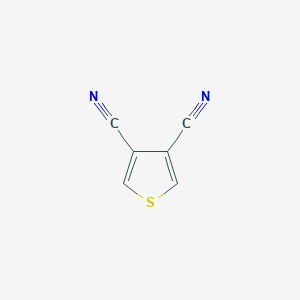
3,4-Dicyanothiophene
Übersicht
Beschreibung
3,4-Dicyanothiophene is an organic compound with the molecular formula C6H2N2S. It is a derivative of thiophene, a five-membered aromatic ring containing sulfur. The compound is characterized by the presence of two cyano groups (-CN) attached to the 3 and 4 positions of the thiophene ring. This structural modification imparts unique electronic properties to the molecule, making it a valuable building block in various scientific and industrial applications.
Vorbereitungsmethoden
Synthetic Routes and Reaction Conditions: The synthesis of 3,4-dicyanothiophene typically involves the reaction of thiophene with cyanogen bromide (BrCN) in the presence of a base such as sodium hydroxide (NaOH). The reaction proceeds through the formation of an intermediate, which subsequently undergoes cyclization to yield this compound. The reaction conditions often include a solvent such as acetonitrile (CH3CN) and a temperature range of 50-70°C.
Industrial Production Methods: In an industrial setting, the production of this compound can be scaled up by optimizing the reaction parameters, such as the concentration of reactants, reaction time, and temperature. Continuous flow reactors may be employed to enhance the efficiency and yield of the synthesis process. The purification of the final product is typically achieved through recrystallization or chromatography techniques.
Analyse Chemischer Reaktionen
Types of Reactions: 3,4-Dicyanothiophene undergoes various chemical reactions, including:
Oxidation: The compound can be oxidized using oxidizing agents such as potassium permanganate (KMnO4) or hydrogen peroxide (H2O2) to form corresponding sulfoxides or sulfones.
Reduction: Reduction of this compound can be achieved using reducing agents like lithium aluminum hydride (LiAlH4) or sodium borohydride (NaBH4), leading to the formation of amines.
Substitution: The cyano groups in this compound can undergo nucleophilic substitution reactions with nucleophiles such as amines or alcohols, resulting in the formation of substituted derivatives.
Common Reagents and Conditions:
Oxidation: Potassium permanganate (KMnO4) in aqueous or acidic medium.
Reduction: Lithium aluminum hydride (LiAlH4) in anhydrous ether.
Substitution: Amines or alcohols in the presence of a base such as sodium hydroxide (NaOH).
Major Products:
Oxidation: Sulfoxides or sulfones.
Reduction: Amines.
Substitution: Substituted thiophene derivatives.
Wissenschaftliche Forschungsanwendungen
Polymer Solar Cells
Overview
3,4-Dicyanothiophene is primarily recognized for its application in polymer solar cells (PSCs). It serves as a versatile building block for constructing conjugated polymers that exhibit high efficiency and low production costs.
Performance Metrics
Research has shown that polymers derived from DCT can achieve impressive power conversion efficiencies (PCEs). For example, the polymer PB3TCN-C66, which incorporates DCT, demonstrated a PCE of 11.2% when paired with the electron acceptor IT-4F. In contrast, its cyano-free analogue PB3T-C66 achieved only 2.3% PCE, highlighting the advantages of incorporating cyano groups into the polymer structure .
Table 1: Performance Comparison of DCT-Based Polymers
Polymer | Electron Acceptor | Power Conversion Efficiency (%) |
---|---|---|
PB3TCN-C66 | IT-4F | 11.2 |
PB3T-C66 | IT-4F | 2.3 |
Other DCT Polymers | Various | Up to 15.7 |
Organic Semiconductors
Properties and Benefits
DCT's electron-deficient nature allows it to form high-performance organic semiconductors. The conjugated structure facilitates efficient charge transport, making it suitable for various electronic applications.
Case Studies
- Ternary Blended Systems : A study on ternary blended PSCs utilizing DCT-based polymers achieved a remarkable efficiency of 15.7% when combined with Y6-BO as an electron acceptor. This efficiency is competitive with traditional fullerene-based systems .
- Halogen-Free Polymers : Research has demonstrated that halogen-free polymer donors based on DCT can also yield high efficiencies in PSCs, further emphasizing its versatility as a building block in sustainable energy solutions .
