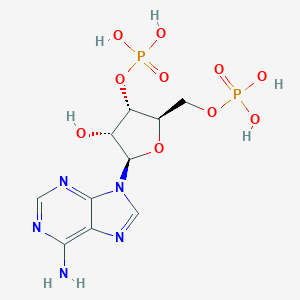
Adenosine-3'-5'-diphosphate
Übersicht
Beschreibung
Adenosine-3'-5'-diphosphate (PAP) is a dinucleotide cofactor critical in sulfotransferase (SULT)-catalyzed reactions. Structurally, it consists of adenosine with phosphate groups at the 3' and 5' positions of the ribose ring. PAP is the desulfated product of adenosine-3'-phosphate-5'-phosphosulfate (PAPS), the universal sulfate donor in biological sulfation. After PAPS transfers its sulfate group to substrates (e.g., hormones, xenobiotics), PAP remains tightly bound to SULTs, acting as a competitive inhibitor that must be recycled to sustain enzymatic activity . PAP’s role extends beyond sulfation; it is also implicated in phosphatase interactions and structural studies of enzyme-ligand complexes .
Biologische Aktivität
Adenosine-3',5'-diphosphate (PAP), also known as 3',5'-adenosine diphosphate, is a nucleotide that plays a crucial role in various biological processes. This article provides a comprehensive overview of its biological activity, including its biochemical pathways, cellular functions, and implications in health and disease.
PAP is structurally similar to adenosine diphosphate (ADP) but differs in its phosphate group arrangement. It contains two phosphate groups attached to the ribose sugar at the 3' and 5' positions, along with the adenine base. The conversion of PAP to 3'-phosphoadenosine 5'-phosphosulfate (PAPS) is catalyzed by sulfotransferases, which facilitate the transfer of sulfate groups to various substrates, a process essential for cellular communication and metabolic regulation .
Biological Functions
1. Sulfation Reactions
- PAP is involved in sulfation reactions through its conversion to PAPS. This reaction is critical for the detoxification of xenobiotics and the metabolism of hormones and neurotransmitters. Sulfotransferases utilize PAPS as a sulfate donor in these processes .
2. Role in Cell Signaling
- PAP has been implicated in cell signaling pathways, particularly in the modulation of cyclic adenosine monophosphate (cAMP) levels. It acts as a regulator of various physiological responses, including hormone secretion and neuronal signaling .
3. Toxicity and Regulation
- Accumulation of PAP can be toxic to cells; thus, phosphatases that convert PAP back to adenosine monophosphate (AMP) are vital for maintaining cellular homeostasis. These enzymes are sensitive to lithium, suggesting a potential therapeutic target for mood disorders like bipolar depression .
Case Study 1: Nephrogenic Diabetes Insipidus
A study investigated the effects of exogenous PAP on urinary output in children with nephrogenic diabetes insipidus. Despite expectations, administration of PAP did not reduce urine volume; instead, it paradoxically increased urine output by up to 188% when combined with vasopressin . This finding highlights the complex role of nucleotide signaling in renal function.
Case Study 2: Bipolar Disorder
Research has shown that lithium treatment may influence PAP levels, suggesting that modulation of PAP phosphatase activity could be beneficial in managing bipolar disorder symptoms. This connection emphasizes the importance of PAP in neuropsychiatric health .
