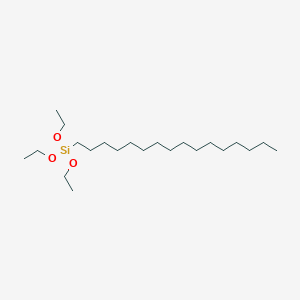
Hexadecyltriethoxysilan
Übersicht
Beschreibung
Hexadecyltriethoxysilane is a monomeric long-chain alkylfunctional silane. It is a clear, colorless liquid that is soluble in organic solvents but not in water. This compound is known for its superior hydrophobicity and is widely used as a water-repellent or surface modifier .
Wissenschaftliche Forschungsanwendungen
Hexadecyltriethoxysilane has a wide range of applications in scientific research:
Wirkmechanismus
Target of Action
Hexadecyltriethoxysilane (HDTMS) primarily targets metal oxide nanoparticles such as CeO2, Fe3O4, TiO2, and ZnO . These nanoparticles are used in a variety of applications, including electronics, energy storage, and catalysis .
Mode of Action
HDTMS interacts with its targets by coating the metal oxide nanoparticles . This coating process occurs when the nanoparticles and the organosilane are refluxed in a hydrocarbon solvent . The organosilane-coated metal oxide nanoparticles give stable dispersions in hydrocarbon solvents due to their hydrophobic surface .
Biochemical Pathways
The coating of metal oxide nanoparticles by hdtms can influence the properties of these nanoparticles, potentially affecting their interactions with other substances and their stability in various environments .
Pharmacokinetics
Given its use in coating nanoparticles, it is likely that these properties would be significantly influenced by the nature of the nanoparticles it is associated with, as well as the specific environment in which it is used .
Result of Action
The primary result of HDTMS’s action is the creation of hydrophobic surfaces on metal oxide nanoparticles . This hydrophobicity allows the nanoparticles to form stable dispersions in hydrocarbon solvents . On heating in air, the organosilane-coated metal oxide nanoparticles yield to silica-coated core-shell type nanoparticles .
Action Environment
The action of HDTMS is influenced by environmental factors such as temperature and the presence of a hydrocarbon solvent . For instance, the coating process occurs when the nanoparticles and the organosilane are refluxed in a hydrocarbon solvent . Additionally, heating in air can lead to the formation of silica-coated core-shell type nanoparticles .
Biochemische Analyse
Biochemical Properties
Hexadecyltriethoxysilane has been found to interact with metal oxide nanoparticles such as CeO2, Fe3O4, TiO2, and ZnO . The organosilane-coated metal oxide nanoparticles give stable dispersions in hydrocarbon solvents due to their hydrophobic surface .
Cellular Effects
Its role in coating metal oxide nanoparticles suggests that it may influence cellular processes by altering the properties of these nanoparticles .
Molecular Mechanism
It is known to exert its effects at the molecular level by coating metal oxide nanoparticles, thereby altering their properties .
Temporal Effects in Laboratory Settings
Hexadecyltriethoxysilane has been observed to form stable dispersions of metal oxide nanoparticles in hydrocarbon solvents . Over time, these organosilane-coated nanoparticles can yield silica-coated core-shell type nanoparticles when heated in air .
Vorbereitungsmethoden
Synthetic Routes and Reaction Conditions: Hexadecyltriethoxysilane can be synthesized through the hydrolysis and condensation of tetraethyl orthosilicate (TEOS) in the presence of hexadecyltrimethoxysilane. The reaction typically involves the following steps:
- Hydrolysis of TEOS to form silica nanoparticles.
- Addition of hexadecyltrimethoxysilane to allow the condensation reaction between the hydroxyl groups of hexadecyltrimethoxysilane and silica, rendering the silica particles hydrophobic .
Industrial Production Methods: In industrial settings, hexadecyltriethoxysilane is produced by refluxing the nanoparticles and the organosilane in a hydrocarbon solvent. This method ensures the formation of stable dispersions of metal oxide nanoparticles in hydrocarbon solvents due to their hydrophobic surface .
Analyse Chemischer Reaktionen
Types of Reactions: Hexadecyltriethoxysilane primarily undergoes condensation reactions. It can also participate in surface modification reactions where it reacts with hydroxyl groups on surfaces to form hydrophobic layers.
Common Reagents and Conditions:
Reagents: Tetraethyl orthosilicate, hexadecyltrimethoxysilane, hydrocarbon solvents.
Conditions: Refluxing in hydrocarbon solvents, hydrothermal-assisted sol-gel process
Major Products:
- Hydrophobic silica nanoparticles.
- Organosilane-coated metal oxide nanoparticles .
