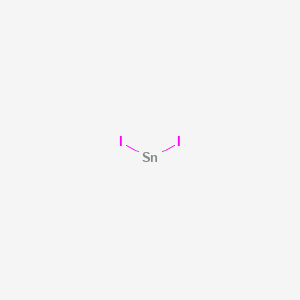
Tin(II) iodide
Übersicht
Beschreibung
Tin(II) iodide (SnI₂) is a red-orange crystalline solid with a density of 7.6 g/cm³ and a melting point of 320°C . It is a critical precursor for tin-based halide perovskites, such as methylammonium tin iodide (CH₃NH₃SnI₃), which are lead-free alternatives in photovoltaic applications . SnI₂ exhibits a direct bandgap of ~1.12 eV (theoretical, PBE functional) , closely matching experimental values (~1.2 eV) . Its orthorhombic structure consists of SnI₆ octahedra, with iodine 5p orbitals dominating the valence band and tin 5p orbitals contributing to the conduction band .
Thermodynamically, SnI₂-based perovskites have a formation enthalpy (ΔfH°) of -390 kJ/mol at 0 K, indicating stability at low temperatures . However, Sn(II) is prone to oxidation to Sn(IV) in the presence of moisture and oxygen, forming detrimental iodine species (e.g., I₂) that accelerate decomposition .
Vorbereitungsmethoden
Traditional Synthesis Methods
Direct Elemental Combination
The direct reaction between metallic tin and iodine remains the foundational method for SnI₂ synthesis. This approach involves heating tin powder with iodine in an inert atmosphere to prevent oxidation. The stoichiometric reaction proceeds as:
Key parameters include temperature control (typically 320–400°C) and reaction duration (12–24 hours) . While this method yields SnI₂ with high purity, challenges such as incomplete reactant conversion and oxidation of Sn²⁺ to Sn⁴⁺ persist. Recent modifications involve using tin nanoparticles to increase surface area, accelerating the reaction kinetics .
Solution-Based Synthesis
Alternative methods employ hydroiodic acid (HI) as both a solvent and reactant. Tin oxide (SnO) reacts with HI under reflux conditions:
This method avoids high-temperature processing but requires rigorous moisture control due to SnI₂’s hygroscopic nature . Solubility in polar aprotic solvents like dimethyl sulfoxide (DMSO) facilitates ink formulation for thin-film deposition, though residual solvents can compromise device performance .
Carboxylic Acid-Assisted Synthesis (CAAS)
Methodology and Reaction Mechanism
The CAAS method, pioneered in 2024, introduces carboxylic acid ligands to functionalize tin nanoparticles in situ. Tin powder is treated with formic acid or related derivatives, forming a tin-carboxylic acid complex that reacts with iodine in DMSO . The reaction pathway involves:
-
Coordination : Carboxylic acid ligands (e.g., formate) adsorb onto tin surfaces via dipole or pincer interactions (Figure 1a).
-
Oxidative Addition : Iodine molecules bind to the tin-carboxylate complex, facilitating the formation of SnI₂.
-
Regeneration : The carboxylic acid is released, enabling catalytic cycles that enhance reaction efficiency .
This method reduces particle size to 10–50 nm, increasing the surface-area-to-volume ratio and accelerating synthesis .
Role of Carboxylic Acid Ligands
Carboxylic acids serve dual roles:
-
Stabilization : Ligands prevent nanoparticle aggregation and shield Sn²⁺ from oxidation.
-
Crystallization Control : During perovskite formation, carboxylate groups modulate crystal growth, reducing defects .
119Sn MAS NMR confirmed the absence of Sn⁴⁺ in CAAS-derived SnI₂, highlighting its superiority over traditional methods .
Optimization of Reaction Conditions
Key parameters for CAAS include:
-
Ligand Selection : Formic acid outperforms bulkier derivatives due to its small size and strong coordination.
-
Solvent System : DMSO enhances iodine solubility but requires inert atmospheres. Recent adaptations enable synthesis in air using thiourea-based antioxidants .
-
Temperature : Reactions proceed at 80–100°C, completing within 2–4 hours .