Table 1: Enzymatic Reactions Involving PAP
Reaction | Enzyme | Product |
---|---|---|
PAP + H2O → AMP + Pi | Phosphatase | Adenosine Monophosphate (AMP) |
PAPS + Acceptor → Sulfated Product | Sulfotransferase | Sulfated Metabolite |
PAP + Thioredoxin → Reduced Thioredoxin + AMP | Glutaredoxin | Reduced Thioredoxin |
Table 2: Clinical Implications of PAP Levels
Condition | Effect on PAP Levels | Implications |
---|---|---|
Bipolar Disorder | Increased | Potential target for therapy |
Nephrogenic Diabetes Insipidus | Unchanged or increased | Altered renal response |
Liver Disease | Decreased | Impaired sulfation processes |
Wissenschaftliche Forschungsanwendungen
Molecular Mechanisms and Inhibition Studies
ADP has been studied for its inhibitory effects on specific enzymes. For example, research has shown that adenosine 3',5'-bisphosphate (pAp), a derivative of ADP, inhibits the nuclease activity of the exoribonuclease DXO, which is involved in pre-mRNA capping quality control. Structural analysis revealed that pAp occupies the active site of DXO, acting as a competitive inhibitor. This discovery provides insights into the molecular mechanisms underlying RNA processing and potential therapeutic targets for diseases related to RNA metabolism .
Wound Healing Applications
Recent studies have demonstrated that ADP can significantly enhance wound healing processes, particularly in diabetic models. In experiments with diabetic mice, local application of ADP accelerated skin wound healing by promoting new tissue formation and increasing collagen deposition. The mechanism involves activation of P2Y receptors, which are pivotal in mediating inflammatory responses and tissue repair. ADP treatment was shown to reduce reactive oxygen species (ROS) levels and modulate various immune cell populations in the wound area, suggesting its role as a pro-resolution mediator in chronic wounds .
Biochemical Assays and Analytical Applications
ADP is frequently utilized in biochemical assays to study enzyme activities and metabolic pathways. It serves as a substrate or standard in various analytical techniques, such as high-performance thin-layer chromatography (HPTLC) and enzyme activity assays. For instance, ADP has been employed to assess the activities of specific proteins involved in nucleotide metabolism, thus contributing to our understanding of cellular energy dynamics .
Drug Development and Pharmacological Research
In pharmacological contexts, ADP is being investigated for its potential therapeutic applications. It is classified as an experimental small molecule with implications in drug development due to its roles in purinergic signaling pathways. Research into ADP's interactions with specific receptors could lead to novel treatments for conditions such as vascular diseases and inflammatory disorders .
Case Study: Wound Healing in Diabetic Mice
- Objective : To evaluate the effects of ADP on wound healing.
- Method : Full-thickness excisional wound model in diabetic mice.
- Findings :
- Enhanced collagen deposition.
- Increased expression of P2Y receptors.
- Reduced levels of TNF-α and ROS.
- Improved recruitment of immune cells involved in tissue repair.
This study underlines the therapeutic potential of ADP in managing chronic wounds associated with diabetes .
Summary Table of Applications
Analyse Chemischer Reaktionen
Sulfotransferase-Mediated Sulfate Transfer
PAP is generated during sulfotransferase (ST) reactions when 3'-phosphoadenosine 5'-phosphosulfate (PAPS) donates a sulfate group to substrates. This reaction is reversible, with PAP acting as a byproduct:
Reaction | Enzyme Involved | Role of PAP |
---|---|---|
PAPS + substrate → Sulfated substrate + PAP | Sulfotransferase (ST) | Byproduct recycling |
-
Mechanism : The sulfate group from PAPS is transferred to acceptors (e.g., tyrosine residues in proteins or small molecules like steroids), forming PAP .
-
Biological Impact : PAP accumulation inhibits critical enzymes (e.g., Xrn1, DXO) by mimicking RNA substrates, disrupting RNA processing .
Hydrolysis to AMP and Phosphate
PAP is hydrolyzed to adenosine 5'-monophosphate (AMP) and inorganic phosphate (Pi) by lithium-sensitive phosphatases, preventing cellular toxicity:
Reaction | Enzyme Involved | Catalytic Features |
---|---|---|
PAP + H₂O → AMP + Pi | 3'(2'),5'-Bisphosphate nucleotidase (CysQ/Hal2) | Requires Mg²⁺; inhibited by Li⁺ |
-
Structural Basis : The 3'-phosphate of PAP binds to a conserved phosphatase active site, with Mg²⁺ ions stabilizing the transition state .
