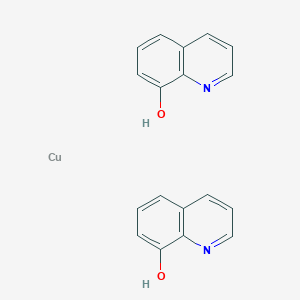
8-Hydroxychinolin-Kupfer(II)
Übersicht
Beschreibung
Oxine-copper, also known as copper oxinate, is a coordination compound with the chemical formula C₁₈H₁₂CuN₂O₂. It is a yellow-green crystalline powder that is insoluble in water but soluble in organic solvents. This compound is widely used in various fields due to its unique chemical properties and biological activities .
Wissenschaftliche Forschungsanwendungen
Oxine-copper has a wide range of scientific research applications, including:
Chemistry: Used as a reagent in various chemical reactions and as a catalyst in organic synthesis.
Biology: Employed in biological studies due to its antimicrobial and antifungal properties.
Medicine: Investigated for its potential therapeutic effects, including anticancer and neuroprotective activities.
Wirkmechanismus
Target of Action
Oxine-copper primarily targets pathogens . It is used as a fungicide and has been found to be effective against molds, mildews, sapstain fungi, termites, and wood-eating beetles .
Mode of Action
The mode of action of Oxine-copper involves the disruption of enzyme systems in pathogens . The compound is absorbed and the copper ions it contains disrupt these systems, leading to a multi-site activity . The compound’s action involves the generation of reactive oxygen species (ROS) by reduction of copper through a Fenton-like reaction, leading to enzyme and non-enzyme mediated oxidative damage involving lipid peroxidation, protein oxidation, and DNA damage . The final mechanism is the release of copper ions, Cu+ and Cu2+, which damage the membrane and infiltrate the cell, inducing an oxidative stress response involving endogenous ROS .
Biochemical Pathways
The biochemical pathways affected by Oxine-copper are multifaceted. The compound’s action leads to the generation of ROS, which irreversibly damages membranes . Copper ions released from surfaces lead to RNA degradation and membrane disruption of enveloped viruses . For fungi, the mechanism involves the physical deterioration of the membrane and copper ion influx .
Pharmacokinetics
It is known that oxine-copper dissociates under weak acidity and becomes water-soluble .
Result of Action
The result of Oxine-copper’s action is the inhibition of the growth of pathogens . The compound’s bactericidal activity is due to the generation of ROS, which irreversibly damages membranes . The release of copper ions leads to RNA degradation and membrane disruption of enveloped viruses . For fungi, the mechanism involves the physical deterioration of the membrane and copper ion influx .
Action Environment
The action of Oxine-copper can be influenced by environmental factors. For instance, Oxine-copper has been reported to become less fungistatic as the pH is lowered from neutral . The loss of activity is explained by an increase in cationic ionization, consequent loss of ability to chelate, and decreasing lipid solubility .
Safety and Hazards
Biochemische Analyse
Biochemical Properties
Oxine-copper plays a significant role in biochemical reactions. It interacts with various enzymes, proteins, and other biomolecules. The most widely held hypothesis on the mechanism of action of Oxine-copper holds that it is only active when it can form saturated chelates with metals in the medium which enter the cell and dissociate to liberate a toxic half-chelate .
Cellular Effects
Oxine-copper has been found to have significant effects on various types of cells and cellular processes. It influences cell function, including impacts on cell signaling pathways, gene expression, and cellular metabolism . For instance, Oxine-copper has been found to induce developmental toxicity and behavioral alterations by affecting AChE enzyme activity and oxidative stress .
Molecular Mechanism
Oxine-copper exerts its effects at the molecular level through various mechanisms. It forms more fungitoxic and soluble metal oxinates and antagonism by the formation of less soluble or less active metal oxinates . It also involves the formation of a copper(I)–peptide complex from cuprous oxide (Cu2O) and free radical generation from cupric oxide (CuO) which were identified as key sources of toxicity .
Temporal Effects in Laboratory Settings
The effects of Oxine-copper change over time in laboratory settings. Studies have shown that the half-lives of Oxine-copper in cucumber and watermelon were 1.77−2.11 and 3.57−4.68 days, respectively . This indicates the product’s stability and degradation over time.
Dosage Effects in Animal Models
The effects of Oxine-copper vary with different dosages in animal models. For instance, in zebrafish, Oxine-copper induced developmental defects and behavioral alterations at concentrations of 10, 20, and 40 μg/L .