Comparative Analysis of Preparation Methods
Method | Reactants | Conditions | Yield | Purity | Applications |
---|---|---|---|---|---|
Direct Elemental | Sn, I₂ | 320°C, inert atmosphere | 85–90% | 95% | Bulk material synthesis |
Solution-Based | SnO, HI | Reflux, moisture-controlled | 75–80% | 90% | Thin-film deposition |
CAAS | Sn, I₂, carboxylic acid | 80–100°C, DMSO | 95–98% | >99% | High-efficiency TPSCs |
The CAAS method achieves near-quantitative yields and exceptional purity, making it ideal for optoelectronic applications .
Applications in Photovoltaic Devices
SnI₂’s role in TPSCs hinges on its optical bandgap (~1.3 eV) and carrier mobility. CAAS-derived SnI₂ enables flexible devices with:
Wissenschaftliche Forschungsanwendungen
Tin(II) iodide is a versatile compound with a range of applications in scientific research:
Chemistry: Used as a precursor in the synthesis of tin-based perovskites for solar cells. .
Biology: Employed in the preparation of organic-inorganic hybrid materials with potential biological applications.
Medicine: Investigated for its potential use in medical imaging and as an analytical reagent.
Industry: Utilized in semiconductor technology, photonics, and the preparation of thin films for electronic and optoelectronic devices
Wirkmechanismus
The mechanism by which tin(II) iodide exerts its effects involves its reducing properties and ability to form complexes with various ligands. In solar cells, it acts as a precursor for tin-based perovskites, enhancing the stability and performance of the devices. The molecular targets and pathways involved include the formation of stable tin-iodide bonds and the interaction with other components in the perovskite structure .
Vergleich Mit ähnlichen Verbindungen
Comparison with Lead-Based Iodides (PbI₂ and CH₃NH₃PbI₃)
Structural and Electronic Properties
- Bandgap : CH₃NH₃SnI₃ has a narrower bandgap (~1.2 eV) compared to CH₃NH₃PbI₃ (~1.5 eV), making it suitable for near-infrared applications but less efficient in visible-light absorption .
- Formation Enthalpy : The formation enthalpy of CH₃NH₃SnI₃ (-390 kJ/mol) is 24 kJ/mol less stable than CH₃NH₃PbI₃ (-414 kJ/mol), reflecting the higher stability of Pb-based perovskites .
- Decomposition : Sn-based perovskites decompose with ΔrH° = 37 kJ/mol, whereas Pb-based perovskites have a lower decomposition enthalpy (34.5 kJ/mol) but greater thermodynamic stability due to stronger Pb-I bonding .
Stability and Environmental Impact
- Oxidation Sensitivity: Sn(II) in CH₃NH₃SnI₃ oxidizes readily to Sn(IV), forming conductive SnO₂ and iodine byproducts (e.g., HI, I₂), which degrade device performance . Pb(II) is less susceptible to oxidation, contributing to the superior stability of Pb-based perovskites .
- Toxicity: Pb-based compounds pose significant environmental and health risks, driving research into SnI₂ as a non-toxic alternative .
Table 1: Comparison of SnI₂ and PbI₂-Based Perovskites
Property | CH₃NH₃SnI₃ | CH₃NH₃PbI₃ |
---|---|---|
Bandgap (eV) | 1.12–1.2 | 1.5–1.6 |
Formation Enthalpy (kJ/mol) | -390 | -414 |
Decomposition Enthalpy (kJ/mol) | 37 | 34.5 |
Oxidation Stability | Low | High |
Toxicity | Low | High |
Comparison with Other Tin Halides (SnF₂, SnCl₂)
- SnF₂ : Used as an additive in Sn-based perovskites to suppress Sn(II) oxidation. Fluoride ions coordinate with Sn, stabilizing the +2 oxidation state and reducing defect density . SnF₂-modified perovskites show improved carrier lifetimes and device efficiency .
- SnCl₂ : Less common in perovskites due to weaker Sn-Cl bonds compared to Sn-I. SnCl₂-based materials exhibit wider bandgaps (~2.2–3.0 eV), limiting their photovoltaic utility .
