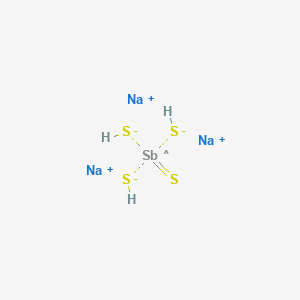
Sodium thioantimonate
- Klicken Sie auf QUICK INQUIRY, um ein Angebot von unserem Expertenteam zu erhalten.
- Mit qualitativ hochwertigen Produkten zu einem WETTBEWERBSFÄHIGEN Preis können Sie sich mehr auf Ihre Forschung konzentrieren.
Übersicht
Beschreibung
Sodium thioantimonate, also known as sodium tetrathioantimonate (V), is an inorganic compound with the chemical formula Na₃SbS₄. The nonahydrate form of this compound, Na₃SbS₄·9H₂O, is commonly referred to as Schlippe’s salt, named after Johann Karl Friedrich von Schlippe. This compound is a member of the sulfosalt family and is characterized by its yellow crystalline appearance .
Vorbereitungsmethoden
Synthetic Routes and Reaction Conditions: Sodium thioantimonate nonahydrate can be synthesized through the reaction of antimony trisulfide, elemental sulfur, and an aqueous sulfide source. The reaction is as follows: [ 3 \text{Na}_2\text{S} + 2 \text{S} + \text{Sb}_2\text{S}_3 + 18 \text{H}_2\text{O} \rightarrow 2 \text{Na}_3\text{SbS}_4·9\text{H}_2\text{O} ]
Alternatively, sodium sulfide can be generated in situ by reacting sodium hydroxide with sulfur, which co-generates sodium sulfate: [ \text{Sb}_2\text{S}_3 + 8 \text{NaOH} + 6 \text{S} \rightarrow 2 \text{Na}_3\text{SbS}_4 + \text{Na}_2\text{SO}_4 + 4 \text{H}_2\text{O} ]
Industrial Production Methods: High-energy ball milling is an innovative method for synthesizing high-purity this compound nonahydrate. This method allows for a yield of approximately 90% in around four hours .
Types of Reactions:
Dissolution: this compound nonahydrate dissolves in water to form the tetrahedral SbS₄³⁻ ion.
Acidification: Upon acidification, this compound yields antimony pentasulfide: [ 2 \text{Na}_3\text{SbS}_4 + 6 \text{HCl} \rightarrow \text{Sb}_2\text{S}_5 + 6 \text{NaCl} + 3 \text{H}_2\text{S} ]
Common Reagents and Conditions:
Reagents: Hydrochloric acid, sodium hydroxide, sulfur, antimony trisulfide.
Conditions: Aqueous solutions, controlled temperatures, and specific pH levels.
Major Products:
- Antimony pentasulfide (Sb₂S₅)
- Sodium chloride (NaCl)
- Hydrogen sulfide (H₂S)
Wissenschaftliche Forschungsanwendungen
Sodium thioantimonate has several applications in scientific research:
- Chemistry: Used as a precursor for synthesizing hybrid compounds and as a reagent in qualitative inorganic analysis .
- Biology and Medicine: Potential applications in developing sodium-ion conductors for solid-state batteries due to its high electrochemical stability .
- Industry: Utilized in the production of high-performance lithium-ion batteries and as a safer alternative to flammable organic liquid electrolytes .
Wirkmechanismus
The exact mechanism of action of sodium thioantimonate is not fully understood. it is believed that antimony, a key component, interacts with sulfhydryl groups in enzymes crucial for tissue respiration. This interaction may inhibit enzyme activity, leading to various biochemical effects .
Vergleich Mit ähnlichen Verbindungen
- Potassium thioantimonate (K₃SbS₄)
- Ammonium thioantimonate ((NH₄)₃SbS₄)
- Antimony (III) sulfide (Sb₂S₃)
Comparison: Sodium thioantimonate is unique due to its high solubility in water and its ability to form stable nonahydrate crystals. Compared to potassium and ammonium thioantimonates, this compound is more commonly used in industrial applications due to its ease of synthesis and high yield .
