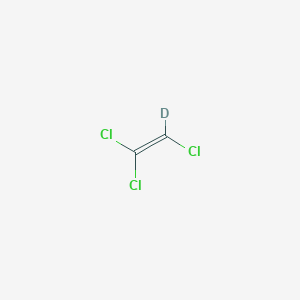
Trichloroethylene-d
Übersicht
Beschreibung
Trichloroethylene-d is a useful research compound. Its molecular formula is C2HCl3 and its molecular weight is 132.39 g/mol. The purity is usually 95%.
The exact mass of the compound this compound is unknown and the complexity rating of the compound is unknown. The United Nations designated GHS hazard class pictogram is Irritant;Health Hazard, and the GHS signal word is DangerThe storage condition is unknown. Please store according to label instructions upon receipt of goods.
BenchChem offers high-quality this compound suitable for many research applications. Different packaging options are available to accommodate customers' requirements. Please inquire for more information about this compound including the price, delivery time, and more detailed information at info@benchchem.com.
Wissenschaftliche Forschungsanwendungen
Cancer Epidemiology : Trichloroethylene has been associated with increased incidences of kidney, liver, and non-Hodgkin's lymphoma cancers among occupationally exposed individuals (Wartenberg, Reyner, & Scott, 2000).
Groundwater Remediation : Palladium nanoparticles bio-precipitated on bacteria and encapsulated in various materials have shown effectiveness in dechlorinating trichloroethylene in contaminated groundwater, with potential applications in environmental remediation (Hennebel et al., 2009).
Health Risk Assessment : Significant research has been conducted to understand the health risks of trichloroethylene exposure, with emphasis on its metabolism and toxicity, and the varying perspectives on critical scientific issues (Chiu, Caldwell, Keshava, & Scott, 2006).
Occupational Health : Trichloroethylene exposure in occupational settings has been linked to various health effects, including skin irritation, systemic toxicity, and potential autoimmune responses (Condé‐Salazar, Guimaraens, Romero, & Yus, 1983; Griffin, Gilbert, & Pumford, 2000).
Mitochondrial Dysfunction : Metabolites of trichloroethylene have been shown to induce mitochondrial dysfunction in placental cells, suggesting a mechanism for adverse effects on pregnancy (Elkin, Bridges, & Loch-Caruso, 2019).
Photocatalysis : Studies have explored the oxidation of trichloroethylene in air using heterogeneous photocatalysis, potentially useful in air purification technologies (Jacoby, Blake, Noble, & Koval, 1995).
Diffusion Coefficient Measurement : Research has been conducted to determine the diffusion coefficient of trichloroethylene in water, crucial for understanding its behavior in aquatic environments (Rossi et al., 2015).
Wirkmechanismus
Target of Action
Trichloroethylene (TCE) is a halocarbon commonly used as an industrial solvent . It is metabolized in the body by two major pathways: the oxidative pathway and the glutathione-conjugation pathway . The primary targets of TCE are the liver and kidneys, where it is metabolized to multiple metabolites .
Mode of Action
TCE interacts with its targets primarily through metabolic transformation. The oxidative pathway and the glutathione-conjugation pathway have been shown to be both toxic and carcinogenic to the kidneys . The metabolite S-dichlorovinyl-L-cysteine has been linked with the development of kidney cancer .
Biochemical Pathways
TCE is metabolized in the body by two major pathways: the oxidative pathway and the glutathione-conjugation pathway . Many animal studies show that TCE and its metabolites are associated with several health effects, including cancer .
Pharmacokinetics
The pharmacokinetics of TCE is complex. It is clear that TCE is metabolized to multiple metabolites either locally or into systemic circulation . Many of these metabolites are thought to have toxicological importance .
Result of Action
TCE and its metabolites are associated with several health effects, including cancer . Studies with experimental animals and human tissues indicate a genotoxic mode of action . TCE has been shown to induce lung tumors in rodents . Acute inhalation or ingestion of TCE can cause systemic effects such as excitement, headache, dizziness, nausea, and vomiting followed by loss of coordination, drowsiness, and difficulty speaking .