-
Toxicity Mitigation : Lithium inhibits Hal2, leading to PAP accumulation and growth defects in yeast, highlighting its regulatory role .
Redox Reactions with Thioredoxin/Glutaredoxin
PAP is regenerated from PAPS in sulfur assimilation pathways involving thioredoxin (Trx) or glutaredoxin (Grx):
Reaction | Redox Partner | Electron Pathway |
---|---|---|
PAPS + Reduced Trx/Grx → Sulfated product + PAP + Oxidized Trx/Grx | Thioredoxin/Glutaredoxin | Facilitates sulfate reduction |
-
Role in Sulfur Metabolism : These reactions enable sulfite production for cysteine biosynthesis, linking PAP to cellular redox balance .
Enzymatic Inhibition Mechanisms
PAP competitively inhibits 5'→3' exoribonucleases (e.g., DXO, Xrn1) by mimicking RNA termini:
Q & A
Basic Research Questions
Q. Q1. What is the biochemical role of adenosine-3'-5'-diphosphate (PAP) in sulfotransferase-mediated reactions?
A1. PAP serves as the cofactor product in sulfotransferase (SULT) reactions, where it is generated after the transfer of a sulfate group from 3'-phosphoadenosine-5'-phosphosulfate (PAPS) to a substrate. Structural studies of human SULT1B1 and SULT1C1 reveal that PAP remains tightly bound to the enzyme's active site post-reaction, influencing conformational changes and substrate specificity . Methodologically, PAP's presence is critical for crystallographic studies of SULT enzymes, as it stabilizes the enzyme-product complex for structural analysis .
Q. Q2. How is PAP synthesized and quantified in enzymatic assays?
A2. PAP is synthesized via enzymatic degradation of PAPS during sulfation reactions (e.g., in chondroitin sulfation) . Quantification methods include hydrophilic interaction liquid chromatography-mass spectrometry (HILIC-MS), which offers high sensitivity for detecting PAP in cellular fractions. This approach minimizes interference from polar metabolites and enables accurate measurement of PAP levels in Golgi fractions or cell lysates .
Q. Q3. What are the stability considerations for PAP in experimental workflows?
A3. PAP is prone to enzymatic and chemical degradation due to its labile phosphate groups. For stability, storage at ≤–20°C in anhydrous conditions is recommended, with handling under inert atmospheres (e.g., nitrogen) to prevent hydrolysis . Avoid repeated freeze-thaw cycles, and validate purity via ³¹P NMR or HPLC-MS before critical experiments .
Advanced Research Questions
Q. Q4. How do structural studies leverage PAP to elucidate sulfotransferase mechanisms?
A4. Co-crystallization of SULT enzymes with PAP provides insights into active-site geometry and catalytic residues. For example, in SULT2B1b, PAP binding induces a conformational shift that positions the substrate-binding loop for optimal sulfation . Advanced techniques include soaking pre-formed enzyme crystals with PAP or using non-hydrolysable PAP analogues (e.g., adenosine-3',5'-bisdiphosphate) to overcome degradation during X-ray diffraction experiments .
Q. Q5. What experimental strategies address PAP's interference in coupled enzyme assays?
A5. PAP can inhibit sulfotransferases via product feedback. To mitigate this, researchers employ:
- Dialysis or size-exclusion chromatography to remove PAP post-reaction.
- Coupled enzyme systems (e.g., pyrophosphatase) to hydrolyze PAP into non-inhibitory adenosine-3'-phosphate (3'-AMP) .
- Isocratic paired-ion reverse-phase HPLC to monitor PAP formation in real time, enabling kinetic analysis without assay interruption .
