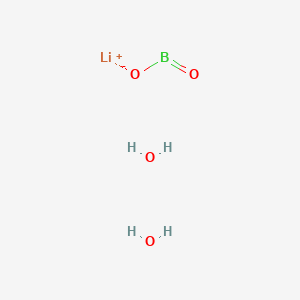
Lithium metaborate dihydrate
Übersicht
Beschreibung
Lithium metaborate dihydrate is a chemical compound composed of lithium, boron, oxygen, and water molecules. Its chemical formula is LiBO₂·2H₂O. This compound is often encountered as a white, hygroscopic crystalline powder. It is widely used in various industrial and scientific applications due to its unique properties, such as its ability to dissolve acidic oxides and its role as a flux in analytical chemistry .
Vorbereitungsmethoden
Laboratory-Scale Synthesis of Lithium Metaborate Dihydrate
Reaction of Lithium Hydroxide and Boric Acid
The most widely documented method for synthesizing this compound involves the neutralization of lithium hydroxide (LiOH) with orthoboric acid (H₃BO₃) in an aqueous medium. The reaction proceeds as follows:
This exothermic reaction requires careful temperature modulation to prevent premature crystallization. Studies indicate that maintaining the solution at 60–80°C ensures complete dissolution of reactants, followed by gradual cooling to 25°C to precipitate high-purity LiBO₂·2H₂O crystals . Excess water must be evaporated under reduced pressure to avoid forming higher hydrates, such as the octahydrate (LiBO₂·8H₂O) .
Table 1: Optimal Conditions for LiOH-H₃BO₃ Reaction
Parameter | Optimal Value | Impact on Yield |
---|---|---|
Molar Ratio (Li:B) | 1:1 | Prevents Li₂B₄O₇ formation |
Temperature | 70°C | Maximizes dissolution |
Cooling Rate | 2°C/min | Enhances crystal purity |
Evaporation Pressure | 0.5 atm | Avoids octahydrate contamination |
Alternative Reactants: Lithium Carbonate and Boric Acid
Lithium carbonate (Li₂CO₃) serves as a cost-effective alternative to LiOH in industrial settings. The reaction with boric acid proceeds via carbon dioxide evolution:
This method requires pressurized reactors to mitigate CO₂-induced foaming and ensure stoichiometric conversion. Research by Yalçın and Gönen demonstrated that introducing CO₂ at 10–40 bar enhances reactant solubility, achieving 95% yield within 2 hours at 50°C . However, residual Li₂CO₃ may persist if the reaction pH exceeds 9.0, necessitating post-synthesis acid washing .
Industrial Production Methods
Industrial-scale production prioritizes cost efficiency and batch consistency. A typical流程 involves:
-
Continuous Reactor Systems : LiOH and H₃BO₃ are fed into a jacketed reactor at 80°C with automated pH control (target pH: 6.5–7.0).
-
Crystallization : The hot solution is transferred to cooling crystallizers equipped with scraping blades to prevent agglomeration.
-
Centrifugation and Drying : Crystals are separated via sieve centrifuges and dried at 110°C in fluidized-bed dryers to maintain the dihydrate structure .
Table 2: Industrial Process Parameters
Stage | Equipment | Key Parameters |
---|---|---|
Reaction | Jacketed Reactor | T=80°C, τ=45 min |
Crystallization | Scraped Crystallizer | Cooling rate=1.5°C/min |
Drying | Fluidized-Bed Dryer | T=110°C, τ=30 min |
Thermal Dehydration of Lithium Metaborate Octahydrate
Lithium metaborate octahydrate (LiBO₂·8H₂O) serves as a precursor for dihydrate synthesis. Controlled dehydration at 90–100°C under nitrogen atmosphere yields phase-pure LiBO₂·2H₂O:
Thermogravimetric analysis (TGA) reveals a two-step mass loss: initial dehydration (25–100°C) removes six water molecules, while the remaining two are structurally bound until 140°C . Overheating beyond 140°C risks forming anhydrous LiBO₂, which exhibits reduced flux capacity due to structural reorganization .