Biologische Aktivität
Sodium thioantimonate (Na₃SbS₄), also known as Schlippe's salt, is an inorganic compound that has garnered attention for its biological activity, particularly in the context of its potential applications in medicine and biochemistry. This article explores its biological mechanisms, effects on cellular systems, and relevant case studies.
Chemical Structure and Properties
This compound is characterized by its yellow crystalline appearance and is a member of the sulfosalt family. It exhibits high solubility in water, which facilitates its use in various biological applications. The compound's chemical formula is Na₃SbS₄, and it can exist in a hydrated form (Na₃SbS₄·9H₂O) .
The exact mechanism of action of this compound is not fully elucidated; however, it is believed to interact with sulfhydryl groups in enzymes that are critical for cellular respiration. This interaction may inhibit enzyme activity, leading to various biochemical effects. Specifically, antimony ions can disrupt metabolic processes by binding to thiol groups, which are essential for the function of many enzymes .
Biological Activity
Research has indicated several biological activities associated with this compound:
- Antimicrobial Activity : this compound has shown potential antimicrobial properties against various pathogens. Its ability to disrupt cellular processes makes it a candidate for further investigation as an antimicrobial agent.
- Cytotoxic Effects : Studies have reported cytotoxic effects on certain cancer cell lines. The compound's mechanism may involve inducing oxidative stress or apoptosis in these cells .
- Enzyme Inhibition : The interaction with sulfhydryl groups suggests that this compound could inhibit enzymes involved in critical metabolic pathways, potentially leading to therapeutic applications in conditions where enzyme inhibition is beneficial .
Case Studies
- Antimicrobial Studies : A study demonstrated that this compound exhibited significant antibacterial activity against Staphylococcus aureus and Escherichia coli. The minimum inhibitory concentration (MIC) was determined to be lower than that of conventional antibiotics, suggesting its potential as an alternative treatment option .
- Cytotoxicity in Cancer Research : In vitro studies on human cancer cell lines revealed that this compound induced apoptosis through the activation of caspase pathways. This finding highlights its potential as a chemotherapeutic agent .
- Enzyme Interaction Studies : Research focusing on the inhibition of key metabolic enzymes demonstrated that this compound effectively inhibited lactate dehydrogenase activity, suggesting a possible mechanism for its cytotoxic effects .
Comparative Analysis
To better understand the biological activity of this compound, a comparison with similar compounds can be beneficial.
Compound | Solubility | Antimicrobial Activity | Cytotoxicity |
---|---|---|---|
This compound | High | Yes | Yes |
Potassium Thioantimonate | Moderate | Limited | Limited |
Ammonium Thioantimonate | Low | No | No |
This table illustrates that this compound stands out due to its high solubility and notable biological activities compared to other thioantimonates.
