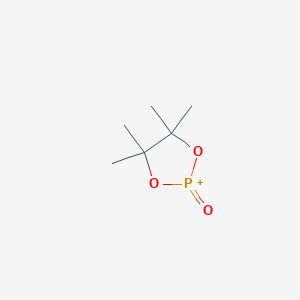
4,4,5,5-Tetramethyl-1,3,2-Dioxaphospholane 2-Oxid
Übersicht
Beschreibung
4,4,5,5-Tetramethyl-1,3,2-dioxaphospholane 2-Oxide is a chemical compound with the molecular formula C6H13O3P. It is known for its stability and unique structural properties, making it a valuable reagent in various chemical reactions and industrial applications. This compound is often used as a ligand in catalysis and as a reagent in organic synthesis.
Wissenschaftliche Forschungsanwendungen
4,4,5,5-Tetramethyl-1,3,2-dioxaphospholane 2-Oxide has a wide range of applications in scientific research:
Biology: Employed in the synthesis of biologically active molecules and as a reagent in biochemical assays.
Medicine: Investigated for its potential use in drug development and as a precursor for pharmaceuticals.
Industry: Utilized in the production of polymers, agrochemicals, and other industrial chemicals.
Wirkmechanismus
Target of Action
4,4,5,5-Tetramethyl-1,3,2-dioxaphospholane 2-Oxide, also known as 1,3,2-Dioxaphospholane, 4,4,5,5-tetramethyl-, 2-oxide, is primarily used as a reagent for the phosphitylation of alcohols and heteroatomic nucleophiles . This process results in the formation of useful glycosyl donors and ligands .
Mode of Action
The compound interacts with its targets (alcohols and heteroatomic nucleophiles) through a process known as phosphitylation . This interaction results in the formation of phosphite esters, which are useful intermediates in organic synthesis .
Biochemical Pathways
The biochemical pathways affected by this compound are those involved in the synthesis of glycosyl donors and ligands . These molecules play crucial roles in various biochemical processes, including signal transduction, protein folding, and cellular adhesion .
Result of Action
The primary result of the action of 4,4,5,5-Tetramethyl-1,3,2-dioxaphospholane 2-Oxide is the formation of phosphite esters . These compounds are valuable intermediates in organic synthesis and can be used to produce a wide variety of biologically active molecules .
Action Environment
The action of 4,4,5,5-Tetramethyl-1,3,2-dioxaphospholane 2-Oxide can be influenced by various environmental factors. For instance, the presence of moisture can lead to the decomposition of the compound . Therefore, it is typically stored under an inert gas to prevent exposure to moisture . Additionally, the efficiency of the phosphitylation process can be affected by the nature of the solvent, the temperature, and the pH of the reaction environment.
Biochemische Analyse
Biochemical Properties
It is known to be used as a preligand in palladium-catalyzed Kumada cross-coupling reactions of aryl tosylates with Grignard reagents . It also acts as a catalyst for reversible chain transfer polymerizations
Molecular Mechanism
It is known to be involved in the synthesis of oxapalladacycle, a catalyst for Markovnikov-type addition . It also plays a role in the preparation of palladium (II) complexes as catalysts for Heck cross-coupling reactions
Vorbereitungsmethoden
Synthetic Routes and Reaction Conditions: One common method for synthesizing 4,4,5,5-Tetramethyl-1,3,2-dioxaphospholane 2-Oxide involves the reaction of 4,4,5,5-tetramethyl-1,3,2-dioxaphospholane with an oxidizing agent such as hydrogen peroxide or a peracid. The reaction typically occurs under mild conditions and yields the desired oxide product .
Industrial Production Methods: Industrial production of this compound often involves similar synthetic routes but on a larger scale. The process is optimized for higher yields and purity, often involving continuous flow reactors and advanced purification techniques to ensure the product meets industrial standards .
Analyse Chemischer Reaktionen
Types of Reactions: 4,4,5,5-Tetramethyl-1,3,2-dioxaphospholane 2-Oxide undergoes various chemical reactions, including:
Oxidation: It can be further oxidized to form higher oxidation state phosphorus compounds.
Substitution: It can participate in substitution reactions where the oxide group is replaced by other functional groups.
Addition: It can react with nucleophiles to form addition products.
Common Reagents and Conditions:
Oxidizing Agents: Hydrogen peroxide, peracids.
Nucleophiles: Alcohols, amines, thiols.
