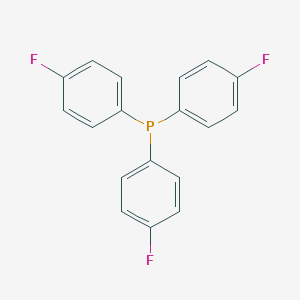
Tris(4-Fluorphenyl)phosphin
Übersicht
Beschreibung
Tris(4-fluorophenyl)phosphine is an organophosphorus compound with the chemical formula C18H12F3P. It is a phosphine derivative where three fluorophenyl groups are attached to a central phosphorus atom. This compound is known for its applications in organic synthesis, pharmaceuticals, agrochemicals, and dyestuff industries .
Wissenschaftliche Forschungsanwendungen
Tris(4-fluorophenyl)phosphine has a wide range of scientific research applications, including:
Biology: It is employed in the synthesis of biologically active compounds and pharmaceuticals.
Medicine: It plays a role in the development of new drugs and therapeutic agents.
Wirkmechanismus
Target of Action
Tris(4-fluorophenyl)phosphine is a ligand that primarily targets rhodium . Rhodium is a chemical element often used in catalysis, a process that speeds up chemical reactions. The compound’s interaction with rhodium plays a crucial role in various chemical reactions .
Mode of Action
The compound acts as a ligand for rhodium-catalyzed oxygenative addition to terminal alkynes . This means that it binds to rhodium and facilitates the addition of oxygen to alkynes, a type of organic compound. This interaction results in the synthesis of esters, amides, and carboxylic acids .
Biochemical Pathways
Its role as a ligand in rhodium-catalyzed reactions suggests that it may influence pathways involving the synthesis of esters, amides, and carboxylic acids .
Result of Action
The primary result of Tris(4-fluorophenyl)phosphine’s action is the synthesis of esters, amides, and carboxylic acids . These compounds have various applications in organic chemistry and biochemistry, including the production of pharmaceuticals, polymers, and more.
Action Environment
Tris(4-fluorophenyl)phosphine is sensitive to air . It should be stored in a cool place, with the container kept tightly closed in a dry and well-ventilated place . It should also be kept away from strong oxidizing agents . These environmental factors can influence the compound’s action, efficacy, and stability.
Vorbereitungsmethoden
Synthetic Routes and Reaction Conditions: Tris(4-fluorophenyl)phosphine can be synthesized by reacting fluorobenzene with phosphorus trichloride under appropriate reaction conditions. The specific steps involve the reaction of fluorobenzene with phosphorus trichloride to obtain the desired product .
Industrial Production Methods: The industrial production of tris(4-fluorophenyl)phosphine follows similar synthetic routes but on a larger scale. The reaction conditions are optimized to ensure high yield and purity of the product. The process involves careful control of temperature, pressure, and reaction time to achieve the desired outcome.
Analyse Chemischer Reaktionen
Types of Reactions: Tris(4-fluorophenyl)phosphine undergoes various types of reactions, including:
Substitution Reactions: It can participate in substitution reactions where the fluorophenyl groups can be replaced by other substituents.
Coupling Reactions: It is commonly used as a ligand in coupling reactions such as Buchwald-Hartwig cross-coupling, Heck reaction, Hiyama coupling, Negishi coupling, Sonogashira coupling, Stille coupling, and Suzuki-Miyaura coupling
Common Reagents and Conditions:
Buchwald-Hartwig Cross Coupling Reaction: Typically involves palladium catalysts and bases like potassium tert-butoxide.
Heck Reaction: Utilizes palladium catalysts and bases such as triethylamine.
Suzuki-Miyaura Coupling: Employs palladium catalysts and bases like potassium carbonate.
Major Products: The major products formed from these reactions depend on the specific reagents and conditions used. For example, in Suzuki-Miyaura coupling, the product is typically a biaryl compound.
Vergleich Mit ähnlichen Verbindungen
- Tris(pentafluorophenyl)phosphine
- Tris(4-trifluoromethylphenyl)phosphine
- Tris(4-methoxyphenyl)phosphine
- Tri(o-tolyl)phosphine
- Tri(p-tolyl)phosphine
- Triphenylphosphine
- Tris(2,4,6-trimethylphenyl)phosphine
- Tris(4-chlorophenyl)phosphine
Comparison: Tris(4-fluorophenyl)phosphine is unique due to the presence of fluorine atoms in the phenyl groups, which can influence the electronic properties and reactivity of the compound. Compared to other similar compounds, it may offer different selectivity and efficiency in catalytic reactions, making it a valuable ligand in various chemical processes.
