological Answer : Detection relies on chromatographic techniques:
- Thin-layer chromatography (TLC) : Separates lipids using silica gel plates and solvent systems (e.g., chloroform:methanol:acetic acid, 90:10:1).
- Liquid chromatography-mass spectrometry (LC-MS) : Provides high sensitivity and specificity using reverse-phase columns and electrospray ionization.
- Nuclear magnetic resonance (NMR) : Confirms structural integrity via characteristic glycerol backbone and acyl chain signals (e.g., δ 4.2–4.3 ppm for sn-1,2-glycerol protons). Calibration curves with synthetic standards ensure accurate quantification .
Q. What concentration-dependent effects does this compound exhibit in plant cell mitosis?
- Methodological Answer : In Tradescantia stamen hair cells, 0.5 µg/mL accelerates metaphase-to-anaphase transition by ~15%, while 60 µg/mL induces premature nuclear envelope breakdown. Experimental design requires precise timing:
- Late prophase treatment : Reduces metaphase duration by 28 vs. 33 minutes in controls.
- Early prophase treatment : Blocks mitosis, reverting cells to interphase.
- Use phase-contrast microscopy and synchronized cell populations to minimize variability .
Advanced Research Questions
Q. How does the timing of this compound application affect cell cycle checkpoint regulation?
- Methodological Answer : Application timing determines checkpoint engagement:
- Prometaphase : Accelerates anaphase onset by overriding spindle assembly checkpoints.
- Late metaphase : Triggers precocious sister chromatid separation, suggesting bypass of the anaphase-promoting complex (APC/C) safeguards.
- Experimental validation involves live-cell imaging paired with inhibitors (e.g., MG132 for proteasome inhibition) to dissect checkpoint pathways .
Q. Why do discrepancies arise in this compound’s biological activity across studies?
- Methodological Answer : Variability stems from:
- Acyl chain specificity : Unlike endogenous DAGs (e.g., 1-stearoyl-2-arachidonoyl), short-chain analogs like this compound lack natural membrane affinity, altering PKC binding kinetics.
- Cell type differences : Plant cells (e.g., Tradescantia) show time-dependent responses, while mammalian models (e.g., rat brain) exhibit behavioral modulation via PKC-βII.
- Standardize protocols using isoform-specific PKC inhibitors (e.g., Gö6983) and lipidomics to profile endogenous DAGs .
Q. How do in vivo and in vitro models differ in response to this compound-induced PKC activation?
- Methodological Answer :
- In vitro : PKC-βII relocation in HeLa cells peaks at 5–10 minutes post-treatment.
- In vivo : Intracerebroventricular injection in rats reduces ACTH-induced grooming by 22–31%, requiring behavioral scoring systems (e.g., 5-point scales) and PKC knockout controls.
- Consider pharmacokinetic factors (e.g., blood-brain barrier penetration) when translating findings .
Q. Safety and Application
Q. What safety protocols are recommended for handling this compound?
- Methodological Answer :
- Personal protective equipment (PPE) : Gloves, lab coat, and safety goggles.
- Disposal : Collect waste in sealed containers; avoid sewer release.
- First aid : Flush eyes with water for 15 minutes; seek medical advice if ingested.
- Refer to OSHA-aligned safety data sheets for spill management and storage (e.g., -20°C under nitrogen) .
Q. How does this compound complexation influence drug solubility in lipid-based delivery systems?
- Methodological Answer : In self-emulsifying drug delivery systems (SEDDS), this compound forms insoluble complexes with drugs like Tipranavir (log P = 6.0), reducing free drug concentration. Study equilibrium solubility via:
- Phase solubility analysis : Vary lipid:drug ratios (1:1 to 10:1) in biorelevant media (e.g., FaSSIF).
- Dynamic light scattering (DLS) : Monitor droplet size changes post-complexation.
- Optimize formulations using ternary phase diagrams .