Comparison with Inorganic Tin Iodides (CsSnI₃)
Cesium tin iodide (CsSnI₃) is an inorganic perovskite with a bandgap of ~1.3 eV and high hole mobility (>300 cm²/V·s) . Unlike hybrid perovskites (e.g., CH₃NH₃SnI₃), CsSnI₃ lacks organic cations, enhancing thermal stability but requiring high-temperature synthesis . Both materials suffer from Sn(II) oxidation, but CsSnI₃ exhibits better phase stability under humidity .
Stability and Environmental Factors
SnI₂-based materials degrade via a cyclic process:
Oxidation : Sn²⁺ → Sn⁴⁺ + 2e⁻
Iodine Release : 2I⁻ → I₂ + 2e⁻
Accelerated Decomposition : I₂ further oxidizes Sn²⁺, perpetuating degradation .
Strategies to mitigate this include:
Biologische Aktivität
Tin(II) iodide (SnI) is an inorganic compound that has garnered interest for its unique properties and potential applications in various fields, including photovoltaics and biomedicine. This article explores the biological activity of this compound, focusing on its interactions with biological systems, potential therapeutic applications, and stability characteristics.
This compound is a layered material that can exist in both bulk and nanoscale forms. Its structure allows for significant interactions with biological molecules, making it a candidate for various applications. The compound has a molecular weight of approximately 392.2 g/mol and exhibits distinctive optical properties, which are crucial for its applications in photonics and solar energy conversion .
Research indicates that this compound interacts with several biological targets, particularly enzymes involved in metabolic processes. Notably, it has been shown to inhibit alcohol dehydrogenase (ADH), an enzyme critical for ethanol metabolism. This inhibition suggests a potential role in modulating metabolic pathways related to alcohol consumption .
Target Enzymes:
- Alcohol Dehydrogenase 1A (ADH1A) : Involved in the oxidation of alcohols.
- Alcohol Dehydrogenase 1B (ADH1B) : Plays a role in the metabolism of ethanol.
- Alcohol Dehydrogenase 1C (ADH1C) : Another isoform contributing to alcohol metabolism.
Enzyme Name | Gene Name | Uniprot ID | General Function |
---|---|---|---|
Alcohol Dehydrogenase 1A | ADH1A | P07327 | Zinc ion binding |
Alcohol Dehydrogenase 1B | ADH1B | P00325 | Zinc ion binding |
Alcohol Dehydrogenase 1C | ADH1C | P00326 | Zinc ion binding |
This inhibition may have implications for developing treatments for alcohol-related disorders, although further research is required to elucidate the full scope of its effects .
Cytotoxicity Studies
Studies have evaluated the cytotoxic effects of this compound on various cell lines. The compound exhibits dose-dependent cytotoxicity, which is critical for assessing its safety profile in potential therapeutic applications. For instance, this compound has been shown to induce apoptosis in cancer cell lines at specific concentrations while demonstrating lower toxicity to normal cells .
Photovoltaic Applications
This compound has been extensively studied in the context of solar energy conversion. Hybrid organic-inorganic perovskites based on this compound have shown promising results in photovoltaic efficiency while maintaining lower toxicity compared to lead-based counterparts. These materials display enhanced stability under ambient conditions, making them suitable for sustainable energy solutions .
Stability and Environmental Impact
The stability of this compound under various environmental conditions is crucial for its application in biomedicine and photovoltaics. Research indicates that this compound-based materials exhibit significant resistance to oxidation and degradation when exposed to air and moisture, which enhances their viability as long-term solutions in solar energy applications .
Analyse Chemischer Reaktionen
Oxidation Reactions
SnI₂ acts as a reducing agent due to the oxidation state of Sn(II):
Oxidation by Dimethylsulfoxide (DMSO)
In acidic, heated conditions (e.g., perovskite precursor solutions), DMSO oxidizes SnI₂ to Sn(IV) species:
-
Mechanism : Proton-assisted oxidation forms iododimethylsulfonium iodide intermediates .
-
Impact : This reaction destabilizes tin halide perovskites, necessitating antioxidant additives .
