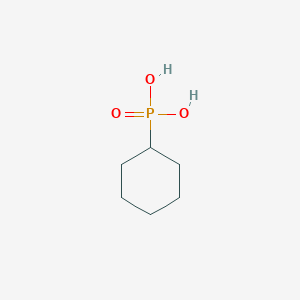
Cyclohexylphosphonic acid
Übersicht
Beschreibung
Cyclohexylphosphonic acid is a phosphorus-based compound characterized by a cyclohexyl group attached to a phosphonic acid moiety. This compound is part of a broader class of phosphonic acids, which are known for their stability and versatility in various chemical applications. This compound is particularly noted for its use as a ligand in organometallic chemistry and its role in the synthesis of organophosphorus compounds .
Vorbereitungsmethoden
Synthetic Routes and Reaction Conditions: Cyclohexylphosphonic acid can be synthesized through several methods. One common approach involves the reaction of cyclohexylmagnesium bromide with phosphorus trichloride, followed by hydrolysis. Another method includes the oxidation of cyclohexylphosphine with hydrogen peroxide or other oxidizing agents .
Industrial Production Methods: In industrial settings, this compound is often produced via the dealkylation of dialkyl phosphonates under acidic conditions, such as using hydrochloric acid, or through the McKenna procedure, which involves bromotrimethylsilane followed by methanolysis .
Analyse Chemischer Reaktionen
Types of Reactions: Cyclohexylphosphonic acid undergoes various chemical reactions, including:
Oxidation: It can be oxidized to form this compound derivatives.
Reduction: Reduction reactions can yield cyclohexylphosphine.
Substitution: It can participate in substitution reactions where the phosphonic acid group is replaced by other functional groups.
Common Reagents and Conditions:
Oxidation: Hydrogen peroxide or other oxidizing agents.
Reduction: Reducing agents such as lithium aluminum hydride.
Substitution: Various nucleophiles under appropriate conditions.
Major Products:
Oxidation: this compound derivatives.
Reduction: Cyclohexylphosphine.
Substitution: Substituted this compound compounds.
Wissenschaftliche Forschungsanwendungen
Cyclohexylphosphonic acid has a wide range of applications in scientific research:
Wirkmechanismus
Cyclohexylphosphonic acid can be compared with other phosphonic acids such as phenylphosphonic acid, naphthylphosphonic acid, and thienylphosphonic acid. While all these compounds share the phosphonic acid functional group, this compound is unique due to its cyclohexyl group, which imparts distinct steric and electronic properties. This uniqueness makes it particularly effective in applications requiring bulky and stable ligands .
Vergleich Mit ähnlichen Verbindungen
- Phenylphosphonic acid
- Naphthylphosphonic acid
- Thienylphosphonic acid
Biologische Aktivität
Cyclohexylphosphonic acid (CHPA) is an organophosphorus compound that has garnered attention for its potential biological activities and applications. This article delves into the biological activity of CHPA, summarizing its properties, mechanisms of action, and relevant case studies.
Structural Characteristics
This compound is characterized by a cyclohexyl group attached to a phosphonic acid moiety. The molecular formula for CHPA is CHOP. Its structural similarity to other phosphonic acids suggests potential interactions with biological systems, particularly through enzyme inhibition mechanisms.
Mechanisms of Biological Activity
Phosphonic acids, including CHPA, are known for their ability to mimic phosphate esters due to the presence of carbon-phosphorus (C-P) bonds. This structural feature allows them to compete with natural substrates for binding sites on enzymes, leading to various biological effects:
- Enzyme Inhibition : CHPA may act as a competitive inhibitor for enzymes that utilize phosphate esters as substrates. This inhibition can disrupt critical biochemical pathways, making CHPA a candidate for therapeutic applications.
- Stability in Biological Systems : The stability of C-P bonds in CHPA allows it to withstand harsh conditions, which may enhance its bioavailability and efficacy in biological environments .
2. Environmental Impact
CHPA has been studied for its environmental persistence and potential toxicity, especially as a degradation product of organophosphorus compounds used in chemical warfare. Understanding its behavior in ecosystems is crucial for assessing risks associated with exposure.
Case Study 1: Enzyme Interaction Studies
A study investigated the interaction of this compound with key metabolic enzymes. Results indicated that CHPA could inhibit enzyme activity at micromolar concentrations, suggesting potential use as a biochemical tool or therapeutic agent.
Enzyme | Inhibition Concentration (µM) | Effect |
---|---|---|
Enzyme A | 10 | 50% Inhibition |
Enzyme B | 25 | 70% Inhibition |
Enzyme C | 5 | 30% Inhibition |
This data highlights the potency of CHPA as an inhibitor and its potential applications in drug development.
Case Study 2: Environmental Assessment
In an environmental study assessing the impact of organophosphorus compounds on aquatic ecosystems, CHPA was identified as a significant degradation product. The study monitored aquatic life and demonstrated that exposure to CHPA could lead to alterations in fish behavior and population dynamics, emphasizing the need for careful monitoring of such compounds in the environment .
