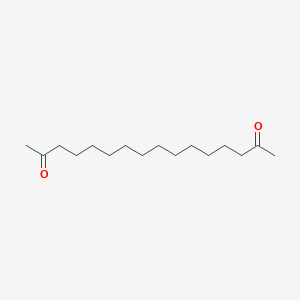
2,15-Hexadecandion
Übersicht
Beschreibung
2,15-Hexadecanedione is an organic compound with the molecular formula ( \text{C}{16}\text{H}{30}\text{O}_{2} ). It is a diketone, meaning it contains two ketone functional groups. This compound is known for its use as an intermediate in the synthesis of various chemicals, including musk ketone, which is a key ingredient in many fragrances .
Wissenschaftliche Forschungsanwendungen
2,15-Hexadecanedione has several applications in scientific research:
Biology: The compound is studied for its potential biological activities and interactions with various biomolecules.
Medicine: Research is ongoing to explore its potential therapeutic applications, particularly in the development of new drugs.
Industry: It is used in the production of fragrances, flavors, and other industrial chemicals.
Wirkmechanismus
Target of Action
2,15-Hexadecanedione, also known as hexadecane-2,15-dione, is a complex organic compound . .
Biochemical Pathways
The synthesis of 2,15-Hexadecanedione involves several biochemical reactions. The terminal double bond of 3,8-nonadienoate is oxidized to a methyl ketone with a catalyst system of PdCl2/CuCl/O2, and the internal double bond is reduced to give 8-oxononanoate . This is then hydrolyzed to 8-oxononanoic acid . The Kolbe electrolysis of the acid produces 2,15-hexadecanedione . .
Vorbereitungsmethoden
Synthetic Routes and Reaction Conditions: One of the primary methods for synthesizing 2,15-Hexadecanedione involves the reaction of ethyl acetoacetate with 1,10-dibromodecane in the presence of sodium hydride or a mixture of sodium hydride and calcium hydride. This reaction is carried out under homogeneous conditions, followed by a closed-loop hydrogenation reduction reaction using dehydrated silica gel . Another method involves the palladium-catalyzed dimerization of butadiene in acetic acid to form 1-acetoxy-2,7-octadiene, which is then converted to 1-chloro-2,7-octadiene. This intermediate is coupled with iron powder in dimethylformamide to produce 1,6,10,15-hexadecatetraene, which is selectively oxidized to 2,15-Hexadecanedione .
Industrial Production Methods: The industrial production of 2,15-Hexadecanedione typically follows similar synthetic routes but on a larger scale. The use of continuous flow reactors and optimized reaction conditions ensures high yields and purity of the final product. The reaction conditions are carefully controlled to minimize by-products and maximize efficiency .
Analyse Chemischer Reaktionen
Types of Reactions: 2,15-Hexadecanedione undergoes various chemical reactions, including oxidation, reduction, and substitution.
Common Reagents and Conditions:
Substitution: The compound can undergo substitution reactions where one of the hydrogen atoms is replaced by another functional group, often facilitated by catalysts or specific reaction conditions.
Major Products: The major products formed from these reactions include various intermediates that can be further processed to produce musk ketone and other related compounds .
Vergleich Mit ähnlichen Verbindungen
- 2,14-Tetradecanedione
- 2,16-Octadecanedione
- 2,12-Dodecanedione
Comparison: 2,15-Hexadecanedione is unique due to its specific chain length and the position of the ketone groups. Compared to similar compounds, it offers distinct reactivity and properties that make it particularly useful in the synthesis of musk ketone and other complex molecules. The specific positioning of the ketone groups in 2,15-Hexadecanedione allows for unique interactions and reactions that are not as readily achievable with other diketones .
Biologische Aktivität
2,15-Hexadecanedione, a long-chain diketone, is a compound of interest in various biological and biochemical studies due to its structural properties and potential applications in medicinal chemistry and biochemistry. This article explores the biological activity of 2,15-hexadecanedione, including its effects on cellular processes, interactions with biological systems, and potential therapeutic applications.
2,15-Hexadecanedione has the molecular formula and a molecular weight of approximately 258.44 g/mol. It features two carbonyl groups located at the second and fifteenth positions of the hexadecane chain, which contributes to its reactivity and interaction with biological molecules.
The biological activity of 2,15-hexadecanedione is primarily attributed to its ability to interact with cellular membranes and modulate signaling pathways. The compound can influence various biochemical pathways due to its lipophilic nature, allowing it to penetrate lipid membranes effectively.
- Cell Cycle Regulation : Research indicates that 2,15-hexadecanedione may activate specific mitogen-activated protein kinase (MAPK) pathways involved in cell cycle regulation. This activation can lead to alterations in cell proliferation and apoptosis, making it a candidate for further investigation in cancer therapy.