Vergleich Mit ähnlichen Verbindungen
- Hexadecyltrimethoxysilane
- Octadecyltriethoxysilane
- Dodecyltriethoxysilane
Comparison: Hexadecyltriethoxysilane is unique due to its specific chain length and the presence of three ethoxy groups, which provide superior hydrophobicity compared to other similar compounds. For instance, hexadecyltrimethoxysilane has methoxy groups instead of ethoxy groups, which may affect its reactivity and hydrophobic properties .
Eigenschaften
IUPAC Name |
triethoxy(hexadecyl)silane | |
---|---|---|
Source | PubChem | |
URL | https://pubchem.ncbi.nlm.nih.gov | |
Description | Data deposited in or computed by PubChem | |
InChI |
InChI=1S/C22H48O3Si/c1-5-9-10-11-12-13-14-15-16-17-18-19-20-21-22-26(23-6-2,24-7-3)25-8-4/h5-22H2,1-4H3 | |
Source | PubChem | |
URL | https://pubchem.ncbi.nlm.nih.gov | |
Description | Data deposited in or computed by PubChem | |
InChI Key |
OYGYKEULCAINCL-UHFFFAOYSA-N | |
Source | PubChem | |
URL | https://pubchem.ncbi.nlm.nih.gov | |
Description | Data deposited in or computed by PubChem | |
Canonical SMILES |
CCCCCCCCCCCCCCCC[Si](OCC)(OCC)OCC | |
Source | PubChem | |
URL | https://pubchem.ncbi.nlm.nih.gov | |
Description | Data deposited in or computed by PubChem | |
Molecular Formula |
C22H48O3Si | |
Source | PubChem | |
URL | https://pubchem.ncbi.nlm.nih.gov | |
Description | Data deposited in or computed by PubChem | |
DSSTOX Substance ID |
DTXSID60936917 | |
Record name | Triethoxy(hexadecyl)silane | |
Source | EPA DSSTox | |
URL | https://comptox.epa.gov/dashboard/DTXSID60936917 | |
Description | DSSTox provides a high quality public chemistry resource for supporting improved predictive toxicology. | |
Molecular Weight |
388.7 g/mol | |
Source | PubChem | |
URL | https://pubchem.ncbi.nlm.nih.gov | |
Description | Data deposited in or computed by PubChem | |
CAS No. |
16415-13-7 | |
Record name | Hexadecyltriethoxysilane | |
Source | CAS Common Chemistry | |
URL | https://commonchemistry.cas.org/detail?cas_rn=16415-13-7 | |
Description | CAS Common Chemistry is an open community resource for accessing chemical information. Nearly 500,000 chemical substances from CAS REGISTRY cover areas of community interest, including common and frequently regulated chemicals, and those relevant to high school and undergraduate chemistry classes. This chemical information, curated by our expert scientists, is provided in alignment with our mission as a division of the American Chemical Society. | |
Explanation | The data from CAS Common Chemistry is provided under a CC-BY-NC 4.0 license, unless otherwise stated. | |
Record name | Triethoxyhexadecylsilane | |
Source | ChemIDplus | |
URL | https://pubchem.ncbi.nlm.nih.gov/substance/?source=chemidplus&sourceid=0016415137 | |
Description | ChemIDplus is a free, web search system that provides access to the structure and nomenclature authority files used for the identification of chemical substances cited in National Library of Medicine (NLM) databases, including the TOXNET system. | |
Record name | Triethoxy(hexadecyl)silane | |
Source | EPA DSSTox | |
URL | https://comptox.epa.gov/dashboard/DTXSID60936917 | |
Description | DSSTox provides a high quality public chemistry resource for supporting improved predictive toxicology. | |
Record name | Triethoxyhexadecylsilane | |
Source | European Chemicals Agency (ECHA) | |
URL | https://echa.europa.eu/substance-information/-/substanceinfo/100.036.771 | |
Description | The European Chemicals Agency (ECHA) is an agency of the European Union which is the driving force among regulatory authorities in implementing the EU's groundbreaking chemicals legislation for the benefit of human health and the environment as well as for innovation and competitiveness. | |
Explanation | Use of the information, documents and data from the ECHA website is subject to the terms and conditions of this Legal Notice, and subject to other binding limitations provided for under applicable law, the information, documents and data made available on the ECHA website may be reproduced, distributed and/or used, totally or in part, for non-commercial purposes provided that ECHA is acknowledged as the source: "Source: European Chemicals Agency, http://echa.europa.eu/". Such acknowledgement must be included in each copy of the material. ECHA permits and encourages organisations and individuals to create links to the ECHA website under the following cumulative conditions: Links can only be made to webpages that provide a link to the Legal Notice page. | |
Retrosynthesis Analysis
AI-Powered Synthesis Planning: Our tool employs the Template_relevance Pistachio, Template_relevance Bkms_metabolic, Template_relevance Pistachio_ringbreaker, Template_relevance Reaxys, Template_relevance Reaxys_biocatalysis model, leveraging a vast database of chemical reactions to predict feasible synthetic routes.