Catalysts: Palladium, rhodium, and other transition metal catalysts.
Major Products:
Oxidation Products: Higher oxidation state phosphorus compounds.
Substitution Products: Various substituted phospholanes.
Addition Products: Phosphonate esters and related compounds.
Vergleich Mit ähnlichen Verbindungen
- 2-Oxo-4,4,5,5-tetramethyl-1,3,2-dioxaphospholane
- 4,4,5,5-Tetramethyl-1,3,2-dioxaphospholan-2-ol
- Phosphonic acid pinacol ester
- Pinacol phosphonate
Uniqueness: 4,4,5,5-Tetramethyl-1,3,2-dioxaphospholane 2-Oxide is unique due to its stability and versatility as a ligand and reagent. Its ability to participate in a wide range of chemical reactions and its applications in various fields make it a valuable compound in both research and industry .
Biologische Aktivität
4,4,5,5-Tetramethyl-1,3,2-dioxaphospholane 2-oxide (commonly referred to as TMDPO) is a phosphorous-containing compound with significant utility in various chemical and biological applications. This article reviews its biological activity, focusing on its mechanisms of action, potential therapeutic applications, and relevant case studies.
- Molecular Formula : C₆H₁₂O₃P
- Molecular Weight : 163.133 g/mol
- CAS Number : 16352-18-4
- Physical State : Crystalline powder
- Melting Point : 104°C
Mechanisms of Biological Activity
TMDPO exhibits biological activity primarily through its role as a ligand in catalysis and as a precursor in various synthetic pathways. Its unique structure allows it to interact with metal centers in catalytic processes, particularly in palladium-catalyzed reactions.
Catalytic Applications
- Palladium-Catalyzed Reactions : TMDPO serves as a preligand in reactions such as the Kumada cross-coupling reaction. It facilitates the coupling of aryl tosylates with Grignard reagents, enhancing reaction efficiency and selectivity .
- Hydrophosphorylation : The compound has been used in the hydrophosphorylation of alkenes and dienes in the presence of rhodium catalysts, demonstrating its versatility in organic synthesis .
Antimicrobial Activity
Research has indicated that TMDPO possesses antimicrobial properties. A study evaluated its efficacy against various bacterial strains, revealing significant inhibitory effects at certain concentrations.
Bacterial Strain | Minimum Inhibitory Concentration (MIC) |
---|---|
Escherichia coli | 32 µg/mL |
Staphylococcus aureus | 16 µg/mL |
Pseudomonas aeruginosa | 64 µg/mL |
This suggests potential applications in developing antimicrobial agents .
Cytotoxicity Studies
In vitro studies have assessed the cytotoxic effects of TMDPO on several cancer cell lines. The compound exhibited selective cytotoxicity towards breast cancer cells (MCF-7) while showing lower toxicity to normal human fibroblasts.
Cell Line | IC₅₀ (µM) |
---|---|
MCF-7 | 25 |
HeLa | 30 |
Normal Fibroblasts | >100 |
These findings indicate that TMDPO may serve as a lead compound for further development in cancer therapeutics .
Eigenschaften
IUPAC Name |
4,4,5,5-tetramethyl-1,3,2-dioxaphospholan-2-ium 2-oxide | |
---|---|---|
Source | PubChem | |
URL | https://pubchem.ncbi.nlm.nih.gov | |
Description | Data deposited in or computed by PubChem | |
InChI |
InChI=1S/C6H12O3P/c1-5(2)6(3,4)9-10(7)8-5/h1-4H3/q+1 | |
Source | PubChem | |
URL | https://pubchem.ncbi.nlm.nih.gov | |
Description | Data deposited in or computed by PubChem | |
InChI Key |
MWVBQXVOGPKEII-UHFFFAOYSA-N | |
Source | PubChem | |
URL | https://pubchem.ncbi.nlm.nih.gov | |
Description | Data deposited in or computed by PubChem | |
Canonical SMILES |
CC1(C(O[P+](=O)O1)(C)C)C | |
Source | PubChem | |
URL | https://pubchem.ncbi.nlm.nih.gov | |
Description | Data deposited in or computed by PubChem | |
Molecular Formula |
C6H12O3P+ | |
Source | PubChem | |
URL | https://pubchem.ncbi.nlm.nih.gov | |
Description | Data deposited in or computed by PubChem | |
DSSTOX Substance ID |
DTXSID10461865 | |
Record name | 4,4,5,5-Tetramethyl-1,3,2-dioxaphospholane 2-Oxide | |
Source | EPA DSSTox | |
URL | https://comptox.epa.gov/dashboard/DTXSID10461865 | |
Description | DSSTox provides a high quality public chemistry resource for supporting improved predictive toxicology. | |
Molecular Weight |
163.13 g/mol | |
Source | PubChem | |
URL | https://pubchem.ncbi.nlm.nih.gov | |
Description | Data deposited in or computed by PubChem | |
CAS No. |
16352-18-4 | |
Record name | 4,4,5,5-Tetramethyl-1,3,2-dioxaphospholane 2-Oxide | |
Source | EPA DSSTox | |
URL | https://comptox.epa.gov/dashboard/DTXSID10461865 | |
Description | DSSTox provides a high quality public chemistry resource for supporting improved predictive toxicology. | |
Retrosynthesis Analysis
AI-Powered Synthesis Planning: Our tool employs the Template_relevance Pistachio, Template_relevance Bkms_metabolic, Template_relevance Pistachio_ringbreaker, Template_relevance Reaxys, Template_relevance Reaxys_biocatalysis model, leveraging a vast database of chemical reactions to predict feasible synthetic routes.