Safety and Hazards
Zukünftige Richtungen
The U.S. Environmental Protection Agency (EPA) announced a proposal to ban all uses of TCE due to its serious health risks including cancer, neurotoxicity, and reproductive toxicity . This proposal aligns with President Biden’s Cancer Moonshot, a whole-of-government approach to end cancer as we know it .
Biochemische Analyse
Biochemical Properties
Trichloroethylene-d plays a significant role in biochemical reactions. It interacts with various enzymes, proteins, and other biomolecules. The main biodegradation processes leading to this compound biodegradation by isolated bacteria and mixed cultures are anaerobic reductive dechlorination, anaerobic cometabolic reductive dichlorination, aerobic co-metabolism, and aerobic direct oxidation .
Cellular Effects
This compound has profound effects on various types of cells and cellular processes. It influences cell function, including impacts on cell signaling pathways, gene expression, and cellular metabolism .
Molecular Mechanism
This compound exerts its effects at the molecular level through binding interactions with biomolecules, enzyme inhibition or activation, and changes in gene expression .
Temporal Effects in Laboratory Settings
In laboratory settings, the effects of this compound change over time. This includes information on the product’s stability, degradation, and any long-term effects on cellular function observed in in vitro or in vivo studies .
Dosage Effects in Animal Models
The effects of this compound vary with different dosages in animal models. This includes any threshold effects observed in these studies, as well as any toxic or adverse effects at high doses .
Metabolic Pathways
This compound is involved in various metabolic pathways, interacting with enzymes or cofactors. This also includes any effects on metabolic flux or metabolite levels .
Transport and Distribution
This compound is transported and distributed within cells and tissues. This includes any transporters or binding proteins that it interacts with, as well as any effects on its localization or accumulation .
Subcellular Localization
The subcellular localization of this compound and any effects on its activity or function include any targeting signals or post-translational modifications that direct it to specific compartments or organelles .
Eigenschaften
IUPAC Name |
1,1,2-trichloro-2-deuterioethene | |
---|---|---|
Source | PubChem | |
URL | https://pubchem.ncbi.nlm.nih.gov | |
Description | Data deposited in or computed by PubChem | |
InChI |
InChI=1S/C2HCl3/c3-1-2(4)5/h1H/i1D | |
Source | PubChem | |
URL | https://pubchem.ncbi.nlm.nih.gov | |
Description | Data deposited in or computed by PubChem | |
InChI Key |
XSTXAVWGXDQKEL-MICDWDOJSA-N | |
Source | PubChem | |
URL | https://pubchem.ncbi.nlm.nih.gov | |
Description | Data deposited in or computed by PubChem | |
Canonical SMILES |
C(=C(Cl)Cl)Cl | |
Source | PubChem | |
URL | https://pubchem.ncbi.nlm.nih.gov | |
Description | Data deposited in or computed by PubChem | |
Isomeric SMILES |
[2H]C(=C(Cl)Cl)Cl | |
Source | PubChem | |
URL | https://pubchem.ncbi.nlm.nih.gov | |
Description | Data deposited in or computed by PubChem | |
Molecular Formula |
C2HCl3 | |
Source | PubChem | |
URL | https://pubchem.ncbi.nlm.nih.gov | |
Description | Data deposited in or computed by PubChem | |
DSSTOX Substance ID |
DTXSID40482422 | |
Record name | Trichloroethylene-d | |
Source | EPA DSSTox | |
URL | https://comptox.epa.gov/dashboard/DTXSID40482422 | |
Description | DSSTox provides a high quality public chemistry resource for supporting improved predictive toxicology. | |
Molecular Weight |
132.39 g/mol | |
Source | PubChem | |
URL | https://pubchem.ncbi.nlm.nih.gov | |
Description | Data deposited in or computed by PubChem | |
CAS No. |
13291-68-4 | |
Record name | Trichloroethylene-d | |
Source | CAS Common Chemistry | |
URL | https://commonchemistry.cas.org/detail?cas_rn=13291-68-4 | |