Other Optoelectronic Applications
In addition to solar cells, DCT is being explored for its potential in other optoelectronic applications:
- Light Emitting Diodes (LEDs) : The incorporation of DCT into polymer matrices can enhance the light-emitting properties of organic LEDs.
- Field Effect Transistors (FETs) : DCT-based materials are being investigated for use in organic FETs due to their favorable charge transport characteristics.
Synthesis and Characterization
Synthesis Methods
The synthesis of this compound typically involves reactions between thiophene derivatives and cyanogen bromide or similar agents. The yield from these reactions can range from 60% to 70%, depending on specific conditions.
Characterization Techniques
Characterization of DCT and its derivatives employs various techniques:
- Nuclear Magnetic Resonance (NMR) : Used to confirm the molecular structure.
- Infrared (IR) Spectroscopy : Helps identify functional groups and verify the presence of cyano groups.
Wirkmechanismus
The mechanism of action of 3,4-dicyanothiophene is primarily attributed to its electron-withdrawing cyano groups, which modulate the electronic properties of the thiophene ring. This modulation enhances the compound’s ability to participate in various chemical reactions, such as electron transfer processes. The cyano groups also influence the compound’s interaction with molecular targets, such as enzymes or receptors, by altering its binding affinity and specificity.
Vergleich Mit ähnlichen Verbindungen
3,4-Dicyanothiophene can be compared with other thiophene derivatives, such as:
2,5-Dicyanothiophene: Similar to this compound but with cyano groups at the 2 and 5 positions.
3,4-Dibromothiophene: Contains bromine atoms instead of cyano groups at the 3 and 4 positions.
3,4-Dimethylthiophene: Contains methyl groups at the 3 and 4 positions.
Uniqueness: The presence of cyano groups in this compound imparts strong electron-withdrawing properties, making it distinct from other thiophene derivatives. This unique electronic characteristic enhances its utility in the synthesis of high-performance materials and its application in various scientific fields.
Biologische Aktivität
3,4-Dicyanothiophene (DCT) is a sulfur-containing heterocyclic compound that has garnered attention for its potential applications in various fields, particularly in organic electronics and medicinal chemistry. This article provides a comprehensive overview of the biological activity of DCT, including its synthesis, structural properties, and relevant research findings.
Chemical Structure and Properties
This compound is characterized by two cyano groups attached to a thiophene ring. The presence of these electron-withdrawing cyano groups significantly influences its electronic properties, making it a valuable building block for constructing conjugated polymers used in organic solar cells (OSCs) and other electronic applications.
Synthesis of this compound
DCT can be synthesized through various methods, including:
- Cyclization Reactions : Utilizing thiophene derivatives and carbonyl compounds.
- Nucleophilic Substitution : Involving reactions with isocyanates or thioisocyanates in the presence of bases.
These synthetic routes allow for the introduction of diverse functional groups, enhancing the compound's versatility for further modifications.
Antimicrobial Properties
Research has indicated that thiophene derivatives exhibit significant antimicrobial activity. DCT has been evaluated for its potential as an antibacterial and antifungal agent. A study demonstrated that certain derivatives of thiophene, including DCT, showed promising activity against various bacterial strains and fungi, suggesting its utility in developing new antimicrobial agents .
Anticancer Activity
The anticancer potential of DCT has also been explored. Compounds derived from thiophene scaffolds have shown efficacy against different cancer cell lines. For instance, studies have reported that thiophene-based compounds can induce apoptosis in cancer cells through various mechanisms, including the inhibition of specific signaling pathways involved in cell proliferation .
Case Studies
- Antimicrobial Efficacy :
- Anticancer Research :
Research Findings
Recent investigations into DCT have focused on its role in organic electronics as well as its biological activities:
- Organic Solar Cells : DCT has been incorporated into polymer blends to enhance photovoltaic performance. Ternary copolymers containing DCT have shown improved efficiency due to better charge transport properties and reduced energy losses during operation .
- Structure-Activity Relationship (SAR) : The biological activity of DCT derivatives is influenced by their structural modifications. Variations in substituents can enhance or diminish their antimicrobial and anticancer properties, emphasizing the importance of SAR studies in drug design .
Q & A
Basic Research Questions
Q. What are the key synthetic methodologies for incorporating 3,4-dicyanothiophene (DCT) into conjugated polymers, and how do these methods influence polymer properties?