Biologische Aktivität
Tris(4-fluorophenyl)phosphine (TFPP), with the chemical formula and CAS number 18437-78-0, has garnered attention in various fields of research due to its unique chemical properties and potential biological applications. This article explores the biological activity of TFPP, including its synthesis, applications, and relevant case studies.
Molecular Characteristics:
- Molecular Weight: 316.26 g/mol
- Melting Point: 79-83 °C
- Boiling Point: 351.5 °C
- Density: Not available
- Flash Point: 166.4 °C
- Solubility: Highly soluble in organic solvents
Structural Formula:
The structure of TFPP features a phosphorus atom bonded to three para-fluorophenyl groups, which influences its reactivity and interaction with biological systems.
Biological Applications
-
Biochemical Reagent:
TFPP is primarily utilized as a biochemical reagent in organic synthesis and life sciences research. Its role as a phosphine ligand in catalysis has been extensively studied, particularly in transition metal complexation, which is crucial for various biochemical reactions . -
Catalytic Activity:
Research indicates that TFPP can act as an internal standard in quantitative NMR spectroscopy, facilitating the monitoring of reactions involving halophosphines. This application is significant in studies involving the activation and transformation of phosphines into more complex structures . -
Pharmaceutical Chemistry:
The compound has been investigated for its potential as a building block in the synthesis of biologically active molecules. Its ability to stabilize transition states in catalytic cycles makes it a candidate for drug development processes .
Case Study 1: Photoredox Catalysis
A study highlighted the use of TFPP in photoredox catalysis, where it was employed to monitor reaction progress via NMR spectroscopy. The results showed that TFPP effectively facilitated the conversion of various halophosphines under light irradiation, demonstrating its utility in synthetic organic chemistry .
Table 1: Reaction Yields Using TFPP as Internal Standard
Reaction Type | Yield (%) | Notes |
---|---|---|
Photoredox Conversion | 65 | Initial yield after 45 minutes |
Secondary Phosphine Formation | 74 | Final yield after 24 hours |
Diphosphine Production | Variable | Dependent on substituent bulkiness |
Case Study 2: Synthesis of Luminescent Complexes
TFPP has also been incorporated into luminescent copper(I) complexes, showing promising photophysical properties. These complexes are being explored for applications in light-emitting devices and sensors due to their high stability and efficiency .
Safety and Handling
TFPP is classified with a warning signal due to its potential hazards upon exposure:
- Hazard Statements: H315 (causes skin irritation), H319 (causes serious eye irritation), H335 (may cause respiratory irritation).
- Precautionary Measures: Use personal protective equipment (PPE) such as gloves and goggles when handling this compound.
Eigenschaften
IUPAC Name |
tris(4-fluorophenyl)phosphane | |
---|---|---|
Source | PubChem | |
URL | https://pubchem.ncbi.nlm.nih.gov | |
Description | Data deposited in or computed by PubChem | |
InChI |
InChI=1S/C18H12F3P/c19-13-1-7-16(8-2-13)22(17-9-3-14(20)4-10-17)18-11-5-15(21)6-12-18/h1-12H | |
Source | PubChem | |
URL | https://pubchem.ncbi.nlm.nih.gov | |
Description | Data deposited in or computed by PubChem | |
InChI Key |
GEPJPYNDFSOARB-UHFFFAOYSA-N | |
Source | PubChem | |
URL | https://pubchem.ncbi.nlm.nih.gov | |
Description | Data deposited in or computed by PubChem | |
Canonical SMILES |
C1=CC(=CC=C1F)P(C2=CC=C(C=C2)F)C3=CC=C(C=C3)F | |
Source | PubChem | |
URL | https://pubchem.ncbi.nlm.nih.gov | |
Description | Data deposited in or computed by PubChem | |
Molecular Formula |
C18H12F3P | |
Source | PubChem | |
URL | https://pubchem.ncbi.nlm.nih.gov | |
Description | Data deposited in or computed by PubChem | |
DSSTOX Substance ID |
DTXSID80171565 | |
Record name | Phosphine, tris(4-fluorophenyl)- | |
Source | EPA DSSTox | |
URL | https://comptox.epa.gov/dashboard/DTXSID80171565 | |
Description | DSSTox provides a high quality public chemistry resource for supporting improved predictive toxicology. | |
Molecular Weight |
316.3 g/mol | |
Source | PubChem | |
URL | https://pubchem.ncbi.nlm.nih.gov | |
Description | Data deposited in or computed by PubChem | |
CAS No. |
18437-78-0 | |
Record name | Phosphine, tris(4-fluorophenyl)- | |
Source | ChemIDplus | |
URL | https://pubchem.ncbi.nlm.nih.gov/substance/?source=chemidplus&sourceid=0018437780 | |