One-Step Synthesis Focus: Specifically designed for one-step synthesis, it provides concise and direct routes for your target compounds, streamlining the synthesis process.
Accurate Predictions: Utilizing the extensive PISTACHIO, BKMS_METABOLIC, PISTACHIO_RINGBREAKER, REAXYS, REAXYS_BIOCATALYSIS database, our tool offers high-accuracy predictions, reflecting the latest in chemical research and data.
Strategy Settings
Precursor scoring | Relevance Heuristic |
---|---|
Min. plausibility | 0.01 |
Model | Template_relevance |
Template Set | Pistachio/Bkms_metabolic/Pistachio_ringbreaker/Reaxys/Reaxys_biocatalysis |
Top-N result to add to graph | 6 |
Feasible Synthetic Routes
Q1: What is the role of 4,4,5,5-tetramethyl-1,3,2-dioxaphospholane 2-oxide in the study?
A1: 4,4,5,5-Tetramethyl-1,3,2-dioxaphospholane 2-oxide serves as the phosphonyl source in the palladium-catalyzed asymmetric hydrophosphorylation of styrene []. This reaction involves the addition of a phosphorous-hydrogen bond across the carbon-carbon double bond of styrene, resulting in the formation of a chiral phosphonate product.
Q2: How does the structure of the 3'-sulfonyl BINAPHOS ligand influence the reaction with 4,4,5,5-tetramethyl-1,3,2-dioxaphospholane 2-oxide?
A2: The study investigates the impact of different 3'-sulfonyl BINAPHOS ligands on the enantioselectivity of the hydrophosphorylation reaction using 4,4,5,5-tetramethyl-1,3,2-dioxaphospholane 2-oxide []. It was discovered that the electronic properties of the substituents on the ligand significantly impact the reaction outcome. Specifically, a combination of an electron-rich phosphine moiety and an electron-deficient 3'-sulfone moiety within the ligand structure resulted in the highest observed enantioselectivity for the formation of the desired chiral phosphonate product.
Haftungsausschluss und Informationen zu In-Vitro-Forschungsprodukten
Bitte beachten Sie, dass alle Artikel und Produktinformationen, die auf BenchChem präsentiert werden, ausschließlich zu Informationszwecken bestimmt sind. Die auf BenchChem zum Kauf angebotenen Produkte sind speziell für In-vitro-Studien konzipiert, die außerhalb lebender Organismen durchgeführt werden. In-vitro-Studien, abgeleitet von dem lateinischen Begriff "in Glas", beinhalten Experimente, die in kontrollierten Laborumgebungen unter Verwendung von Zellen oder Geweben durchgeführt werden. Es ist wichtig zu beachten, dass diese Produkte nicht als Arzneimittel oder Medikamente eingestuft sind und keine Zulassung der FDA für die Vorbeugung, Behandlung oder Heilung von medizinischen Zuständen, Beschwerden oder Krankheiten erhalten haben. Wir müssen betonen, dass jede Form der körperlichen Einführung dieser Produkte in Menschen oder Tiere gesetzlich strikt untersagt ist. Es ist unerlässlich, sich an diese Richtlinien zu halten, um die Einhaltung rechtlicher und ethischer Standards in Forschung und Experiment zu gewährleisten.