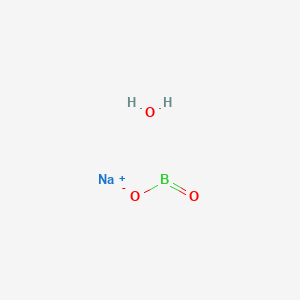
Sodium;oxido(oxo)borane;hydrate
Beschreibung
Sodium;oxido(oxo)borane;hydrate (IUPAC name) is the hydrated sodium salt of metaboric acid, with the general formula NaBO₂·nH₂O. It belongs to the class of inorganic borates, characterized by the presence of the metaborate anion (BO₂⁻) coordinated with a metal cation (Na⁺) and water molecules . Metaborates are typically synthesized via the dehydration of borax (Na₂B₄O₇·10H₂O) or hydrolysis of sodium borohydride (NaBH₄), yielding sodium metaborate as a byproduct .
Eigenschaften
IUPAC Name |
sodium;oxido(oxo)borane;hydrate | |
---|---|---|
Source | PubChem | |
URL | https://pubchem.ncbi.nlm.nih.gov | |
Description | Data deposited in or computed by PubChem | |
InChI |
InChI=1S/BO2.Na.H2O/c2-1-3;;/h;;1H2/q-1;+1; | |
Source | PubChem | |
URL | https://pubchem.ncbi.nlm.nih.gov | |
Description | Data deposited in or computed by PubChem | |
InChI Key |
UYAQHLFAIDLZLE-UHFFFAOYSA-N | |
Source | PubChem | |
URL | https://pubchem.ncbi.nlm.nih.gov | |
Description | Data deposited in or computed by PubChem | |
Canonical SMILES |
B(=O)[O-].O.[Na+] | |
Source | PubChem | |
URL | https://pubchem.ncbi.nlm.nih.gov | |
Description | Data deposited in or computed by PubChem | |
Isomeric SMILES |
B(=O)[O-].O.[Na+] | |
Source | PubChem | |
URL | https://pubchem.ncbi.nlm.nih.gov | |
Description | Data deposited in or computed by PubChem | |
Molecular Formula |
BH2NaO3 | |
Source | PubChem | |
URL | https://pubchem.ncbi.nlm.nih.gov | |
Description | Data deposited in or computed by PubChem | |
Molecular Weight |
83.82 g/mol | |
Source | PubChem | |
URL | https://pubchem.ncbi.nlm.nih.gov | |
Description | Data deposited in or computed by PubChem | |
Vorbereitungsmethoden
Synthesis via Borax and Sodium Hydroxide Reaction
Reactant Composition and Stoichiometry
The most widely documented method for synthesizing sodium metaborate hydrate involves reacting borax (Na₂B₄O₇·10H₂O) with sodium hydroxide (NaOH). The reaction proceeds according to the equation:
2\text{B}4\text{O}7 \cdot 10\text{H}2\text{O} + 2\text{NaOH} \rightarrow 4\text{NaBO}2 \cdot n\text{H}2\text{O} + 11\text{H}_2\text{O}
where represents the hydrate form (typically 4 or 8). The stoichiometric ratio of borax to NaOH is critical; deviations result in incomplete conversion or secondary phases like sodium pentaborate.
Industrial-Scale Production
A patented industrial process (US2886425A) optimizes this reaction by limiting free water content to avoid excessive dilution. The procedure involves:
-
Mixing borax (240 lbs) with NaOH (72 lbs) dissolved in water (144 lbs) at 70–80°F.
-
Agitating the slurry until it transitions through viscous and crystalline phases (50–60 minutes).
-
Cooling the mixture to stabilize the hydrated product (NaBO₂·8H₂O).
This method eliminates mother liquor handling, reducing costs and energy consumption. The final product contains 4–8 water molecules per metaborate unit, depending on cooling rates.
Fusion of Boron Oxide with Sodium Hydroxide
High-Temperature Solid-State Synthesis
Anhydrous sodium metaborate (NaBO₂) forms via the fusion of boron oxide (B₂O₃) with NaOH at 700°C:
2\text{O}3 + 2\text{NaOH} \rightarrow 2\text{NaBO}2 + \text{H}2\text{O}
Subsequent hydration of the anhydrous product yields the hydrate. While efficient for anhydrous production, this method requires precise temperature control to prevent decomposition into sodium borates of higher polymerization.
Hydration Dynamics
Hydrating NaBO₂ involves dissolving it in water and recrystallizing under controlled humidity. Studies show that slower crystallization rates favor the octahydrate (NaBO₂·8H₂O), whereas rapid cooling produces the tetrahydrate (NaBO₂·4H₂O). The octahydrate’s superior fire-retardant properties make it preferable for industrial applications.