Metabolic Pathways
Oxine-copper is involved in various metabolic pathways. It interacts with enzymes and cofactors, and can affect metabolic flux or metabolite levels. For instance, Oxine-copper rapidly inactivated Fumarase A, an iron sulphur cluster enzyme suggesting the cuprous state of copper binding to the proteins .
Subcellular Localization
The subcellular localization of Oxine-copper and its effects on activity or function are also areas of active research. Current studies suggest that Oxine-copper may localize to peroxisomes
Vorbereitungsmethoden
Synthetic Routes and Reaction Conditions
The preparation of copper 8-hydroxyquinolinate typically involves the reaction of 8-hydroxyquinoline with a copper salt. One common method includes heating quinoline with fuming sulfuric acid to obtain quinolyl-8-sulfonic acid, which is then hydrolyzed to produce 8-hydroxyquinoline. This 8-hydroxyquinoline is then reacted with anhydrous cupric sulfate in the presence of sodium hydroxide to form copper 8-hydroxyquinolinate .
Industrial Production Methods
In industrial settings, the production of copper 8-hydroxyquinolinate follows similar synthetic routes but on a larger scale. The process ensures high yield and purity, making the compound suitable for various high-end applications .
Analyse Chemischer Reaktionen
Types of Reactions
Oxine-copper undergoes several types of chemical reactions, including:
Oxidation: The compound can be oxidized under specific conditions.
Reduction: It can also be reduced, although this is less common.
Substitution: The compound can participate in substitution reactions where the 8-hydroxyquinoline ligand is replaced by other ligands.
Common Reagents and Conditions
Common reagents used in reactions with copper 8-hydroxyquinolinate include oxidizing agents, reducing agents, and various ligands for substitution reactions. The conditions for these reactions vary but often involve specific temperatures and solvents to facilitate the desired chemical transformations .
Major Products Formed
The major products formed from these reactions depend on the type of reaction and the reagents used. For example, oxidation may yield oxidized derivatives of the compound, while substitution reactions can produce a variety of copper complexes with different ligands .
Vergleich Mit ähnlichen Verbindungen
Oxine-copper is unique compared to other similar compounds due to its specific chemical structure and properties. Similar compounds include:
- Copper 8-quinolinolate
- Copper oxinate
- Copper hydroxyquinolate
- Copper oxyquinolate
These compounds share similar chelating properties but differ in their specific chemical structures and applications. Oxine-copper stands out for its high stability and effectiveness in various applications .
Eigenschaften
{ "Design of the Synthesis Pathway": "The synthesis of Copper 8-hydroxyquinolinate can be achieved through the reaction of 8-hydroxyquinoline with copper sulfate in the presence of a base.", "Starting Materials": [ "8-hydroxyquinoline", "Copper sulfate", "Base (e.g. sodium hydroxide)" ], "Reaction": [ "Dissolve 8-hydroxyquinoline in a solvent such as ethanol", "Add copper sulfate to the solution and stir", "Add a base such as sodium hydroxide to the solution and stir", "Heat the solution to a temperature of around 60-70°C and stir for several hours", "Allow the solution to cool and filter the resulting precipitate", "Wash the precipitate with a solvent such as ethanol to remove impurities", "Dry the resulting Copper 8-hydroxyquinolinate powder" ] } | |
CAS-Nummer |
10380-28-6 |
Molekularformel |
C18H14CuN2O2 |
Molekulargewicht |
353.9 g/mol |
IUPAC-Name |
copper;quinolin-8-ol |
InChI |
InChI=1S/2C9H7NO.Cu/c2*11-8-5-1-3-7-4-2-6-10-9(7)8;/h2*1-6,11H; |
InChI-Schlüssel |
IURRXCRWRKQLGC-UHFFFAOYSA-N |
SMILES |
C1=CC2=C(C(=C1)O)N=CC=C2.C1=CC2=C(C(=C1)O)N=CC=C2.[Cu] |
Kanonische SMILES |
C1=CC2=C(C(=C1)O)N=CC=C2.C1=CC2=C(C(=C1)O)N=CC=C2.[Cu] |
Dichte |
Relative density (water = 1): 1.63 |
Flammpunkt |
Flash Point > 200 °C |
10380-28-6 13014-03-4 |
|
Physikalische Beschreibung |
Yellow-green solid, insoluble in water; [Hawley] Greenish-yellow crystalline powder; [MSDSonline] GREEN-TO-YELLOW CRYSTALLINE POWDER. |
Piktogramme |
Acute Toxic; Irritant; Environmental Hazard |
Löslichkeit |
Solubility in water: none |
Synonyme |
is(8-quinolinolato)copper(II) copper oxinate copper(II) oxinate Cu 8Q cupric 8-quinolinoxide |
Herkunft des Produkts |
United States |
Retrosynthesis Analysis
AI-Powered Synthesis Planning: Our tool employs the Template_relevance Pistachio, Template_relevance Bkms_metabolic, Template_relevance Pistachio_ringbreaker, Template_relevance Reaxys, Template_relevance Reaxys_biocatalysis model, leveraging a vast database of chemical reactions to predict feasible synthetic routes.