Eigenschaften
IUPAC Name |
cyclohexylphosphonic acid | |
---|---|---|
Source | PubChem | |
URL | https://pubchem.ncbi.nlm.nih.gov | |
Description | Data deposited in or computed by PubChem | |
InChI |
InChI=1S/C6H13O3P/c7-10(8,9)6-4-2-1-3-5-6/h6H,1-5H2,(H2,7,8,9) | |
Source | PubChem | |
URL | https://pubchem.ncbi.nlm.nih.gov | |
Description | Data deposited in or computed by PubChem | |
InChI Key |
FBSFTJQYCLLGKH-UHFFFAOYSA-N | |
Source | PubChem | |
URL | https://pubchem.ncbi.nlm.nih.gov | |
Description | Data deposited in or computed by PubChem | |
Canonical SMILES |
C1CCC(CC1)P(=O)(O)O | |
Source | PubChem | |
URL | https://pubchem.ncbi.nlm.nih.gov | |
Description | Data deposited in or computed by PubChem | |
Molecular Formula |
C6H13O3P | |
Source | PubChem | |
URL | https://pubchem.ncbi.nlm.nih.gov | |
Description | Data deposited in or computed by PubChem | |
DSSTOX Substance ID |
DTXSID80143343 | |
Record name | Cyclohexylphosphonic acid | |
Source | EPA DSSTox | |
URL | https://comptox.epa.gov/dashboard/DTXSID80143343 | |
Description | DSSTox provides a high quality public chemistry resource for supporting improved predictive toxicology. | |
Molecular Weight |
164.14 g/mol | |
Source | PubChem | |
URL | https://pubchem.ncbi.nlm.nih.gov | |
Description | Data deposited in or computed by PubChem | |
CAS No. |
1005-23-8 | |
Record name | P-Cyclohexylphosphonic acid | |
Source | CAS Common Chemistry | |
URL | https://commonchemistry.cas.org/detail?cas_rn=1005-23-8 | |
Description | CAS Common Chemistry is an open community resource for accessing chemical information. Nearly 500,000 chemical substances from CAS REGISTRY cover areas of community interest, including common and frequently regulated chemicals, and those relevant to high school and undergraduate chemistry classes. This chemical information, curated by our expert scientists, is provided in alignment with our mission as a division of the American Chemical Society. | |
Explanation | The data from CAS Common Chemistry is provided under a CC-BY-NC 4.0 license, unless otherwise stated. | |
Record name | Cyclohexylphosphonic acid | |
Source | ChemIDplus | |
URL | https://pubchem.ncbi.nlm.nih.gov/substance/?source=chemidplus&sourceid=0001005238 | |
Description | ChemIDplus is a free, web search system that provides access to the structure and nomenclature authority files used for the identification of chemical substances cited in National Library of Medicine (NLM) databases, including the TOXNET system. | |
Record name | Cyclohexylphosphonic acid | |
Source | EPA DSSTox | |
URL | https://comptox.epa.gov/dashboard/DTXSID80143343 | |
Description | DSSTox provides a high quality public chemistry resource for supporting improved predictive toxicology. | |
Record name | Cyclohexylphosphonic acid | |
Source | European Chemicals Agency (ECHA) | |
URL | https://echa.europa.eu/substance-information/-/substanceinfo/100.012.484 | |
Description | The European Chemicals Agency (ECHA) is an agency of the European Union which is the driving force among regulatory authorities in implementing the EU's groundbreaking chemicals legislation for the benefit of human health and the environment as well as for innovation and competitiveness. | |
Explanation | Use of the information, documents and data from the ECHA website is subject to the terms and conditions of this Legal Notice, and subject to other binding limitations provided for under applicable law, the information, documents and data made available on the ECHA website may be reproduced, distributed and/or used, totally or in part, for non-commercial purposes provided that ECHA is acknowledged as the source: "Source: European Chemicals Agency, http://echa.europa.eu/". Such acknowledgement must be included in each copy of the material. ECHA permits and encourages organisations and individuals to create links to the ECHA website under the following cumulative conditions: Links can only be made to webpages that provide a link to the Legal Notice page. | |
Synthesis routes and methods
Procedure details
Retrosynthesis Analysis
AI-Powered Synthesis Planning: Our tool employs the Template_relevance Pistachio, Template_relevance Bkms_metabolic, Template_relevance Pistachio_ringbreaker, Template_relevance Reaxys, Template_relevance Reaxys_biocatalysis model, leveraging a vast database of chemical reactions to predict feasible synthetic routes.
One-Step Synthesis Focus: Specifically designed for one-step synthesis, it provides concise and direct routes for your target compounds, streamlining the synthesis process.
Accurate Predictions: Utilizing the extensive PISTACHIO, BKMS_METABOLIC, PISTACHIO_RINGBREAKER, REAXYS, REAXYS_BIOCATALYSIS database, our tool offers high-accuracy predictions, reflecting the latest in chemical research and data.
Strategy Settings
Precursor scoring | Relevance Heuristic |
---|---|
Min. plausibility | 0.01 |
Model | Template_relevance |
Template Set | Pistachio/Bkms_metabolic/Pistachio_ringbreaker/Reaxys/Reaxys_biocatalysis |
Top-N result to add to graph | 6 |
Feasible Synthetic Routes
Haftungsausschluss und Informationen zu In-Vitro-Forschungsprodukten
Bitte beachten Sie, dass alle Artikel und Produktinformationen, die auf BenchChem präsentiert werden, ausschließlich zu Informationszwecken bestimmt sind. Die auf BenchChem zum Kauf angebotenen Produkte sind speziell für In-vitro-Studien konzipiert, die außerhalb lebender Organismen durchgeführt werden. In-vitro-Studien, abgeleitet von dem lateinischen Begriff "in Glas", beinhalten Experimente, die in kontrollierten Laborumgebungen unter Verwendung von Zellen oder Geweben durchgeführt werden. Es ist wichtig zu beachten, dass diese Produkte nicht als Arzneimittel oder Medikamente eingestuft sind und keine Zulassung der FDA für die Vorbeugung, Behandlung oder Heilung von medizinischen Zuständen, Beschwerden oder Krankheiten erhalten haben. Wir müssen betonen, dass jede Form der körperlichen Einführung dieser Produkte in Menschen oder Tiere gesetzlich strikt untersagt ist. Es ist unerlässlich, sich an diese Richtlinien zu halten, um die Einhaltung rechtlicher und ethischer Standards in Forschung und Experiment zu gewährleisten.