Description | ChemIDplus is a free, web search system that provides access to the structure and nomenclature authority files used for the identification of chemical substances cited in National Library of Medicine (NLM) databases, including the TOXNET system. | |
Record name | Tris(4-fluorophenyl)phosphine | |
Source | DTP/NCI | |
URL | https://dtp.cancer.gov/dtpstandard/servlet/dwindex?searchtype=NSC&outputformat=html&searchlist=158458 | |
Description | The NCI Development Therapeutics Program (DTP) provides services and resources to the academic and private-sector research communities worldwide to facilitate the discovery and development of new cancer therapeutic agents. | |
Explanation | Unless otherwise indicated, all text within NCI products is free of copyright and may be reused without our permission. Credit the National Cancer Institute as the source. | |
Record name | Phosphine, tris(4-fluorophenyl)- | |
Source | EPA DSSTox | |
URL | https://comptox.epa.gov/dashboard/DTXSID80171565 | |
Description | DSSTox provides a high quality public chemistry resource for supporting improved predictive toxicology. | |
Record name | Tris(4-fluorophenyl)phosphine | |
Source | European Chemicals Agency (ECHA) | |
URL | https://echa.europa.eu/information-on-chemicals | |
Description | The European Chemicals Agency (ECHA) is an agency of the European Union which is the driving force among regulatory authorities in implementing the EU's groundbreaking chemicals legislation for the benefit of human health and the environment as well as for innovation and competitiveness. | |
Explanation | Use of the information, documents and data from the ECHA website is subject to the terms and conditions of this Legal Notice, and subject to other binding limitations provided for under applicable law, the information, documents and data made available on the ECHA website may be reproduced, distributed and/or used, totally or in part, for non-commercial purposes provided that ECHA is acknowledged as the source: "Source: European Chemicals Agency, http://echa.europa.eu/". Such acknowledgement must be included in each copy of the material. ECHA permits and encourages organisations and individuals to create links to the ECHA website under the following cumulative conditions: Links can only be made to webpages that provide a link to the Legal Notice page. | |
Retrosynthesis Analysis
AI-Powered Synthesis Planning: Our tool employs the Template_relevance Pistachio, Template_relevance Bkms_metabolic, Template_relevance Pistachio_ringbreaker, Template_relevance Reaxys, Template_relevance Reaxys_biocatalysis model, leveraging a vast database of chemical reactions to predict feasible synthetic routes.
One-Step Synthesis Focus: Specifically designed for one-step synthesis, it provides concise and direct routes for your target compounds, streamlining the synthesis process.
Accurate Predictions: Utilizing the extensive PISTACHIO, BKMS_METABOLIC, PISTACHIO_RINGBREAKER, REAXYS, REAXYS_BIOCATALYSIS database, our tool offers high-accuracy predictions, reflecting the latest in chemical research and data.
Strategy Settings
Precursor scoring | Relevance Heuristic |
---|---|
Min. plausibility | 0.01 |
Model | Template_relevance |
Template Set | Pistachio/Bkms_metabolic/Pistachio_ringbreaker/Reaxys/Reaxys_biocatalysis |
Top-N result to add to graph | 6 |
Feasible Synthetic Routes
Q1: What is Tris(4-fluorophenyl)phosphine and what are its key applications?
A1: Tris(4-fluorophenyl)phosphine, often abbreviated as TFPP, is an organophosphorus compound with a central phosphorus atom bonded to three 4-fluorophenyl groups. It finds extensive use as a ligand in various chemical reactions, particularly in organometallic chemistry and catalysis. Its electron-withdrawing fluorine substituents influence its electronic properties and coordination behavior, making it a valuable tool for tuning catalyst reactivity and selectivity.
Q2: How does the structure of Tris(4-fluorophenyl)phosphine influence its coordination behavior with metals?
A2: The electron-withdrawing nature of the fluorine atoms in the para position of the phenyl rings increases the π-acidity of TFPP compared to its non-fluorinated counterpart, triphenylphosphine (TPP). [] This enhanced π-acidity results in stronger back-bonding interactions with transition metals, impacting the stability and reactivity of the resulting metal complexes. [, ] For instance, in the case of rhodium complexes used in hydroformylation reactions, TFPP leads to higher selectivity towards linear aldehydes at low P/Ph ratios. []
Q3: What is the significance of the "cone angle" in Tris(4-fluorophenyl)phosphine and how does it relate to its applications?