- Methodology : DCT is typically integrated into polymer backbones via Stille or Suzuki coupling reactions due to its electron-deficient nature, which enhances charge transport. For example, ternary copolymerization of DCT with electron-rich monomers (e.g., benzodithiophene derivatives) and halogen-free comonomers optimizes energy level alignment and film morphology. Researchers use nuclear magnetic resonance (NMR) and gel permeation chromatography (GPC) to confirm structural integrity and molecular weight distribution .
- Impact : Adjusting the DCT content (e.g., 25–75 mol%) in copolymers like PBCNT-series polymers improves power conversion efficiency (PCE) in organic solar cells (OSCs) by reducing energy loss and enhancing open-circuit voltage (VOC) .
Q. How does DCT contribute to reducing non-radiative recombination energy loss in organic solar cells?
- Mechanism : The strong electron-withdrawing cyano groups in DCT lower the highest occupied molecular orbital (HOMO) energy levels of donor polymers, minimizing the energy offset between donor and acceptor materials. This reduces charge recombination and non-radiative energy losses (ΔEnr), as demonstrated in DCT-based terpolymers achieving ΔEnr as low as 0.22 eV .
- Validation : Transient absorption spectroscopy and electroluminescence quantum efficiency (EQEEL) measurements quantify energy loss reduction, correlating with PCE improvements up to 16.6% in Y6-BO-based OSCs .
Q. What spectroscopic techniques are critical for characterizing DCT-based polymers?
- Techniques : Ultraviolet-visible (UV-Vis) spectroscopy identifies optical bandgaps, while cyclic voltammetry (CV) determines HOMO/lowest unoccupied molecular orbital (LUMO) levels. Grazing-incidence wide-angle X-ray scattering (GIWAXS) analyzes molecular packing and crystallinity, which are crucial for charge transport .
- Case Study : In PBCNT75:Y6-BO blends, GIWAXS revealed preferential face-on orientation, enabling high hole mobility (>10<sup>−3</sup> cm<sup>2</sup> V<sup>−1</sup> s<sup>−1</sup>) and reduced bimolecular recombination .
Advanced Research Questions
Q. How can researchers resolve contradictions in reported PCE values for DCT-based polymers across different acceptor systems?
- Analysis Framework :
Material Purity : Verify monomer purity via high-performance liquid chromatography (HPLC) to eliminate batch-to-batch variability.
Morphology Optimization : Use solvent additives (e.g., 1,8-diiodooctane) or thermal annealing to refine phase separation.
Device Architecture : Compare inverted vs. conventional structures to isolate interfacial effects. For instance, DCT polymers paired with PC71BM acceptors showed lower PCE (10–12%) than Y6-BO (15–16.6%) due to reduced miscibility .
- Data Reconciliation : Cross-validate external quantum efficiency (EQE) and current density-voltage (J-V) curves under standardized AM1.5G illumination to minimize measurement discrepancies .
Q. What strategies optimize the ternary copolymer design of DCT-based polymers for balanced charge transport and mechanical stability?
- Design Principles :
- Comonomer Selection : Incorporate flexible alkyl side chains (e.g., 2-ethylhexyl) to enhance mechanical durability without sacrificing charge mobility.
- Random vs. Block Copolymerization : Random terpolymers (e.g., PBCNT75) achieve better phase separation than block copolymers, as shown by atomic force microscopy (AFM) and tensile testing .
Q. How do solvent choice and processing conditions affect the morphology and efficiency of DCT-based active layers?
- Solvent Engineering : High-boiling-point solvents (e.g., chlorobenzene) promote slower crystallization, yielding larger donor-acceptor domains. Conversely, low-boiling-point solvents (e.g., chloroform) result in finer phase separation but increased defect density .
- Process Optimization : Blade-coating vs. spin-coating impacts film uniformity. Blade-coated DCT:Y6-BO films showed 5% higher PCE due to improved molecular alignment, validated by polarized optical microscopy .
Q. Contradictions and Emerging Challenges
Q. Why do some studies report conflicting trends between DCT content and device stability?
- Hypothesis : Excessive DCT (>75 mol%) induces backbone rigidity, leading to brittle films and delamination under thermal stress. However, moderate DCT (50 mol%) balances mechanical and electronic properties, as evidenced by accelerated aging tests (85°C/85% RH for 500 hours) showing <10% PCE degradation .