One-Step Synthesis Focus: Specifically designed for one-step synthesis, it provides concise and direct routes for your target compounds, streamlining the synthesis process.
Accurate Predictions: Utilizing the extensive PISTACHIO, BKMS_METABOLIC, PISTACHIO_RINGBREAKER, REAXYS, REAXYS_BIOCATALYSIS database, our tool offers high-accuracy predictions, reflecting the latest in chemical research and data.
Strategy Settings
Precursor scoring | Relevance Heuristic |
---|---|
Min. plausibility | 0.01 |
Model | Template_relevance |
Template Set | Pistachio/Bkms_metabolic/Pistachio_ringbreaker/Reaxys/Reaxys_biocatalysis |
Top-N result to add to graph | 6 |
Feasible Synthetic Routes
Q1: How does oxine-copper exert its fungicidal activity?
A1: While the precise mechanism is still under investigation, research suggests that oxine-copper disrupts fungal cell membrane function. [] One proposed mechanism suggests that oxine-copper dissociates, releasing copper ions that interfere with essential cellular processes. [] Additionally, oxine-copper might interfere with fungal enzyme activity or DNA synthesis, ultimately leading to cell death. []
Q2: What are the downstream effects of oxine-copper on fungal pathogens?
A2: Oxine-copper effectively inhibits various stages of fungal development, including sporangium formation, sporangium germination, and zoospore cyst germination. [, ] Studies have demonstrated its potent control against various fungal diseases, such as cucumber downy mildew, grape downy mildew, potato late blight, and Fusarium head blight. [, , , , , ]
Q3: What is the molecular formula and weight of oxine-copper?
A3: Oxine-copper has the molecular formula C18H12CuN2O2 and a molecular weight of 351.82 g/mol. [, ]
Q4: How is the structure of oxine-copper characterized?
A4: X-ray crystallography studies have revealed that oxine-copper adopts a planar structure, with the copper atom centrally coordinated to two 8-hydroxyquinolinate ligands. [, ] This planar structure contributes to its ability to form stacked arrangements with electron acceptor molecules, as seen in charge-transfer complexes. []
Q5: What are the material compatibility concerns with oxine-copper?
A5: While generally stable, oxine-copper can contribute to corrosion in some metal systems. [] This necessitates careful consideration when formulating oxine-copper-containing products for specific applications.
Q6: Does oxine-copper exhibit catalytic activity?
A6: Yes, oxine-copper can act as a catalyst in certain chemical reactions. [, ] For example, it catalyzes the chemiluminescent oxidation of luminol, which forms the basis for a sensitive analytical method to detect oxine-copper. []
Q7: What are the specific applications of oxine-copper's catalytic properties?
A7: Its catalytic properties are utilized in various fields, including analytical chemistry for trace metal detection [], organic synthesis [], and potentially in the development of new materials.
Q8: Have computational methods been employed to study oxine-copper?
A8: Yes, computational chemistry techniques, such as density functional theory (DFT) calculations, have been used to investigate the electronic structure, bonding, and reactivity of oxine-copper and its derivatives. [] These studies provide valuable insights into its chemical behavior and guide the development of new applications.
Q9: How do structural modifications of oxine-copper affect its activity?
A9: Modifying the oxine-copper structure can significantly influence its fungicidal activity, solubility, and other properties. For example, introducing alkyl groups at the 5-position of the 8-hydroxyquinolinate ligand can enhance solubility in organic solvents. [] These modifications are crucial for optimizing oxine-copper derivatives for specific applications.
Q10: What strategies are employed to enhance oxine-copper's stability and solubility?