A3: The cone angle is a measure of the steric bulk of a ligand, which is crucial in determining its coordination chemistry and catalytic activity. In the crystal structure of Tris(4-chlorophenyl)phosphine and Tris(4-fluorophenyl)phosphine, the average C—P—C angle for TFPP was found to be 102.5(2)°. [] Calculations on related complexes revealed effective cone angles (θE) ranging from 152° to 168° for TFPP, indicating its significant steric bulk. [, , ] This bulk can influence the accessibility of the metal center to reactants, impacting the selectivity of catalytic reactions.
Q4: Can you elaborate on the use of Tris(4-fluorophenyl)phosphine in palladium-catalyzed cross-coupling reactions?
A4: TFPP has been successfully employed as a ligand in palladium-catalyzed Hiyama cross-coupling reactions. [] These reactions involve the coupling of aryl halides with trialkoxy(aryl)silanes to form biaryl compounds, which are important building blocks in various fields, including pharmaceuticals and materials science. The presence of a small amount of water was found to be beneficial for the reaction.
Q5: How does Tris(4-fluorophenyl)phosphine contribute to the stabilization of chiral lanthanum complex catalysts for enantioselective epoxidation reactions?
A5: Research has demonstrated that Tris(4-fluorophenyl)phosphine oxide, the oxide derivative of TFPP, plays a crucial role in stabilizing chiral lanthanum complex catalysts used for the enantioselective epoxidation of conjugated enones. [] This stabilization leads to highly effective catalyst systems that provide excellent enantioselectivities and yields for valuable chiral epoxy ketone products.
Q6: Are there any studies on the use of Tris(4-fluorophenyl)phosphine in lithium-sulfur batteries?
A6: Yes, recent studies have explored the use of TFPP as an interfacial mediator in the cathodes of lithium-sulfur batteries. [] Theoretical calculations and experimental findings suggest that TFPP exhibits strong binding affinity for polysulfides, influencing the electrochemical processes and promoting the formation of beneficial short-chain clusters at the interface. This, in turn, enhances the battery's performance, particularly in terms of capacity decay rate and cycling stability.
Q7: What is known about the synthesis of Tris(4-fluorophenyl)phosphine and its derivatives?
A7: TFPP can be synthesized through different routes. One common method involves the Grignard reaction of 4-fluorobromobenzene with phosphorus oxychloride, followed by reduction. [] This method allows for the direct synthesis of Tris(4-fluorophenyl) phosphine oxide (TFPPO), a valuable precursor for TFPP and other derivatives. [] Alternative synthetic approaches and modifications to the existing methods are constantly being explored to optimize yield and purity. []
Q8: Has Tris(4-fluorophenyl)phosphine been investigated for its potential in polymer chemistry?
A8: Absolutely. Tris(4-fluorophenyl)phosphine oxide (TFPPO) has been successfully employed as a monomer in the synthesis of hyperbranched poly(arylene ether phosphine oxide)s (HB PAEPOs). [] The electron-withdrawing fluorine atoms activate the molecule for nucleophilic aromatic substitution reactions, enabling the formation of branched polymer structures. The polymerization process can be tuned by adjusting reaction parameters like monomer concentration and addition mode to control the molecular weight and branching structure of the resulting polymers. [, ]
Q9: Are there any documented studies on the luminescent properties of Tris(4-fluorophenyl)phosphine complexes?
A9: Yes, research has shown that certain copper(I) thiocyanate complexes incorporating Tris(4-fluorophenyl)phosphine and pyridine ligands exhibit luminescent properties. [] These complexes display luminescence in the green to blue region when exposed to UV light. The specific coordination environment around the copper center and the nature of the ligands play a crucial role in determining the emission characteristics.
Haftungsausschluss und Informationen zu In-Vitro-Forschungsprodukten
Bitte beachten Sie, dass alle Artikel und Produktinformationen, die auf BenchChem präsentiert werden, ausschließlich zu Informationszwecken bestimmt sind. Die auf BenchChem zum Kauf angebotenen Produkte sind speziell für In-vitro-Studien konzipiert, die außerhalb lebender Organismen durchgeführt werden. In-vitro-Studien, abgeleitet von dem lateinischen Begriff "in Glas", beinhalten Experimente, die in kontrollierten Laborumgebungen unter Verwendung von Zellen oder Geweben durchgeführt werden. Es ist wichtig zu beachten, dass diese Produkte nicht als Arzneimittel oder Medikamente eingestuft sind und keine Zulassung der FDA für die Vorbeugung, Behandlung oder Heilung von medizinischen Zuständen, Beschwerden oder Krankheiten erhalten haben. Wir müssen betonen, dass jede Form der körperlichen Einführung dieser Produkte in Menschen oder Tiere gesetzlich strikt untersagt ist. Es ist unerlässlich, sich an diese Richtlinien zu halten, um die Einhaltung rechtlicher und ethischer Standards in Forschung und Experiment zu gewährleisten.