Oxidation by Nitric Acid
Concentrated HNO₃ oxidizes SnI₂ to metastannic acid (H₂SnO₃):
Hydrolysis and Aqueous Reactivity
SnI₂ undergoes hydrolysis in water, influenced by pH:
-
Acidic conditions : Hydrolysis is suppressed; Sn²⁺ remains stable in 1–3 M HCl .
-
Neutral/basic conditions : Forms insoluble Sn(OH)₂ precipitates .
With Halides
SnI₂ forms complexes in concentrated halide solutions:
Reagent | Product | Conditions |
---|---|---|
Aqueous NH₃ | Sn(OH)₂ (white) | pH > 7 |
NaOH | [Sn(OH)₄]^{2-} | Excess OH⁻ |
H₂S (mildly acidic) | SnS (brown) | pH 2–4 |
With Reducing Agents
Metallic Fe or Al reduces Sn(IV) impurities to Sn(II) in SnI₂ solutions:
Thermodynamic and Stability Data
Property | Value | Source |
---|---|---|
Melting point | 320°C | |
Solubility in H₂O | 0.18 g/100 mL (20°C) | |
ΔfH° (crystalline) | −137.6 kJ/mol | |
Stability in air | Oxidizes slowly; store under inert gas |
Q & A
Basic Research Questions
Q. What experimental methods are used to determine the empirical formula of Tin(II) iodide (SnI₂)?
- Methodological Answer : A classic approach involves reacting elemental tin with iodine under controlled conditions. The mass of reactants and products is measured to calculate the molar ratio of Sn to I. For instance, excess iodine is reacted with tin in a sealed tube, followed by gravimetric analysis of the product. Data inconsistencies (e.g., deviations from the expected 1:2 Sn:I ratio) should be resolved using error propagation analysis and repeating trials under inert atmospheres to avoid oxidation .
Q. How can researchers safely handle this compound in laboratory settings?
- Methodological Answer : SnI₂ is moisture-sensitive and can decompose into toxic hydrogen iodide. Use inert gloveboxes for synthesis/storage, and employ fume hoods during handling. Safety protocols include:
- Pre-experiment risk assessments for iodine vapor exposure.
- Neutralization of waste with sodium thiosulfate.
- Ethical documentation of hazards in line with IB Chemistry IA guidelines for safe experimentation .
Q. What are the foundational steps to formulate a research question on SnI₂’s properties?
- Methodological Answer : Use frameworks like PICO(T) (Population, Intervention, Comparison, Outcome, Time) to structure inquiries. Example: “How does the solvent polarity (Intervention) affect the crystallinity (Outcome) of SnI₂ synthesized via solvothermal methods (Population)?” Refine questions iteratively by reviewing literature on SnI₂’s phase transitions or optoelectronic behavior .
Advanced Research Questions
Q. How can researchers resolve contradictions in reported bandgap values of SnI₂?
- Methodological Answer : Discrepancies may arise from synthesis methods (e.g., solution-processed vs. vapor-deposited SnI₂) or characterization techniques (UV-Vis vs. photoluminescence spectroscopy). Mitigate by:
- Standardizing sample preparation (e.g., annealing conditions).
- Cross-validating results with multiple techniques (e.g., Tauc plot analysis combined with DFT calculations).
- Reporting instrumental uncertainties (e.g., ±0.05 eV for UV-Vis) .
Q. What strategies optimize SnI₂ synthesis for perovskite precursor applications?
- Methodological Answer : To enhance purity and yield:
- Use high-purity precursors (≥99.99%) in stoichiometric ratios.
- Employ mechanochemical synthesis (ball milling) for homogeneity.
- Characterize intermediates via XRD and Raman spectroscopy to track phase evolution. Compare with lead-based perovskites (e.g., CH₃NH₃PbI₃) to identify SnI₂’s stability limitations .
Q. How can computational methods guide the design of SnI₂-based photovoltaic devices?
- Methodological Answer : Density Functional Theory (DFT) predicts electronic structures, while molecular dynamics simulations model degradation pathways. Key steps:
- Validate computational models against experimental XRD and bandgap data.
- Screen dopants (e.g., Sb³⁺) to improve charge carrier lifetimes.
- Collaborate with experimentalists to iteratively refine synthetic protocols .