One-Step Synthesis Focus: Specifically designed for one-step synthesis, it provides concise and direct routes for your target compounds, streamlining the synthesis process.
Accurate Predictions: Utilizing the extensive PISTACHIO, BKMS_METABOLIC, PISTACHIO_RINGBREAKER, REAXYS, REAXYS_BIOCATALYSIS database, our tool offers high-accuracy predictions, reflecting the latest in chemical research and data.
Strategy Settings
Precursor scoring | Relevance Heuristic |
---|---|
Min. plausibility | 0.01 |
Model | Template_relevance |
Template Set | Pistachio/Bkms_metabolic/Pistachio_ringbreaker/Reaxys/Reaxys_biocatalysis |
Top-N result to add to graph | 6 |
Feasible Synthetic Routes
Q1: What are the typical applications of Hexadecyltriethoxysilane and how does its structure facilitate these applications?
A1: Hexadecyltriethoxysilane (HDTMS) is frequently employed in creating superhydrophobic surfaces. [, ] This application stems from its ability to self-assemble into ordered monolayers with low surface energy. The molecule comprises a long hydrophobic alkyl chain (hexadecyl) and a hydrophilic triethoxysilane headgroup. The headgroup readily hydrolyzes and condenses to form siloxane bonds with hydroxyl groups present on various substrates, anchoring the molecule to the surface. [] The extended hydrophobic alkyl chain then orients outwards, repelling water and leading to superhydrophobicity.
Q2: What is the role of Hexadecyltriethoxysilane in nanoparticle dispersion and how does this relate to its chemical properties?
A2: Hexadecyltriethoxysilane acts as a surface modifier for metal oxide nanoparticles, enhancing their dispersion in nonpolar solvents. [] This function is attributed to its ability to graft onto the nanoparticle surface via the reactive triethoxysilane group, effectively replacing the polar surface groups with the long hydrophobic alkyl chains. This substitution reduces the surface energy of the nanoparticles, promoting their dispersion and stability in nonpolar media.
Q3: Can you elaborate on the specific experimental conditions optimized for Hexadecyltriethoxysilane synthesis and the reported yield?
A3: The synthesis of Hexadecyltriethoxysilane involves a hydrosilylation reaction between hexadecene and triethoxysilane. Research indicates that employing a Platinum catalyst at a temperature of 120℃, with a molar ratio of triethoxysilane to hexadecane of 1.1:1, leads to an optimized yield of 82.5%. []
Q4: How does the incorporation of Hexadecyltriethoxysilane affect the stability and durability of superhydrophobic coatings?
A4: Incorporating Hexadecyltriethoxysilane into superhydrophobic coatings significantly enhances their durability. Studies demonstrate that polymerized organosilane/attapulgite nanocomposites containing Hexadecyltriethoxysilane exhibited remarkable resistance to various harsh conditions, including sand abrasion, water pressure, extreme temperatures, UV irradiation, and solvent immersion. [] This robustness is attributed to the strong covalent bonding of the silane molecules to the substrate and the inherent stability of the polymerized siloxane network.
Haftungsausschluss und Informationen zu In-Vitro-Forschungsprodukten
Bitte beachten Sie, dass alle Artikel und Produktinformationen, die auf BenchChem präsentiert werden, ausschließlich zu Informationszwecken bestimmt sind. Die auf BenchChem zum Kauf angebotenen Produkte sind speziell für In-vitro-Studien konzipiert, die außerhalb lebender Organismen durchgeführt werden. In-vitro-Studien, abgeleitet von dem lateinischen Begriff "in Glas", beinhalten Experimente, die in kontrollierten Laborumgebungen unter Verwendung von Zellen oder Geweben durchgeführt werden. Es ist wichtig zu beachten, dass diese Produkte nicht als Arzneimittel oder Medikamente eingestuft sind und keine Zulassung der FDA für die Vorbeugung, Behandlung oder Heilung von medizinischen Zuständen, Beschwerden oder Krankheiten erhalten haben. Wir müssen betonen, dass jede Form der körperlichen Einführung dieser Produkte in Menschen oder Tiere gesetzlich strikt untersagt ist. Es ist unerlässlich, sich an diese Richtlinien zu halten, um die Einhaltung rechtlicher und ethischer Standards in Forschung und Experiment zu gewährleisten.