Q. Q6. How does PAP function as a toxic effector in bacterial systems?
A6. Adenosine-3',5'-bisdiphosphate (ppApp), a structural analogue of PAP, inhibits bacterial growth by disrupting nucleotide metabolism. ppApp is synthesized by RelA-SpoT Homologue (RSH) enzymes and competitively binds to GTPase domains, stalling translation and replication. Methodologically, non-hydrolysable ppApp analogues (e.g., methylene bisphosphonate derivatives) are used to study RSH enzyme kinetics without degradation artifacts .
Q. Methodological Challenges and Solutions
Q. Q7. What are the limitations of PAP quantification in heterogeneous biological samples?
A7. Challenges include:
- Matrix effects from polar metabolites in cell lysates, which obscure PAP detection.
- Solution: HILIC-MS with isotopically labeled PAP (e.g., ¹³C/¹⁵N-PAP) as an internal standard improves specificity .
- Low abundance in subcellular compartments (e.g., Golgi).
- Solution: Immunoprecipitation of PAP-bound enzymes (e.g., SULTs) followed by acid extraction enhances recovery .
Q. Q8. How can researchers validate PAP's role in dynamic sulfation pathways?
A8. Use radiolabeled PAPS (³⁵S-PAPS) in in vitro assays to track sulfate transfer and PAP generation via autoradiography. Alternatively, ³¹P NMR monitors phosphate group dynamics in PAP during enzymatic turnover, providing real-time metabolic flux data .
Q. Data Contradictions and Interpretations
Q. Q9. Why do discrepancies exist in reported PAP binding affinities across SULT isoforms?
A9. Variations arise from:
- Crystallographic conditions (e.g., pH, ionic strength) altering PAP-enzyme interactions.
- Post-translational modifications (e.g., phosphorylation) in native vs. recombinant enzymes .
- Resolution: Compare binding constants (Kd) using surface plasmon resonance (SPR) under standardized buffer conditions.
Q. Q10. How do non-canonical PAP derivatives (e.g., ppApp) impact mechanistic models?
A10. ppApp's toxicity in bacteria challenges the assumption that PAP analogues are inert. Studies using molecular docking and cryo-EM reveal that ppApp occupies GTP-binding pockets in ribosomes, suggesting evolutionary divergence in nucleotide effector mechanisms .
Vergleich Mit ähnlichen Verbindungen
PAP vs. Adenosine-3'-Phosphate-5'-Phosphosulfate (PAPS)
- Structural Difference : PAPS contains a sulfate group esterified to the 5'-phosphate, whereas PAP lacks this modification.
- Functional Roles: PAPS: Direct sulfate donor for SULT-mediated sulfation of substrates (e.g., hydroxysteroids, neurotransmitters). Its synthesis involves ATP sulfurylase and APS kinase, forming a high-energy sulfonate bond . PAP: Product inhibitor of SULTs; its accumulation necessitates enzymatic recycling (e.g., via PAP phosphatase) to regenerate adenosine monophosphate (AMP) and inorganic phosphate .
- Binding Specificity : Luminescent europium complexes distinguish PAPS from PAP due to differential turn-on intensities, despite their structural similarity .
PAP vs. Adenosine-5'-Diphosphate (ADP)
- Structural Difference : ADP has consecutive 5' phosphates, while PAP’s phosphates are at 3' and 5' positions.
- Functional Roles: ADP: Central to energy metabolism (e.g., ATP synthesis) and nucleotide biosynthesis.
- Enzyme Interactions : ADP reductase activity in Ehrlich tumor cells is Mg²⁺-sensitive and inhibited by organic solvents, unlike PAP-dependent SULT inhibition .
PAP vs. Adenosine-5'-Triphosphate (ATP)
- Structural Difference: ATP has three 5' phosphates vs. PAP’s two non-consecutive phosphates.
- Functional Roles: ATP: Primary energy currency; phosphorylates substrates in kinase reactions. PAP: No phosphorylating activity; instead, modulates SULT active sites.
- Thermodynamic Stability : ATP’s γ-phosphate provides high-energy bonds for catalysis, while PAP’s 3'-phosphate enhances binding affinity to SULTs .