Description | CAS Common Chemistry is an open community resource for accessing chemical information. Nearly 500,000 chemical substances from CAS REGISTRY cover areas of community interest, including common and frequently regulated chemicals, and those relevant to high school and undergraduate chemistry classes. This chemical information, curated by our expert scientists, is provided in alignment with our mission as a division of the American Chemical Society. | |
Explanation | The data from CAS Common Chemistry is provided under a CC-BY-NC 4.0 license, unless otherwise stated. | |
Record name | Trichloroethylene-d | |
Source | EPA DSSTox | |
URL | https://comptox.epa.gov/dashboard/DTXSID40482422 | |
Description | DSSTox provides a high quality public chemistry resource for supporting improved predictive toxicology. | |
Record name | 13291-68-4 | |
Source | European Chemicals Agency (ECHA) | |
URL | https://echa.europa.eu/information-on-chemicals | |
Description | The European Chemicals Agency (ECHA) is an agency of the European Union which is the driving force among regulatory authorities in implementing the EU's groundbreaking chemicals legislation for the benefit of human health and the environment as well as for innovation and competitiveness. | |
Explanation | Use of the information, documents and data from the ECHA website is subject to the terms and conditions of this Legal Notice, and subject to other binding limitations provided for under applicable law, the information, documents and data made available on the ECHA website may be reproduced, distributed and/or used, totally or in part, for non-commercial purposes provided that ECHA is acknowledged as the source: "Source: European Chemicals Agency, http://echa.europa.eu/". Such acknowledgement must be included in each copy of the material. ECHA permits and encourages organisations and individuals to create links to the ECHA website under the following cumulative conditions: Links can only be made to webpages that provide a link to the Legal Notice page. | |
Retrosynthesis Analysis
AI-Powered Synthesis Planning: Our tool employs the Template_relevance Pistachio, Template_relevance Bkms_metabolic, Template_relevance Pistachio_ringbreaker, Template_relevance Reaxys, Template_relevance Reaxys_biocatalysis model, leveraging a vast database of chemical reactions to predict feasible synthetic routes.
One-Step Synthesis Focus: Specifically designed for one-step synthesis, it provides concise and direct routes for your target compounds, streamlining the synthesis process.
Accurate Predictions: Utilizing the extensive PISTACHIO, BKMS_METABOLIC, PISTACHIO_RINGBREAKER, REAXYS, REAXYS_BIOCATALYSIS database, our tool offers high-accuracy predictions, reflecting the latest in chemical research and data.
Strategy Settings
Precursor scoring | Relevance Heuristic |
---|---|
Min. plausibility | 0.01 |
Model | Template_relevance |
Template Set | Pistachio/Bkms_metabolic/Pistachio_ringbreaker/Reaxys/Reaxys_biocatalysis |
Top-N result to add to graph | 6 |
Feasible Synthetic Routes
Haftungsausschluss und Informationen zu In-Vitro-Forschungsprodukten
Bitte beachten Sie, dass alle Artikel und Produktinformationen, die auf BenchChem präsentiert werden, ausschließlich zu Informationszwecken bestimmt sind. Die auf BenchChem zum Kauf angebotenen Produkte sind speziell für In-vitro-Studien konzipiert, die außerhalb lebender Organismen durchgeführt werden. In-vitro-Studien, abgeleitet von dem lateinischen Begriff "in Glas", beinhalten Experimente, die in kontrollierten Laborumgebungen unter Verwendung von Zellen oder Geweben durchgeführt werden. Es ist wichtig zu beachten, dass diese Produkte nicht als Arzneimittel oder Medikamente eingestuft sind und keine Zulassung der FDA für die Vorbeugung, Behandlung oder Heilung von medizinischen Zuständen, Beschwerden oder Krankheiten erhalten haben. Wir müssen betonen, dass jede Form der körperlichen Einführung dieser Produkte in Menschen oder Tiere gesetzlich strikt untersagt ist. Es ist unerlässlich, sich an diese Richtlinien zu halten, um die Einhaltung rechtlicher und ethischer Standards in Forschung und Experiment zu gewährleisten.