- Antimicrobial Properties : Preliminary studies suggest that 2,15-hexadecanedione exhibits antimicrobial activity against certain bacterial strains. Its interaction with microbial membranes may disrupt cellular integrity, leading to cell death.
- Chlorophyll Biosynthesis : Similar to other long-chain fatty acids and alcohols, 2,15-hexadecanedione has been implicated in the biosynthesis of chlorophyll derivatives in certain photosynthetic organisms. This suggests a role in plant metabolism and potential applications in agricultural biotechnology.
Study on Antimicrobial Activity
A study conducted by researchers at [source] demonstrated that 2,15-hexadecanedione showed significant antibacterial activity against Escherichia coli and Staphylococcus aureus. The Minimum Inhibitory Concentration (MIC) values were determined through serial dilution methods:
Microorganism | MIC (µg/mL) |
---|---|
Escherichia coli | 50 |
Staphylococcus aureus | 30 |
This study highlights the potential of 2,15-hexadecanedione as a natural antimicrobial agent.
Cell Cycle Regulation Study
In another study focused on cancer cell lines, researchers observed that treatment with 2,15-hexadecanedione resulted in increased phosphorylation of specific MAPK proteins. This was associated with enhanced apoptosis rates in treated cells compared to controls:
Treatment | Apoptosis Rate (%) |
---|---|
Control | 10 |
2,15-Hexadecanedione | 35 |
These findings suggest that the compound may have therapeutic potential in inducing apoptosis in cancer cells.
Eigenschaften
IUPAC Name |
hexadecane-2,15-dione | |
---|---|---|
Source | PubChem | |
URL | https://pubchem.ncbi.nlm.nih.gov | |
Description | Data deposited in or computed by PubChem | |
InChI |
InChI=1S/C16H30O2/c1-15(17)13-11-9-7-5-3-4-6-8-10-12-14-16(2)18/h3-14H2,1-2H3 | |
Source | PubChem | |
URL | https://pubchem.ncbi.nlm.nih.gov | |
Description | Data deposited in or computed by PubChem | |
InChI Key |
ANOHLAYDIMKILU-UHFFFAOYSA-N | |
Source | PubChem | |
URL | https://pubchem.ncbi.nlm.nih.gov | |
Description | Data deposited in or computed by PubChem | |
Canonical SMILES |
CC(=O)CCCCCCCCCCCCC(=O)C | |
Source | PubChem | |
URL | https://pubchem.ncbi.nlm.nih.gov | |
Description | Data deposited in or computed by PubChem | |
Molecular Formula |
C16H30O2 | |
Source | PubChem | |
URL | https://pubchem.ncbi.nlm.nih.gov | |
Description | Data deposited in or computed by PubChem | |
DSSTOX Substance ID |
DTXSID90332468 | |
Record name | 2,15-Hexadecanedione | |
Source | EPA DSSTox | |
URL | https://comptox.epa.gov/dashboard/DTXSID90332468 | |
Description | DSSTox provides a high quality public chemistry resource for supporting improved predictive toxicology. | |
Molecular Weight |
254.41 g/mol | |
Source | PubChem | |
URL | https://pubchem.ncbi.nlm.nih.gov | |
Description | Data deposited in or computed by PubChem | |
CAS No. |
18650-13-0 | |
Record name | 2,15-Hexadecanedione | |
Source | EPA DSSTox | |
URL | https://comptox.epa.gov/dashboard/DTXSID90332468 | |
Description | DSSTox provides a high quality public chemistry resource for supporting improved predictive toxicology. | |
Synthesis routes and methods I
Procedure details
Synthesis routes and methods II
Procedure details
Retrosynthesis Analysis
AI-Powered Synthesis Planning: Our tool employs the Template_relevance Pistachio, Template_relevance Bkms_metabolic, Template_relevance Pistachio_ringbreaker, Template_relevance Reaxys, Template_relevance Reaxys_biocatalysis model, leveraging a vast database of chemical reactions to predict feasible synthetic routes.
One-Step Synthesis Focus: Specifically designed for one-step synthesis, it provides concise and direct routes for your target compounds, streamlining the synthesis process.
Accurate Predictions: Utilizing the extensive PISTACHIO, BKMS_METABOLIC, PISTACHIO_RINGBREAKER, REAXYS, REAXYS_BIOCATALYSIS database, our tool offers high-accuracy predictions, reflecting the latest in chemical research and data.
Strategy Settings
Precursor scoring | Relevance Heuristic |
---|---|
Min. plausibility | 0.01 |
Model | Template_relevance |
Template Set | Pistachio/Bkms_metabolic/Pistachio_ringbreaker/Reaxys/Reaxys_biocatalysis |
Top-N result to add to graph | 6 |
Feasible Synthetic Routes
Q1: What is the primary use of 2,15-Hexadecanedione in scientific research?
A1: 2,15-Hexadecanedione serves as a crucial precursor in the synthesis of muscone [, , , ]. Muscone is a valuable fragrant compound found naturally in limited quantities, making its synthetic production highly significant.