PAP vs. Adenosine-2',5'-Diphosphate (2',5'-ADP)
- Structural Difference : 2',5'-ADP has phosphates at 2' and 5' positions.
- Functional Impact: In eosinophil-derived neurotoxin (EDN), 3',5'-ADP (PAP) forms specific hydrogen bonds (e.g., His129, Thr44) that 2',5'-ADP cannot replicate, highlighting positional sensitivity in enzyme inhibition .
Key Research Findings and Data Tables
Table 1: Structural and Functional Comparison of PAP and Analogues
Table 2: Enzymatic Recycling Pathways Involving PAP
Mechanistic Insights from Structural Studies
- SULT1B1/PAP Complex : Crystal structures reveal PAP’s 3'-phosphate forms hydrogen bonds with Arg144 and Ser143, stabilizing its position in the active site. This interaction is absent in ADP-bound structures .
- HS2ST Inhibition : PAP competes with PAPS in heparan sulfate 2-O-sulfotransferase (HS2ST), occupying the cofactor-binding pocket and preventing sulfate transfer .
- EDN Inhibition : PAP’s 3',5'-diphosphate geometry matches EDN’s catalytic cleft, achieving sub-micromolar inhibition (Ki = 0.2 µM), whereas 5'-ADP is 100-fold less potent .
Eigenschaften
IUPAC Name |
[(2R,3S,4R,5R)-5-(6-aminopurin-9-yl)-4-hydroxy-2-(phosphonooxymethyl)oxolan-3-yl] dihydrogen phosphate | |
---|---|---|
Source | PubChem | |
URL | https://pubchem.ncbi.nlm.nih.gov | |
Description | Data deposited in or computed by PubChem | |
InChI |
InChI=1S/C10H15N5O10P2/c11-8-5-9(13-2-12-8)15(3-14-5)10-6(16)7(25-27(20,21)22)4(24-10)1-23-26(17,18)19/h2-4,6-7,10,16H,1H2,(H2,11,12,13)(H2,17,18,19)(H2,20,21,22)/t4-,6-,7-,10-/m1/s1 | |
Source | PubChem | |
URL | https://pubchem.ncbi.nlm.nih.gov | |
Description | Data deposited in or computed by PubChem | |
InChI Key |
WHTCPDAXWFLDIH-KQYNXXCUSA-N | |
Source | PubChem | |
URL | https://pubchem.ncbi.nlm.nih.gov | |
Description | Data deposited in or computed by PubChem | |
Canonical SMILES |
C1=NC(=C2C(=N1)N(C=N2)C3C(C(C(O3)COP(=O)(O)O)OP(=O)(O)O)O)N | |
Source | PubChem | |
URL | https://pubchem.ncbi.nlm.nih.gov | |
Description | Data deposited in or computed by PubChem | |
Isomeric SMILES |
C1=NC(=C2C(=N1)N(C=N2)[C@H]3[C@@H]([C@@H]([C@H](O3)COP(=O)(O)O)OP(=O)(O)O)O)N | |
Source | PubChem | |
URL | https://pubchem.ncbi.nlm.nih.gov | |
Description | Data deposited in or computed by PubChem | |
Molecular Formula |
C10H15N5O10P2 | |
Source | PubChem | |
URL | https://pubchem.ncbi.nlm.nih.gov | |
Description | Data deposited in or computed by PubChem | |
Related CAS |
75431-54-8 (di-hydrochloride salt) | |
Record name | Adenosine 3'-phosphate-5'-phosphate | |
Source | ChemIDplus | |
URL | https://pubchem.ncbi.nlm.nih.gov/substance/?source=chemidplus&sourceid=0001053732 | |
Description | ChemIDplus is a free, web search system that provides access to the structure and nomenclature authority files used for the identification of chemical substances cited in National Library of Medicine (NLM) databases, including the TOXNET system. | |
DSSTOX Substance ID |
DTXSID50909413 | |
Record name | Adenosine 3'-phosphate-5'-phosphate | |
Source | EPA DSSTox | |
URL | https://comptox.epa.gov/dashboard/DTXSID50909413 | |
Description | DSSTox provides a high quality public chemistry resource for supporting improved predictive toxicology. | |
Molecular Weight |
427.20 g/mol | |
Source | PubChem | |
URL | https://pubchem.ncbi.nlm.nih.gov | |
Description | Data deposited in or computed by PubChem | |
Physical Description |
Solid | |
Record name | Adenosine 3',5'-diphosphate | |
Source | Human Metabolome Database (HMDB) | |
URL | http://www.hmdb.ca/metabolites/HMDB0000061 | |
