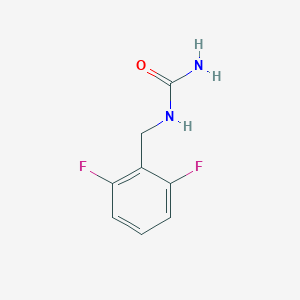
Urea, (2,6-difluorobenzyl)-
Übersicht
Beschreibung
Urea (2,6-difluorobenzyl) is a chemical compound that is widely used in scientific research applications. It is a derivative of urea and is also known as N-(2,6-difluorobenzyl)urea. This compound has gained significant attention due to its unique properties and potential applications in various fields.
Wissenschaftliche Forschungsanwendungen
- Additionally, these benzophenones display TADF, which is crucial for applications like time-gated biosensing and temperature sensing. The small energy gap between the excited singlet and triplet states enables TADF, making them potential molecular thermometers even under aqueous conditions .
- The closely packed lattice of methyl 4-bromo-3-((2,6-difluorophenyl)diazenyl) benzoate (an ortho-fluoroazobenzene derivative) inhibits photo-induced structural reorganization in the crystalline state. While the molecule is photoactive in solution, its behavior changes in the solid state due to crystal packing effects .
- The synthesis of 1-(3,4-difluorophenyl)-(1a) and 1-(2,4-difluorophenyl)-3-phenyl-1,4-dihydrobenzo[e][1,2,4]triazin-4-yl (1b) involves electrocyclic ring closure. These benzotriazines have potential applications in materials science, catalysis, and organic electronics .
Aggregation-Induced Emission (AIE) and Thermally Activated Delayed Fluorescence (TADF)
Photoactivity and Structural Reorganization
Electrocyclic Ring Closure and Benzotriazines
Eigenschaften
IUPAC Name |
(2,6-difluorophenyl)methylurea | |
---|---|---|
Source | PubChem | |
URL | https://pubchem.ncbi.nlm.nih.gov | |
Description | Data deposited in or computed by PubChem | |
InChI |
InChI=1S/C8H8F2N2O/c9-6-2-1-3-7(10)5(6)4-12-8(11)13/h1-3H,4H2,(H3,11,12,13) | |
Source | PubChem | |
URL | https://pubchem.ncbi.nlm.nih.gov | |
Description | Data deposited in or computed by PubChem | |
InChI Key |
XJGQUIHBNMXOQE-UHFFFAOYSA-N | |
Source | PubChem | |
URL | https://pubchem.ncbi.nlm.nih.gov | |
Description | Data deposited in or computed by PubChem | |
Canonical SMILES |
C1=CC(=C(C(=C1)F)CNC(=O)N)F | |
Source | PubChem | |
URL | https://pubchem.ncbi.nlm.nih.gov | |
Description | Data deposited in or computed by PubChem | |
Molecular Formula |
C8H8F2N2O | |
Source | PubChem | |
URL | https://pubchem.ncbi.nlm.nih.gov | |
Description | Data deposited in or computed by PubChem | |
DSSTOX Substance ID |
DTXSID30170321 | |
Record name | Urea, (2,6-difluorobenzyl)- | |
Source | EPA DSSTox | |
URL | https://comptox.epa.gov/dashboard/DTXSID30170321 | |
Description | DSSTox provides a high quality public chemistry resource for supporting improved predictive toxicology. | |
Molecular Weight |
186.16 g/mol | |
Source | PubChem | |
URL | https://pubchem.ncbi.nlm.nih.gov | |
Description | Data deposited in or computed by PubChem | |
Product Name |
Urea, (2,6-difluorobenzyl)- | |
CAS RN |
17751-24-5 | |
Record name | Urea, (2,6-difluorobenzyl)- | |
Source | ChemIDplus | |
URL | https://pubchem.ncbi.nlm.nih.gov/substance/?source=chemidplus&sourceid=0017751245 | |
Description | ChemIDplus is a free, web search system that provides access to the structure and nomenclature authority files used for the identification of chemical substances cited in National Library of Medicine (NLM) databases, including the TOXNET system. | |
Record name | Urea, (2,6-difluorobenzyl)- | |
Source | EPA DSSTox | |
URL | https://comptox.epa.gov/dashboard/DTXSID30170321 | |
Description | DSSTox provides a high quality public chemistry resource for supporting improved predictive toxicology. | |
Synthesis routes and methods I
Procedure details
Synthesis routes and methods II
Procedure details
Haftungsausschluss und Informationen zu In-Vitro-Forschungsprodukten
Bitte beachten Sie, dass alle Artikel und Produktinformationen, die auf BenchChem präsentiert werden, ausschließlich zu Informationszwecken bestimmt sind. Die auf BenchChem zum Kauf angebotenen Produkte sind speziell für In-vitro-Studien konzipiert, die außerhalb lebender Organismen durchgeführt werden. In-vitro-Studien, abgeleitet von dem lateinischen Begriff "in Glas", beinhalten Experimente, die in kontrollierten Laborumgebungen unter Verwendung von Zellen oder Geweben durchgeführt werden. Es ist wichtig zu beachten, dass diese Produkte nicht als Arzneimittel oder Medikamente eingestuft sind und keine Zulassung der FDA für die Vorbeugung, Behandlung oder Heilung von medizinischen Zuständen, Beschwerden oder Krankheiten erhalten haben. Wir müssen betonen, dass jede Form der körperlichen Einführung dieser Produkte in Menschen oder Tiere gesetzlich strikt untersagt ist. Es ist unerlässlich, sich an diese Richtlinien zu halten, um die Einhaltung rechtlicher und ethischer Standards in Forschung und Experiment zu gewährleisten.