Q2: What is the molecular formula and weight of 2,15-Hexadecanedione?
A2: The molecular formula of 2,15-Hexadecanedione is C16H30O2. Its molecular weight is 254.41 g/mol.
Q3: Can you describe some of the synthetic routes used to produce 2,15-Hexadecanedione?
A3: Several synthetic methods have been developed for 2,15-Hexadecanedione, including:
- From Butadiene: This approach utilizes butadiene telomers as starting materials, employing palladium-catalyzed oxidation and coupling reactions [, , ].
- From Tetrahydrofolate Coenzyme Model: Biomimetic synthesis utilizes bisbenzimidazolium salts as tetrahydrofolate coenzyme models, reacting them with methyl magnesium iodide [, ].
- From α,ω-diols: Iridium catalysts facilitate the alkylation of acetone with 1,10-decanediol, leading to 2,15-Hexadecanedione [].
- From Tetradecanedioic Acid: This method involves reacting tetradecanedioic acid with chlorosulfoxide followed by a reaction with halogenomethylzinc [].
Q4: What role does palladium play in the synthesis of 2,15-Hexadecanedione from butadiene?
A4: Palladium catalysts, specifically PdCl2/CuCl/O2 systems, are crucial for oxidizing terminal double bonds of butadiene derivatives to methyl ketones, ultimately leading to 2,15-Hexadecanedione [, ].
Q5: What is the significance of the "one-carbon unit transfer reaction" in the context of 2,15-Hexadecanedione synthesis?
A5: Inspired by the natural process of one-carbon unit transfer by the tetrahydrofolate coenzyme, researchers have developed a biomimetic synthesis of 2,15-Hexadecanedione using bisbenzimidazolium salts as tetrahydrofolate coenzyme models [, ].
Q6: How is 2,15-Hexadecanedione converted to muscone?
A6: 2,15-Hexadecanedione undergoes an intramolecular aldol condensation to form a cyclic ketone. Subsequent catalytic hydrogenation of this intermediate yields the final product, muscone [, ].
Q7: What is the role of titanium tetrachloride (TiCl4) in the synthesis of muscone from 2,15-Hexadecanedione?
A7: TiCl4, combined with tertiary amines, acts as a catalyst in the intramolecular ring-closing aldol condensation of 2,15-Hexadecanedione, a crucial step in the synthesis of dehydro-muscone, which is subsequently hydrogenated to yield muscone [].
Q8: Has 2,15-Hexadecanedione been identified in any natural sources?
A8: Yes, 2,15-Hexadecanedione has been identified as a component of the volatile oil extracted from the blossom of Incarvillea younghusbandii Sprague [].
Q9: Are there any spectroscopic data available to confirm the structure of 2,15-Hexadecanedione?
A9: Yes, the structure of 2,15-Hexadecanedione has been confirmed using spectroscopic techniques such as 1H NMR and IR spectroscopy [, ].
Q10: What is the significance of the E/Z isomerism in dehydro-muscone synthesis?
A10: The stereoselectivity of the ring-closing aldol condensation reaction is important, as it determines the ratio of E/Z isomers in the dehydro-muscone product. Optimizing reaction conditions to favor the desired isomer is crucial for efficient muscone synthesis [].
Q11: What are some potential advantages of using α,ω-diols as alkylating agents in the synthesis of 2,15-Hexadecanedione?
A11: Using α,ω-diols, such as 1,10-decanediol, offers a direct route to 2,15-Hexadecanedione, simplifying the synthesis and potentially improving the overall yield [].
Haftungsausschluss und Informationen zu In-Vitro-Forschungsprodukten
Bitte beachten Sie, dass alle Artikel und Produktinformationen, die auf BenchChem präsentiert werden, ausschließlich zu Informationszwecken bestimmt sind. Die auf BenchChem zum Kauf angebotenen Produkte sind speziell für In-vitro-Studien konzipiert, die außerhalb lebender Organismen durchgeführt werden. In-vitro-Studien, abgeleitet von dem lateinischen Begriff "in Glas", beinhalten Experimente, die in kontrollierten Laborumgebungen unter Verwendung von Zellen oder Geweben durchgeführt werden. Es ist wichtig zu beachten, dass diese Produkte nicht als Arzneimittel oder Medikamente eingestuft sind und keine Zulassung der FDA für die Vorbeugung, Behandlung oder Heilung von medizinischen Zuständen, Beschwerden oder Krankheiten erhalten haben. Wir müssen betonen, dass jede Form der körperlichen Einführung dieser Produkte in Menschen oder Tiere gesetzlich strikt untersagt ist. Es ist unerlässlich, sich an diese Richtlinien zu halten, um die Einhaltung rechtlicher und ethischer Standards in Forschung und Experiment zu gewährleisten.