A10: Researchers have explored different formulation strategies to improve oxine-copper's stability, solubility, and bioavailability. These include incorporating it into various formulations like wettable powders, water-dispersible granules, and emulsions. [, ] Additionally, combining oxine-copper with other fungicides can enhance its efficacy and potentially mitigate resistance development. [, , , , ]
Q11: What are the environmental concerns associated with oxine-copper?
A11: While effective as a fungicide, oxine-copper's potential environmental impact is a concern. Its use is regulated to minimize risks to ecosystems and human health. [, ] Research focuses on understanding its degradation pathways in the environment and developing strategies to mitigate any negative impacts.
Q12: What measures are taken to ensure the safe handling and use of oxine-copper?
A12: Strict safety regulations and guidelines govern the handling, application, and disposal of oxine-copper-containing products. [, ] These measures are crucial for minimizing occupational exposure and environmental contamination.
Q13: How are oxine-copper residues detected and quantified?
A13: Several analytical techniques are employed for detecting and quantifying oxine-copper residues in various matrices, including:
- High-Performance Liquid Chromatography (HPLC): This method is widely used for analyzing oxine-copper residues in water, soil, and crops. [, , , , , ]
- Gas Chromatography (GC): GC coupled with various detectors, such as flame ionization (FID) or mass spectrometry (MS), is utilized for analyzing oxine-copper in water and other samples. [, ]
- Micellar Electrokinetic Chromatography (MEKC): This technique offers a rapid and efficient way to separate and detect oxine-copper in environmental samples. []
- Chemiluminescence Detection: This highly sensitive method exploits oxine-copper's ability to catalyze luminol chemiluminescence for detection in water samples. []
Q14: Are there alternatives to oxine-copper for fungicidal applications?
A14: Yes, several alternative fungicides are available, each with its own efficacy and safety profile. Examples include: * DMI fungicides: Propiconazole, difenoconazole, tebuconazole [, , ]* SDHI fungicides: Fluopyram, fluxapyroxad []* Strobilurin fungicides: Azoxystrobin, pyraclostrobin [, ]* Other fungicides: Mancozeb, metalaxyl, iprodione, chlorothalonil [, , , ]
Q15: How are oxine-copper-containing wastes managed?
A15: Proper waste management is crucial to minimize environmental risks. Regulations and guidelines dictate the disposal of oxine-copper-containing products and waste. Recycling options are limited due to its chemical nature, emphasizing the importance of responsible use and disposal.
Q16: What is the historical context of oxine-copper research?
A16: Oxine-copper has been studied for several decades. Early research focused on its fungicidal properties and applications in agriculture. [, ] Over time, research expanded to encompass its analytical applications, [, , , , ] catalytic properties, [, ] and potential in material science.
Q17: How does oxine-copper research intersect with other scientific disciplines?
A17: Oxine-copper research exemplifies cross-disciplinary collaboration. It bridges fields like:* Agricultural Science: For developing disease control strategies in crops. [, , , , , ]* Analytical Chemistry: For devising sensitive detection methods for oxine-copper and other analytes. [, , , , ] * Environmental Science: For assessing its fate and potential impacts in the environment. [, , ]* Material Science: For exploring its use in developing novel materials with tailored properties. [, ]
Haftungsausschluss und Informationen zu In-Vitro-Forschungsprodukten
Bitte beachten Sie, dass alle Artikel und Produktinformationen, die auf BenchChem präsentiert werden, ausschließlich zu Informationszwecken bestimmt sind. Die auf BenchChem zum Kauf angebotenen Produkte sind speziell für In-vitro-Studien konzipiert, die außerhalb lebender Organismen durchgeführt werden. In-vitro-Studien, abgeleitet von dem lateinischen Begriff "in Glas", beinhalten Experimente, die in kontrollierten Laborumgebungen unter Verwendung von Zellen oder Geweben durchgeführt werden. Es ist wichtig zu beachten, dass diese Produkte nicht als Arzneimittel oder Medikamente eingestuft sind und keine Zulassung der FDA für die Vorbeugung, Behandlung oder Heilung von medizinischen Zuständen, Beschwerden oder Krankheiten erhalten haben. Wir müssen betonen, dass jede Form der körperlichen Einführung dieser Produkte in Menschen oder Tiere gesetzlich strikt untersagt ist. Es ist unerlässlich, sich an diese Richtlinien zu halten, um die Einhaltung rechtlicher und ethischer Standards in Forschung und Experiment zu gewährleisten.