Quality Control and Analytical Techniques
Titrimetric Analysis
The B₂O₃ content in LiBO₂·2H₂O is quantified via titration with 0.1M HCl, using methyl red as an indicator. A study reported 40.56% B₂O₃ in synthesized samples, aligning with the theoretical value of 41.23% . Discrepancies >2% suggest contamination with Li₂B₄O₇ or unreacted H₃BO₃.
Spectroscopic and Diffraction Methods
-
XRD : Confirms monoclinic crystal structure (space group P2₁/c) with lattice parameters a=5.838 Å, b=4.348 Å, c=6.449 Å .
-
FTIR : B-O stretching vibrations at 1,380 cm⁻¹ and 950 cm⁻¹ verify trigonal BO₃ units .
Comparative Analysis of Synthesis Methods
Table 3: Method Comparison
Method | Purity (%) | Yield (%) | Energy Cost (kWh/kg) |
---|---|---|---|
LiOH + H₃BO₃ | 99.2 | 88 | 12.5 |
Li₂CO₃ + H₃BO₃ | 97.8 | 95 | 9.8 |
Thermal Dehydration | 98.5 | 82 | 15.3 |
The Li₂CO₃ route offers higher yield and lower energy costs but requires stringent pH control. Laboratory-scale LiOH reactions yield purer products, making them preferable for analytical applications .
Analyse Chemischer Reaktionen
Thermal Decomposition and Phase Transitions
Lithium metaborate dihydrate undergoes dehydration and structural reorganization upon heating:
Key Findings :
-
The ratio of tetrahedral to trigonal boron in glassy lithium metaborate is temperature-dependent, affecting reactivity .
-
At 400°C, anhydrous LiBO₂ reacts with B₂O₃ (from boric acid dehydration) to yield Li₂B₄O₇, bypassing the need for 700°C+ temperatures required in traditional solid-state syntheses .
Reactions with Acids and Bases
This compound participates in acid-base reactions due to its borate anion:
Acid Dissolution
Alkaline Conditions
-
In basic solutions, metaborate anions may further hydrolyze:
Oxidation
-
Acts as an oxidizing agent in molten states, facilitating the conversion of metal oxides (e.g., Fe₂O₃) to corresponding borates .
Substitution
Role in Flux Reactions
This compound is employed as a flux in analytical chemistry due to its ability to dissolve refractory oxides (e.g., SiO₂, Al₂O₃) at elevated temperatures.
Mechanism :
-
Forms low-melting eutectic mixtures, enhancing oxide solubility.
-
Structural flexibility (trigonal ↔ tetrahedral boron) aids in stabilizing dissolved species .
Environmental and Kinetic Factors
Wissenschaftliche Forschungsanwendungen
Analytical Chemistry
Flux in Spectrochemical Analysis
- Lithium metaborate dihydrate is widely used as a flux in analytical techniques such as X-ray fluorescence (XRF) and inductively coupled plasma (ICP) analyses. It facilitates the dissolution of oxide samples, allowing for accurate elemental analysis. The compound effectively dissolves various acidic oxides including SiO₂, Al₂O₃, and TiO₂, making it essential for preparing samples for spectrochemical analysis .
Comparison with Other Fluxes
Compound | Type of Oxides Dissolved | Unique Properties |
---|---|---|
Lithium Metaborate | Acidic oxides | High solubility and hygroscopic nature |
Lithium Tetraborate | Basic oxides | Used in conjunction with lithium metaborate for comprehensive analysis |
Sodium Metaborate | Similar to lithium metaborate | Different reactivity and solubility |
Material Science
Glass and Ceramics Production
- This compound plays a crucial role in the production of glasses and ceramics. Its ability to form stable glassy phases is utilized in creating materials with specific optical and thermal properties. The compound's unique crystalline structure allows for the manipulation of boron content, which is vital in tailoring the properties of glass materials .
Nonlinear Optical Materials
- The compound is also explored for its applications in nonlinear optics, particularly in devices like cellular phones and pagers. Its properties make it suitable for use in optical components that require specific refractive indices .