Eigenschaften
InChI |
InChI=1S/3Na.3H2S.S.Sb/h;;;3*1H2;;/q3*+1;;;;;/p-3 |
Source
|
---|---|---|
Source | PubChem | |
URL | https://pubchem.ncbi.nlm.nih.gov | |
Description | Data deposited in or computed by PubChem | |
InChI Key |
FBZSTSSPKIVXEO-UHFFFAOYSA-K |
Source
|
Source | PubChem | |
URL | https://pubchem.ncbi.nlm.nih.gov | |
Description | Data deposited in or computed by PubChem | |
Canonical SMILES |
[Na+].[Na+].[Na+].[SH-].[SH-].[SH-].S=[Sb] |
Source
|
Source | PubChem | |
URL | https://pubchem.ncbi.nlm.nih.gov | |
Description | Data deposited in or computed by PubChem | |
Molecular Formula |
H3Na3S4Sb |
Source
|
Source | PubChem | |
URL | https://pubchem.ncbi.nlm.nih.gov | |
Description | Data deposited in or computed by PubChem | |
Molecular Weight |
322.0 g/mol |
Source
|
Source | PubChem | |
URL | https://pubchem.ncbi.nlm.nih.gov | |
Description | Data deposited in or computed by PubChem | |
CAS No. |
13776-84-6 |
Source
|
Record name | Antimonate(3-), tetrathioxo-, sodium (1:3), (T-4)- | |
Source | EPA Chemicals under the TSCA | |
URL | https://www.epa.gov/chemicals-under-tsca | |
Description | EPA Chemicals under the Toxic Substances Control Act (TSCA) collection contains information on chemicals and their regulations under TSCA, including non-confidential content from the TSCA Chemical Substance Inventory and Chemical Data Reporting. | |
Record name | Trisodium tetrathioantimonate | |
Source | European Chemicals Agency (ECHA) | |
URL | https://echa.europa.eu/substance-information/-/substanceinfo/100.033.999 | |
Description | The European Chemicals Agency (ECHA) is an agency of the European Union which is the driving force among regulatory authorities in implementing the EU's groundbreaking chemicals legislation for the benefit of human health and the environment as well as for innovation and competitiveness. | |
Explanation | Use of the information, documents and data from the ECHA website is subject to the terms and conditions of this Legal Notice, and subject to other binding limitations provided for under applicable law, the information, documents and data made available on the ECHA website may be reproduced, distributed and/or used, totally or in part, for non-commercial purposes provided that ECHA is acknowledged as the source: "Source: European Chemicals Agency, http://echa.europa.eu/". Such acknowledgement must be included in each copy of the material. ECHA permits and encourages organisations and individuals to create links to the ECHA website under the following cumulative conditions: Links can only be made to webpages that provide a link to the Legal Notice page. | |
Record name | SODIUM THIOANTIMONATE | |
Source | FDA Global Substance Registration System (GSRS) | |
URL | https://gsrs.ncats.nih.gov/ginas/app/beta/substances/W0I6VDX5GA | |
Description | The FDA Global Substance Registration System (GSRS) enables the efficient and accurate exchange of information on what substances are in regulated products. Instead of relying on names, which vary across regulatory domains, countries, and regions, the GSRS knowledge base makes it possible for substances to be defined by standardized, scientific descriptions. | |
Explanation | Unless otherwise noted, the contents of the FDA website (www.fda.gov), both text and graphics, are not copyrighted. They are in the public domain and may be republished, reprinted and otherwise used freely by anyone without the need to obtain permission from FDA. Credit to the U.S. Food and Drug Administration as the source is appreciated but not required. | |
Haftungsausschluss und Informationen zu In-Vitro-Forschungsprodukten
Bitte beachten Sie, dass alle Artikel und Produktinformationen, die auf BenchChem präsentiert werden, ausschließlich zu Informationszwecken bestimmt sind. Die auf BenchChem zum Kauf angebotenen Produkte sind speziell für In-vitro-Studien konzipiert, die außerhalb lebender Organismen durchgeführt werden. In-vitro-Studien, abgeleitet von dem lateinischen Begriff "in Glas", beinhalten Experimente, die in kontrollierten Laborumgebungen unter Verwendung von Zellen oder Geweben durchgeführt werden. Es ist wichtig zu beachten, dass diese Produkte nicht als Arzneimittel oder Medikamente eingestuft sind und keine Zulassung der FDA für die Vorbeugung, Behandlung oder Heilung von medizinischen Zuständen, Beschwerden oder Krankheiten erhalten haben. Wir müssen betonen, dass jede Form der körperlichen Einführung dieser Produkte in Menschen oder Tiere gesetzlich strikt untersagt ist. Es ist unerlässlich, sich an diese Richtlinien zu halten, um die Einhaltung rechtlicher und ethischer Standards in Forschung und Experiment zu gewährleisten.