Eigenschaften
IUPAC Name |
(3-hydroxy-2-octanoyloxypropyl) octanoate | |
---|---|---|
Source | PubChem | |
URL | https://pubchem.ncbi.nlm.nih.gov | |
Description | Data deposited in or computed by PubChem | |
InChI |
InChI=1S/C19H36O5/c1-3-5-7-9-11-13-18(21)23-16-17(15-20)24-19(22)14-12-10-8-6-4-2/h17,20H,3-16H2,1-2H3 | |
Source | PubChem | |
URL | https://pubchem.ncbi.nlm.nih.gov | |
Description | Data deposited in or computed by PubChem | |
InChI Key |
ZQBULZYTDGUSSK-UHFFFAOYSA-N | |
Source | PubChem | |
URL | https://pubchem.ncbi.nlm.nih.gov | |
Description | Data deposited in or computed by PubChem | |
Canonical SMILES |
CCCCCCCC(=O)OCC(CO)OC(=O)CCCCCCC | |
Source | PubChem | |
URL | https://pubchem.ncbi.nlm.nih.gov | |
Description | Data deposited in or computed by PubChem | |
Molecular Formula |
C19H36O5 | |
Source | PubChem | |
URL | https://pubchem.ncbi.nlm.nih.gov | |
Description | Data deposited in or computed by PubChem | |
DSSTOX Substance ID |
DTXSID00910175 | |
Record name | 3-Hydroxypropane-1,2-diyl dioctanoate | |
Source | EPA DSSTox | |
URL | https://comptox.epa.gov/dashboard/DTXSID00910175 | |
Description | DSSTox provides a high quality public chemistry resource for supporting improved predictive toxicology. | |
Molecular Weight |
344.5 g/mol | |
Source | PubChem | |
URL | https://pubchem.ncbi.nlm.nih.gov | |
Description | Data deposited in or computed by PubChem | |
CAS No. |
1069-87-0 | |
Record name | Monoctanoin Component C | |
Source | ChemIDplus | |
URL | https://pubchem.ncbi.nlm.nih.gov/substance/?source=chemidplus&sourceid=0001069870 | |
Description | ChemIDplus is a free, web search system that provides access to the structure and nomenclature authority files used for the identification of chemical substances cited in National Library of Medicine (NLM) databases, including the TOXNET system. | |
Record name | 3-Hydroxypropane-1,2-diyl dioctanoate | |
Source | EPA DSSTox | |
URL | https://comptox.epa.gov/dashboard/DTXSID00910175 | |
Description | DSSTox provides a high quality public chemistry resource for supporting improved predictive toxicology. | |
Record name | GLYCERYL 1,2-DICAPRYLATE | |
Source | FDA Global Substance Registration System (GSRS) | |
URL | https://gsrs.ncats.nih.gov/ginas/app/beta/substances/SA9937IP23 | |
Description | The FDA Global Substance Registration System (GSRS) enables the efficient and accurate exchange of information on what substances are in regulated products. Instead of relying on names, which vary across regulatory domains, countries, and regions, the GSRS knowledge base makes it possible for substances to be defined by standardized, scientific descriptions. | |
Explanation | Unless otherwise noted, the contents of the FDA website (www.fda.gov), both text and graphics, are not copyrighted. They are in the public domain and may be republished, reprinted and otherwise used freely by anyone without the need to obtain permission from FDA. Credit to the U.S. Food and Drug Administration as the source is appreciated but not required. | |
Retrosynthesis Analysis
AI-Powered Synthesis Planning: Our tool employs the Template_relevance Pistachio, Template_relevance Bkms_metabolic, Template_relevance Pistachio_ringbreaker, Template_relevance Reaxys, Template_relevance Reaxys_biocatalysis model, leveraging a vast database of chemical reactions to predict feasible synthetic routes.
One-Step Synthesis Focus: Specifically designed for one-step synthesis, it provides concise and direct routes for your target compounds, streamlining the synthesis process.
Accurate Predictions: Utilizing the extensive PISTACHIO, BKMS_METABOLIC, PISTACHIO_RINGBREAKER, REAXYS, REAXYS_BIOCATALYSIS database, our tool offers high-accuracy predictions, reflecting the latest in chemical research and data.
Strategy Settings
Precursor scoring | Relevance Heuristic |
---|---|
Min. plausibility | 0.01 |
Model | Template_relevance |
Template Set | Pistachio/Bkms_metabolic/Pistachio_ringbreaker/Reaxys/Reaxys_biocatalysis |
Top-N result to add to graph | 6 |
Feasible Synthetic Routes
Haftungsausschluss und Informationen zu In-Vitro-Forschungsprodukten
Bitte beachten Sie, dass alle Artikel und Produktinformationen, die auf BenchChem präsentiert werden, ausschließlich zu Informationszwecken bestimmt sind. Die auf BenchChem zum Kauf angebotenen Produkte sind speziell für In-vitro-Studien konzipiert, die außerhalb lebender Organismen durchgeführt werden. In-vitro-Studien, abgeleitet von dem lateinischen Begriff "in Glas", beinhalten Experimente, die in kontrollierten Laborumgebungen unter Verwendung von Zellen oder Geweben durchgeführt werden. Es ist wichtig zu beachten, dass diese Produkte nicht als Arzneimittel oder Medikamente eingestuft sind und keine Zulassung der FDA für die Vorbeugung, Behandlung oder Heilung von medizinischen Zuständen, Beschwerden oder Krankheiten erhalten haben. Wir müssen betonen, dass jede Form der körperlichen Einführung dieser Produkte in Menschen oder Tiere gesetzlich strikt untersagt ist. Es ist unerlässlich, sich an diese Richtlinien zu halten, um die Einhaltung rechtlicher und ethischer Standards in Forschung und Experiment zu gewährleisten.