Biological Studies
Antioxidant Properties
- Recent studies have investigated the potential antioxidant effects of this compound. Research indicates that it exhibits antioxidant activity at low concentrations without genotoxic effects on cultured human blood cells. This suggests its potential use in biomedical applications where oxidative stress is a concern .
Environmental Science Applications
- In environmental studies, this compound has been examined for its ability to mitigate oxidative stress caused by heavy metals in organisms. This property could be harnessed to develop strategies for protecting ecosystems from pollution-induced damage .
Industrial Applications
Chemical Processes
- The compound is utilized in various chemical processes, including the synthesis of low-density aluminum oxides under high-pressure conditions. Its role as a solvent helps facilitate reactions that would otherwise be challenging due to the stability of reactants .
Protective Coatings
- This compound can also serve as a protective coating layer for lithium-ion battery cathodes due to its chemical inertness in organic electrolytes, enhancing the durability and performance of battery materials .
Case Studies
Case Study 1: Antioxidant Activity Assessment
A study evaluated the cytogenetic and oxidative effects of this compound on human peripheral blood cultures. The results indicated that while higher concentrations exhibited antioxidant properties, lower doses did not significantly alter total oxidant status or total antioxidant capacity compared to controls. This highlights its potential safety profile for use in various industries .
Case Study 2: Use in Glass Production
In material science research, this compound was combined with other borates to create glass with enhanced thermal stability and optical clarity. The experiments demonstrated that varying the ratio of tetrahedral to trigonal boron significantly influenced the glass properties, showcasing the importance of this compound in developing advanced materials .
Wirkmechanismus
The mechanism by which lithium metaborate dihydrate exerts its effects varies depending on the application:
In Analytical Chemistry: It acts as a flux, breaking down complex oxide matrices to release individual elements for analysis.
In Biological Systems: It has been shown to reduce oxidative stress by decreasing lipid peroxidation and enhancing antioxidant enzyme activities.
In Material Science: It facilitates the formation of glassy phases by disrupting the crystalline structure of the starting materials.
Vergleich Mit ähnlichen Verbindungen
Lithium Tetraborate (Li₂B₄O₇): Another borate compound used as a flux in analytical chemistry.
Sodium Metaborate (NaBO₂): Similar in structure but contains sodium instead of lithium. It has different solubility and reactivity properties.
Potassium Metaborate (KBO₂): Contains potassium and has distinct physical and chemical properties compared to lithium metaborate.
Uniqueness: Lithium metaborate dihydrate is unique due to its high solubility in water and its ability to dissolve a wide range of acidic oxides. Its hygroscopic nature and stability make it particularly useful in various analytical and industrial applications .
Biologische Aktivität
Lithium metaborate dihydrate (LMBDH), a lithium borate compound, has garnered attention for its potential biological activities, particularly concerning its antioxidant properties and effects on trace element homeostasis. This article synthesizes findings from various studies to provide a comprehensive overview of the biological activity of LMBDH.
This compound is represented by the chemical formula . It is characterized by its high solubility in water and ability to dissolve acidic oxides, making it useful in various analytical and industrial applications. The compound's structure consists of infinite chains of trigonal planar metaborate anions, which contribute to its unique chemical behavior and interactions in biological systems.
Target of Action : LMBDH primarily targets acidic oxides, facilitating their dissolution.
Mode of Action : The compound interacts with these targets by disrupting their structural integrity, leading to biochemical changes that can affect cellular functions.
Biochemical Pathways : Research indicates that LMBDH may influence the antioxidant defense system by modulating levels of malondialdehyde (MDA) and superoxide dismutase (SOD), suggesting a protective role against oxidative stress .
Antioxidant Activity
A pivotal study investigated the effects of LMBDH on oxidative stress induced by cadmium chloride (CdCl₂) in Wistar albino male rats. The results demonstrated that pretreatment with LMBDH significantly decreased MDA levels (a marker of lipid peroxidation) and increased SOD activity, indicating enhanced antioxidant defenses. Specifically, the study reported:
- MDA Levels : Decreased significantly in the LMBDH pretreatment group compared to controls.