- Resolution : Use cross-linkable comonomers (e.g., vinyl groups) or interfacial layers (e.g., ZnO nanoparticles) to enhance stability without compromising efficiency .
Eigenschaften
IUPAC Name |
thiophene-3,4-dicarbonitrile | |
---|---|---|
Source | PubChem | |
URL | https://pubchem.ncbi.nlm.nih.gov | |
Description | Data deposited in or computed by PubChem | |
InChI |
InChI=1S/C6H2N2S/c7-1-5-3-9-4-6(5)2-8/h3-4H | |
Source | PubChem | |
URL | https://pubchem.ncbi.nlm.nih.gov | |
Description | Data deposited in or computed by PubChem | |
InChI Key |
JSLBLMYJYPZTEB-UHFFFAOYSA-N | |
Source | PubChem | |
URL | https://pubchem.ncbi.nlm.nih.gov | |
Description | Data deposited in or computed by PubChem | |
Canonical SMILES |
C1=C(C(=CS1)C#N)C#N | |
Source | PubChem | |
URL | https://pubchem.ncbi.nlm.nih.gov | |
Description | Data deposited in or computed by PubChem | |
Molecular Formula |
C6H2N2S | |
Source | PubChem | |
URL | https://pubchem.ncbi.nlm.nih.gov | |
Description | Data deposited in or computed by PubChem | |
DSSTOX Substance ID |
DTXSID20172213 | |
Record name | 3,4-Dicyanothiophene | |
Source | EPA DSSTox | |
URL | https://comptox.epa.gov/dashboard/DTXSID20172213 | |
Description | DSSTox provides a high quality public chemistry resource for supporting improved predictive toxicology. | |
Molecular Weight |
134.16 g/mol | |
Source | PubChem | |
URL | https://pubchem.ncbi.nlm.nih.gov | |
Description | Data deposited in or computed by PubChem | |
CAS No. |
18853-32-2 | |
Record name | 3,4-Dicyanothiophene | |
Source | ChemIDplus | |
URL | https://pubchem.ncbi.nlm.nih.gov/substance/?source=chemidplus&sourceid=0018853322 | |
Description | ChemIDplus is a free, web search system that provides access to the structure and nomenclature authority files used for the identification of chemical substances cited in National Library of Medicine (NLM) databases, including the TOXNET system. | |
Record name | 3,4-Thiophenedicarbonitrile | |
Source | DTP/NCI | |
URL | https://dtp.cancer.gov/dtpstandard/servlet/dwindex?searchtype=NSC&outputformat=html&searchlist=149716 | |
Description | The NCI Development Therapeutics Program (DTP) provides services and resources to the academic and private-sector research communities worldwide to facilitate the discovery and development of new cancer therapeutic agents. | |
Explanation | Unless otherwise indicated, all text within NCI products is free of copyright and may be reused without our permission. Credit the National Cancer Institute as the source. | |
Record name | 3,4-Dicyanothiophene | |
Source | EPA DSSTox | |
URL | https://comptox.epa.gov/dashboard/DTXSID20172213 | |
Description | DSSTox provides a high quality public chemistry resource for supporting improved predictive toxicology. | |
Synthesis routes and methods
Procedure details
Haftungsausschluss und Informationen zu In-Vitro-Forschungsprodukten
Bitte beachten Sie, dass alle Artikel und Produktinformationen, die auf BenchChem präsentiert werden, ausschließlich zu Informationszwecken bestimmt sind. Die auf BenchChem zum Kauf angebotenen Produkte sind speziell für In-vitro-Studien konzipiert, die außerhalb lebender Organismen durchgeführt werden. In-vitro-Studien, abgeleitet von dem lateinischen Begriff "in Glas", beinhalten Experimente, die in kontrollierten Laborumgebungen unter Verwendung von Zellen oder Geweben durchgeführt werden. Es ist wichtig zu beachten, dass diese Produkte nicht als Arzneimittel oder Medikamente eingestuft sind und keine Zulassung der FDA für die Vorbeugung, Behandlung oder Heilung von medizinischen Zuständen, Beschwerden oder Krankheiten erhalten haben. Wir müssen betonen, dass jede Form der körperlichen Einführung dieser Produkte in Menschen oder Tiere gesetzlich strikt untersagt ist. Es ist unerlässlich, sich an diese Richtlinien zu halten, um die Einhaltung rechtlicher und ethischer Standards in Forschung und Experiment zu gewährleisten.