Q. What methodologies ensure reproducibility in SnI₂ thin-film fabrication?
- Methodological Answer : Standardize protocols using:
- Spin-coating parameters (rpm, acceleration) with humidity control (<5% RH).
- In situ grazing-incidence XRD to monitor crystallinity.
- Detailed reporting of precursor concentrations and substrate treatments per Beilstein Journal guidelines .
Q. How do researchers evaluate SnI₂’s stability under operational conditions in solar cells?
- Methodological Answer : Conduct accelerated aging tests:
- Expose devices to 85°C/85% RH while monitoring efficiency decay via J-V curves.
- Use FTIR to detect iodide loss or oxide formation.
- Compare degradation kinetics with CH₃NH₃PbI₃ to contextualize SnI₂’s viability .
Q. Tables for Key Data
Property | SnI₂ | CH₃NH₃PbI₃ | Method |
---|---|---|---|
Bandgap (eV) | 1.3–1.6 | 1.5–1.6 | UV-Vis Tauc plot |
Decomposition Temperature | ~200°C | ~250°C | TGA |
Hole Mobility (cm²/Vs) | ~4.6 | ~24 | Hall effect |
Eigenschaften
IUPAC Name |
tin(2+);diiodide | |
---|---|---|
Details | Computed by LexiChem 2.6.6 (PubChem release 2019.06.18) | |
Source | PubChem | |
URL | https://pubchem.ncbi.nlm.nih.gov | |
Description | Data deposited in or computed by PubChem | |
InChI |
InChI=1S/2HI.Sn/h2*1H;/q;;+2/p-2 | |
Details | Computed by InChI 1.0.5 (PubChem release 2019.06.18) | |
Source | PubChem | |
URL | https://pubchem.ncbi.nlm.nih.gov | |
Description | Data deposited in or computed by PubChem | |
InChI Key |
JTDNNCYXCFHBGG-UHFFFAOYSA-L | |
Details | Computed by InChI 1.0.5 (PubChem release 2019.06.18) | |
Source | PubChem | |
URL | https://pubchem.ncbi.nlm.nih.gov | |
Description | Data deposited in or computed by PubChem | |
Canonical SMILES |
[Sn+2].[I-].[I-] | |
Details | Computed by OEChem 2.1.5 (PubChem release 2019.06.18) | |
Source | PubChem | |
URL | https://pubchem.ncbi.nlm.nih.gov | |
Description | Data deposited in or computed by PubChem | |
Molecular Formula |
I2Sn | |
Details | Computed by PubChem 2.1 (PubChem release 2019.06.18) | |
Source | PubChem | |
URL | https://pubchem.ncbi.nlm.nih.gov | |
Description | Data deposited in or computed by PubChem | |
Molecular Weight |
372.52 g/mol | |
Details | Computed by PubChem 2.1 (PubChem release 2021.05.07) | |
Source | PubChem | |
URL | https://pubchem.ncbi.nlm.nih.gov | |
Description | Data deposited in or computed by PubChem | |
Haftungsausschluss und Informationen zu In-Vitro-Forschungsprodukten
Bitte beachten Sie, dass alle Artikel und Produktinformationen, die auf BenchChem präsentiert werden, ausschließlich zu Informationszwecken bestimmt sind. Die auf BenchChem zum Kauf angebotenen Produkte sind speziell für In-vitro-Studien konzipiert, die außerhalb lebender Organismen durchgeführt werden. In-vitro-Studien, abgeleitet von dem lateinischen Begriff "in Glas", beinhalten Experimente, die in kontrollierten Laborumgebungen unter Verwendung von Zellen oder Geweben durchgeführt werden. Es ist wichtig zu beachten, dass diese Produkte nicht als Arzneimittel oder Medikamente eingestuft sind und keine Zulassung der FDA für die Vorbeugung, Behandlung oder Heilung von medizinischen Zuständen, Beschwerden oder Krankheiten erhalten haben. Wir müssen betonen, dass jede Form der körperlichen Einführung dieser Produkte in Menschen oder Tiere gesetzlich strikt untersagt ist. Es ist unerlässlich, sich an diese Richtlinien zu halten, um die Einhaltung rechtlicher und ethischer Standards in Forschung und Experiment zu gewährleisten.