- SOD Activity : Increased significantly (p < 0.05) in the presence of LMBDH.
- Trace Elements : Alterations were observed in calcium (Ca), iron (Fe), potassium (K), and magnesium (Mg) levels, suggesting a role in trace element homeostasis .
Table 1: Effects of LMBDH on Biological Parameters
Parameter | Control Group | CdCl₂ Group | LMBDH Group | CdCl₂ + LMBDH Group |
---|---|---|---|---|
MDA Levels | Baseline | Increased | Decreased | Significantly Decreased |
SOD Activity | Baseline | Decreased | Increased | Significantly Increased |
Ca Levels | Normal | Decreased | Normal | Decreased |
Fe Levels | Normal | Decreased | Normal | Decreased |
K Levels | Normal | Decreased | Normal | Decreased |
Mg Levels | Normal | Decreased | Increased | Increased |
Pharmacokinetics
The pharmacokinetics of lithium compounds, including LMBDH, suggest that lithium clearance is influenced by renal function and body size. This has implications for dosing and efficacy in biological applications.
Case Studies and Applications
- Antioxidant Studies : The protective effects against heavy metal-induced oxidative stress have been documented, highlighting the potential therapeutic applications of LMBDH in mitigating environmental toxicity .
- Industrial Applications : Beyond its biological implications, LMBDH is utilized as a flux in analytical chemistry for elemental analysis through X-ray fluorescence (XRF) and inductively coupled plasma (ICP) techniques.
- Material Science : Its ability to form stable glassy phases makes it valuable in material science for the preparation of glasses and ceramics.
Q & A
Basic Research Questions
Q. What are the primary research applications of lithium metaborate dihydrate in analytical chemistry?
this compound is widely used in fusion-based sample preparation for X-ray fluorescence (XRF) and inductively coupled plasma (ICP) analyses. Its low melting point (845°C) and reactivity with acidic samples enable efficient decomposition of silicate minerals and oxides. For XRF, fusion with lithium metaborate produces homogeneous glass disks, minimizing matrix effects and improving quantification of major elements like Si, Al, and Fe . In ICP-MS, fusion facilitates complete dissolution of refractory materials, though trace element analysis may require post-fusion acid digestion to recover volatile elements (e.g., As, Hg) .
Q. What are the recommended storage conditions for this compound to ensure stability?
Store this compound in cool (<25°C), dry, and well-ventilated environments , tightly sealed in its original container to prevent moisture absorption. Exposure to humidity can lead to deliquescence, altering stoichiometry and reactivity. Storage areas must avoid ignition sources, electrostatic charges, and incompatible materials (e.g., strong acids) .
Q. How does the hygroscopic nature of this compound affect experimental protocols?
The compound’s hygroscopicity necessitates rigorous moisture control during weighing and handling. Use a glovebox or desiccator for sample preparation, and pre-dry reagents at 105°C for 1–2 hours before fusion to minimize water content. Post-fusion cooling should occur in a dry atmosphere to prevent rehydration, which can introduce errors in gravimetric analyses .
Advanced Research Questions
Q. How can phase diagrams inform the selection of this compound in high-temperature synthesis?
Phase equilibria studies (e.g., LiOH–B(OH)₃–H₂O systems) reveal that this compound forms under basic conditions at elevated temperatures (60°C) . Its stability range guides synthesis protocols for borate-based ceramics or glasses. For example, excess LiOH shifts equilibria toward lithium hydroxide monohydrate, requiring precise pH and temperature control to isolate the dihydrate phase .
Q. What methodological considerations are critical when using this compound fusion for XRF versus ICP-MS sample preparation?
- XRF : Optimize flux-to-sample ratios (typically 5:1 to 10:1) and fusion temperatures (900–1000°C) to ensure complete oxidation of organic matter and mineral decomposition. Use platinum crucibles to avoid contamination .
- ICP-MS : Post-fusion digestion with HNO₃/HF is required to dissolve borate matrices and recover trace elements. Internal standards (e.g., In) mitigate signal drift caused by high dissolved solids .