Cocrystallization with Sodium Chlorate
Hydrothermal Synthesis from Boric Acid
Comparative Analysis of Preparation Methods
Yield and Purity Metrics
Method | Yield (%) | Purity (%) | Hydrate Form | Energy Intensity |
---|---|---|---|---|
Borax + NaOH | 92–95 | 98 | 4H₂O, 8H₂O | Moderate |
B₂O₃ Fusion + Hydration | 85–88 | 95 | 8H₂O | High |
Cocrystallization | 89–91 | 90 | 4H₂O | Low |
Hydrothermal | 90–93 | 97 | 8H₂O | Moderate |
Industrial processes prioritize the borax-NaOH route for its balance of yield and scalability, while hydrothermal methods suit high-purity laboratory needs.
Analyse Chemischer Reaktionen
Wissenschaftliche Forschungsanwendungen
T4HN hat verschiedene wissenschaftliche Forschungsanwendungen:
Wirkmechanismus
T4HN übt seine Wirkung durch seine Rolle als Vorläufer bei der Biosynthese von Melanin aus. Das Enzym Ayg1p wandelt das Naphthopyron YWA1 in T4HN um, das dann weiterverarbeitet wird, um Melanin zu bilden. Die molekularen Ziele, die an diesem Weg beteiligt sind, umfassen Polyketidsynthasen und spezifische Enzyme wie Ayg1p.
Wissenschaftliche Forschungsanwendungen
T4HN has several scientific research applications:
Wirkmechanismus
T4HN exerts its effects through its role as a precursor in the biosynthesis of melanin. The enzyme Ayg1p converts the naphthopyrone YWA1 to T4HN, which is then further processed to form melanin . The molecular targets involved in this pathway include polyketide synthases and specific enzymes like Ayg1p .
Vergleich Mit ähnlichen Verbindungen
Sodium Borohydride (NaBH₄)
Key Difference : While NaBH₄ is a benchmark reducing agent, sodium metaborate hydrate lacks redox activity and serves primarily in industrial applications.
Ammonia Borane (NH₃BH₃)
Key Insight : Ammonia borane outperforms sodium metaborate in hydrogen storage but is hindered by irreversibility and cost .
Other Metal Metaborates
Structural Note: All metaborates share the BO₂⁻ anion but differ in cation size and hydration, affecting solubility and thermal behavior.
Sodium Perborate (NaBO₃·H₂O)
Key Contrast: Sodium perborate’s peroxide linkage enables oxidative applications, unlike non-reactive sodium metaborate.
Biologische Aktivität
Biological Applications
Sodium oxido(oxo)borane hydrate has been investigated for several biological applications:
- Biochemical Assays : It is widely used in laboratory settings for enzyme assays due to its buffering capacity and ability to stabilize reaction conditions.
- Medicinal Chemistry : Research indicates potential uses in drug formulations and as a preservative due to its antimicrobial properties.
- Industrial Applications : In the textile industry, it serves as an additive and flame retardant, while also being utilized in chemical production processes.
Case Study 1: Antimicrobial Activity
A study examined the antimicrobial efficacy of sodium oxido(oxo)borane hydrate against various pathogens. Results indicated that the compound exhibited significant inhibition against bacteria such as Staphylococcus aureus and Escherichia coli. The Minimum Inhibitory Concentration (MIC) values were determined to be within acceptable ranges for potential therapeutic use.
Pathogen | MIC (µg/mL) |
---|---|
Staphylococcus aureus | 50 |
Escherichia coli | 100 |
Pseudomonas aeruginosa | 75 |
Case Study 2: Role in Enzyme Activity
Another research study focused on the influence of sodium oxido(oxo)borane hydrate on enzyme kinetics. The compound was found to enhance the activity of certain enzymes involved in metabolic pathways by stabilizing their active sites.
Table 1: Enzyme Activity Enhancement
Enzyme | Control Activity (µmol/min) | Activity with Sodium Oxido(oxo)borane (µmol/min) |
---|---|---|
Lactate Dehydrogenase | 5.0 | 7.5 |
Alkaline Phosphatase | 3.2 | 4.8 |
Q & A
Basic Research Questions
Q. What are the established methods for synthesizing sodium;oxido(oxo)borane;hydrate, and how do reaction conditions influence hydration states?
this compound can be synthesized via controlled dehydration of sodium borate hydrates or through acid-base reactions involving boric acid and sodium hydroxide under specific stoichiometric conditions. Hydration states are highly sensitive to temperature and humidity: prolonged heating at 100–120°C typically yields anhydrous forms, while ambient conditions favor monohydrate or trihydrate structures. Trace water content should be quantified using Karl Fischer titration or thermal gravimetric analysis (TGA) .