Description | The Human Metabolome Database (HMDB) is a freely available electronic database containing detailed information about small molecule metabolites found in the human body. | |
Explanation | HMDB is offered to the public as a freely available resource. Use and re-distribution of the data, in whole or in part, for commercial purposes requires explicit permission of the authors and explicit acknowledgment of the source material (HMDB) and the original publication (see the HMDB citing page). We ask that users who download significant portions of the database cite the HMDB paper in any resulting publications. | |
Solubility |
614.5 mg/mL | |
Record name | Adenosine 3',5'-diphosphate | |
Source | Human Metabolome Database (HMDB) | |
URL | http://www.hmdb.ca/metabolites/HMDB0000061 | |
Description | The Human Metabolome Database (HMDB) is a freely available electronic database containing detailed information about small molecule metabolites found in the human body. | |
Explanation | HMDB is offered to the public as a freely available resource. Use and re-distribution of the data, in whole or in part, for commercial purposes requires explicit permission of the authors and explicit acknowledgment of the source material (HMDB) and the original publication (see the HMDB citing page). We ask that users who download significant portions of the database cite the HMDB paper in any resulting publications. | |
CAS No. |
1053-73-2 | |
Record name | Adenosine 3′,5′-diphosphate | |
Source | CAS Common Chemistry | |
URL | https://commonchemistry.cas.org/detail?cas_rn=1053-73-2 | |
Description | CAS Common Chemistry is an open community resource for accessing chemical information. Nearly 500,000 chemical substances from CAS REGISTRY cover areas of community interest, including common and frequently regulated chemicals, and those relevant to high school and undergraduate chemistry classes. This chemical information, curated by our expert scientists, is provided in alignment with our mission as a division of the American Chemical Society. | |
Explanation | The data from CAS Common Chemistry is provided under a CC-BY-NC 4.0 license, unless otherwise stated. | |
Record name | Adenosine 3'-phosphate-5'-phosphate | |
Source | ChemIDplus | |
URL | https://pubchem.ncbi.nlm.nih.gov/substance/?source=chemidplus&sourceid=0001053732 | |
Description | ChemIDplus is a free, web search system that provides access to the structure and nomenclature authority files used for the identification of chemical substances cited in National Library of Medicine (NLM) databases, including the TOXNET system. | |
Record name | Adenosine 3',5'-diphosphate | |
Source | DrugBank | |
URL | https://www.drugbank.ca/drugs/DB01812 | |
Description | The DrugBank database is a unique bioinformatics and cheminformatics resource that combines detailed drug (i.e. chemical, pharmacological and pharmaceutical) data with comprehensive drug target (i.e. sequence, structure, and pathway) information. | |
Explanation | Creative Common's Attribution-NonCommercial 4.0 International License (http://creativecommons.org/licenses/by-nc/4.0/legalcode) | |
Record name | Adenosine 3'-phosphate-5'-phosphate | |
Source | EPA DSSTox | |
URL | https://comptox.epa.gov/dashboard/DTXSID50909413 | |
Description | DSSTox provides a high quality public chemistry resource for supporting improved predictive toxicology. | |
Record name | ADENOSINE 3',5'-DIPHOSPHATE | |
Source | FDA Global Substance Registration System (GSRS) | |
URL | https://gsrs.ncats.nih.gov/ginas/app/beta/substances/C65F80D52U | |