Q. How should researchers address discrepancies in elemental recovery data when using different decomposition methods with this compound?
Discrepancies often arise from incomplete dissolution of refractory minerals (e.g., zircon, chromite) during fusion or volatilization losses during high-temperature steps. To resolve contradictions:
- Cross-validate with alternative methods (e.g., pressurised acid digestion for ICP-MS).
- Perform recovery tests using certified reference materials (CRMs) with matched matrices.
- Adjust fusion parameters (e.g., longer heating times) or employ mixed fluxes (lithium tetraborate/metaborate) for complex samples .
Q. Methodological Best Practices
-
Fusion Protocol :
-
Quality Control :
- Include reagent blanks and CRMs in each batch.
- Monitor boron backgrounds in ICP-MS via B signals .
Eigenschaften
IUPAC Name |
lithium;oxido(oxo)borane;dihydrate | |
---|---|---|
Source | PubChem | |
URL | https://pubchem.ncbi.nlm.nih.gov | |
Description | Data deposited in or computed by PubChem | |
InChI |
InChI=1S/BO2.Li.2H2O/c2-1-3;;;/h;;2*1H2/q-1;+1;; | |
Source | PubChem | |
URL | https://pubchem.ncbi.nlm.nih.gov | |
Description | Data deposited in or computed by PubChem | |
InChI Key |
MAXQFCAELLTPNS-UHFFFAOYSA-N | |
Source | PubChem | |
URL | https://pubchem.ncbi.nlm.nih.gov | |
Description | Data deposited in or computed by PubChem | |
Canonical SMILES |
[Li+].B(=O)[O-].O.O | |
Source | PubChem | |
URL | https://pubchem.ncbi.nlm.nih.gov | |
Description | Data deposited in or computed by PubChem | |
Molecular Formula |
BH4LiO4 | |
Source | PubChem | |
URL | https://pubchem.ncbi.nlm.nih.gov | |
Description | Data deposited in or computed by PubChem | |
DSSTOX Substance ID |
DTXSID30635440 | |
Record name | lithium;oxido(oxo)borane;dihydrate | |
Source | EPA DSSTox | |
URL | https://comptox.epa.gov/dashboard/DTXSID30635440 | |
Description | DSSTox provides a high quality public chemistry resource for supporting improved predictive toxicology. | |
Molecular Weight |
85.8 g/mol | |
Source | PubChem | |
URL | https://pubchem.ncbi.nlm.nih.gov | |
Description | Data deposited in or computed by PubChem | |
CAS No. |
15293-74-0 | |
Record name | lithium;oxido(oxo)borane;dihydrate | |
Source | EPA DSSTox | |
URL | https://comptox.epa.gov/dashboard/DTXSID30635440 | |
Description | DSSTox provides a high quality public chemistry resource for supporting improved predictive toxicology. | |
Haftungsausschluss und Informationen zu In-Vitro-Forschungsprodukten
Bitte beachten Sie, dass alle Artikel und Produktinformationen, die auf BenchChem präsentiert werden, ausschließlich zu Informationszwecken bestimmt sind. Die auf BenchChem zum Kauf angebotenen Produkte sind speziell für In-vitro-Studien konzipiert, die außerhalb lebender Organismen durchgeführt werden. In-vitro-Studien, abgeleitet von dem lateinischen Begriff "in Glas", beinhalten Experimente, die in kontrollierten Laborumgebungen unter Verwendung von Zellen oder Geweben durchgeführt werden. Es ist wichtig zu beachten, dass diese Produkte nicht als Arzneimittel oder Medikamente eingestuft sind und keine Zulassung der FDA für die Vorbeugung, Behandlung oder Heilung von medizinischen Zuständen, Beschwerden oder Krankheiten erhalten haben. Wir müssen betonen, dass jede Form der körperlichen Einführung dieser Produkte in Menschen oder Tiere gesetzlich strikt untersagt ist. Es ist unerlässlich, sich an diese Richtlinien zu halten, um die Einhaltung rechtlicher und ethischer Standards in Forschung und Experiment zu gewährleisten.