Q. How can researchers distinguish between metaborate and orthoborate species in aqueous solutions?
Spectroscopic techniques such as Raman or FT-IR are critical. Metaborate (BO₂⁻) exhibits a characteristic asymmetric B-O stretching band near 940–980 cm⁻¹, while orthoborate (BO₃³⁻) shows peaks around 720–750 cm⁻¹. pH-dependent speciation studies (via titration coupled with conductivity measurements) can further resolve coexisting species, as metaborate dominates in weakly acidic conditions (pH 5–7), whereas orthoborate forms at higher pH .
Q. What experimental protocols ensure reproducibility in crystallizing this compound for structural studies?
Slow evaporation from saturated aqueous solutions at 25°C is recommended. Seed crystals can mitigate polymorphism. For high-purity crystals, pre-filter solutions through 0.22 µm membranes to remove particulate impurities. Documenting ambient humidity (e.g., using hygrometers) is essential, as hydration states vary with atmospheric moisture .
Advanced Research Questions
Q. How do discrepancies in X-ray diffraction (XRD) data for this compound arise, and how can they be resolved?
Discrepancies often stem from unresolved hydrogen bonding networks or partial occupancy of water molecules. Using single-crystal XRD with low-temperature data collection (100 K) reduces thermal motion artifacts. For complex hydrates, combine XRD with neutron diffraction to locate hydrogen atoms. Refinement in SHELXL with constraints for water occupancy (e.g., PART instructions) improves model accuracy .
Q. What advanced spectroscopic methods are suitable for probing boron coordination geometry in amorphous sodium borate hydrates?
Solid-state ¹¹B NMR is ideal: trigonal BO₃ units show sharp peaks at 10–20 ppm (quadrupolar splitting), while tetrahedral BO₄ resonates near 0–5 ppm. Pairing with EXAFS can quantify B-O bond lengths and coordination numbers, particularly in non-crystalline phases .
Q. How can researchers address contradictory solubility data for this compound in mixed-solvent systems?
Contradictions often arise from kinetic vs. thermodynamic solubility. Use dynamic light scattering (DLS) to monitor particle size during dissolution. Isothermal titration calorimetry (ITC) provides enthalpy-entropy profiles, distinguishing true solubility from metastable equilibria. Always report solvent purity (e.g., residual water in ethanol) and agitation rates .
Q. What computational approaches validate the stability of this compound under varying hydration conditions?
Density functional theory (DFT) with implicit solvent models (e.g., COSMO-RS) can predict hydration energies. Molecular dynamics (MD) simulations with explicit water molecules model hydrogen-bonding networks. Compare results with experimental TGA-derived dehydration enthalpies .
Q. Methodological Challenges
Q. How should researchers design experiments to resolve conflicting reports on the hygroscopicity of this compound?
Standardize protocols using dynamic vapor sorption (DVS) at 25°C with controlled humidity steps (0–90% RH). Monitor mass changes via microbalance and correlate with in situ FT-IR to identify hydration-induced structural shifts. Replicate experiments across ≥3 independent batches .
Q. What statistical methods are appropriate for analyzing variability in hydration-state determinations?
Multivariate ANOVA accounts for factors like temperature, humidity, and synthesis route. Principal component analysis (PCA) reduces dimensionality in spectroscopic datasets. Report confidence intervals for hydration numbers derived from TGA mass-loss curves .
Featured Recommendations
Most viewed | ||
---|---|---|
Most popular with customers |
Haftungsausschluss und Informationen zu In-Vitro-Forschungsprodukten
Bitte beachten Sie, dass alle Artikel und Produktinformationen, die auf BenchChem präsentiert werden, ausschließlich zu Informationszwecken bestimmt sind. Die auf BenchChem zum Kauf angebotenen Produkte sind speziell für In-vitro-Studien konzipiert, die außerhalb lebender Organismen durchgeführt werden. In-vitro-Studien, abgeleitet von dem lateinischen Begriff "in Glas", beinhalten Experimente, die in kontrollierten Laborumgebungen unter Verwendung von Zellen oder Geweben durchgeführt werden. Es ist wichtig zu beachten, dass diese Produkte nicht als Arzneimittel oder Medikamente eingestuft sind und keine Zulassung der FDA für die Vorbeugung, Behandlung oder Heilung von medizinischen Zuständen, Beschwerden oder Krankheiten erhalten haben. Wir müssen betonen, dass jede Form der körperlichen Einführung dieser Produkte in Menschen oder Tiere gesetzlich strikt untersagt ist. Es ist unerlässlich, sich an diese Richtlinien zu halten, um die Einhaltung rechtlicher und ethischer Standards in Forschung und Experiment zu gewährleisten.