Description | The FDA Global Substance Registration System (GSRS) enables the efficient and accurate exchange of information on what substances are in regulated products. Instead of relying on names, which vary across regulatory domains, countries, and regions, the GSRS knowledge base makes it possible for substances to be defined by standardized, scientific descriptions. | |
Explanation | Unless otherwise noted, the contents of the FDA website (www.fda.gov), both text and graphics, are not copyrighted. They are in the public domain and may be republished, reprinted and otherwise used freely by anyone without the need to obtain permission from FDA. Credit to the U.S. Food and Drug Administration as the source is appreciated but not required. | |
Record name | Adenosine 3',5'-diphosphate | |
Source | Human Metabolome Database (HMDB) | |
URL | http://www.hmdb.ca/metabolites/HMDB0000061 | |
Description | The Human Metabolome Database (HMDB) is a freely available electronic database containing detailed information about small molecule metabolites found in the human body. | |
Explanation | HMDB is offered to the public as a freely available resource. Use and re-distribution of the data, in whole or in part, for commercial purposes requires explicit permission of the authors and explicit acknowledgment of the source material (HMDB) and the original publication (see the HMDB citing page). We ask that users who download significant portions of the database cite the HMDB paper in any resulting publications. | |
Retrosynthesis Analysis
AI-Powered Synthesis Planning: Our tool employs the Template_relevance Pistachio, Template_relevance Bkms_metabolic, Template_relevance Pistachio_ringbreaker, Template_relevance Reaxys, Template_relevance Reaxys_biocatalysis model, leveraging a vast database of chemical reactions to predict feasible synthetic routes.
One-Step Synthesis Focus: Specifically designed for one-step synthesis, it provides concise and direct routes for your target compounds, streamlining the synthesis process.
Accurate Predictions: Utilizing the extensive PISTACHIO, BKMS_METABOLIC, PISTACHIO_RINGBREAKER, REAXYS, REAXYS_BIOCATALYSIS database, our tool offers high-accuracy predictions, reflecting the latest in chemical research and data.
Strategy Settings
Precursor scoring | Relevance Heuristic |
---|---|
Min. plausibility | 0.01 |
Model | Template_relevance |
Template Set | Pistachio/Bkms_metabolic/Pistachio_ringbreaker/Reaxys/Reaxys_biocatalysis |
Top-N result to add to graph | 6 |
Feasible Synthetic Routes
Haftungsausschluss und Informationen zu In-Vitro-Forschungsprodukten
Bitte beachten Sie, dass alle Artikel und Produktinformationen, die auf BenchChem präsentiert werden, ausschließlich zu Informationszwecken bestimmt sind. Die auf BenchChem zum Kauf angebotenen Produkte sind speziell für In-vitro-Studien konzipiert, die außerhalb lebender Organismen durchgeführt werden. In-vitro-Studien, abgeleitet von dem lateinischen Begriff "in Glas", beinhalten Experimente, die in kontrollierten Laborumgebungen unter Verwendung von Zellen oder Geweben durchgeführt werden. Es ist wichtig zu beachten, dass diese Produkte nicht als Arzneimittel oder Medikamente eingestuft sind und keine Zulassung der FDA für die Vorbeugung, Behandlung oder Heilung von medizinischen Zuständen, Beschwerden oder Krankheiten erhalten haben. Wir müssen betonen, dass jede Form der körperlichen Einführung dieser Produkte in Menschen oder Tiere gesetzlich strikt untersagt ist. Es ist unerlässlich, sich an diese Richtlinien zu halten, um die Einhaltung rechtlicher und ethischer Standards in Forschung und Experiment zu gewährleisten.