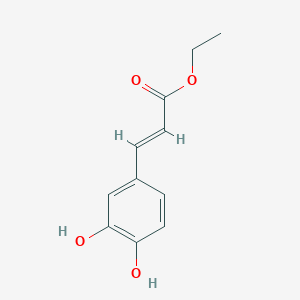
Ethylcaffeat
Übersicht
Beschreibung
Äthylcaffeat ist ein Ester der Caffeinsäure, einer Hydroxyzimtsäure, und Ethanol. Es ist eine natürlich vorkommende organische Verbindung, die in verschiedenen Pflanzen vorkommt, darunter Bidens pilosa, Polygonum amplexicaule und Ligularia fischeri . Äthylcaffeat ist bekannt für seine verschiedenen biologischen Aktivitäten, darunter entzündungshemmende, krebshemmende und antifibrotische Eigenschaften .
Wissenschaftliche Forschungsanwendungen
Wirkmechanismus
Target of Action
Ethyl caffeate primarily targets NF-kappaB and aldosterone synthase (CYP11B2) . NF-kappaB is a protein complex that controls the transcription of DNA and plays a key role in regulating the immune response to infection. Aldosterone synthase is a key enzyme for the biosynthesis of aldosterone, which plays a significant role in the regulation of blood pressure .
Mode of Action
Ethyl caffeate suppresses the activation of NF-kappaB and its downstream inflammatory mediators, iNOS, COX-2, and PGE2 . It also inhibits aldosterone synthase, thereby potentially regulating blood pressure .
Biochemical Pathways
Ethyl caffeate is a conjugate of caffeic acid and ethanol, synthesized via the phenylpropanoid pathway in plants . The genes involved in the caffeic acid synthesis pathway, such as tyrosine ammonia-lyase (TAL) and p-coumaric acid hydroxylase (HpaBC), play a crucial role in its biosynthesis .
Pharmacokinetics
It has been found that ethyl caffeate can be administered intraperitoneally in rats .
Result of Action
Ethyl caffeate exhibits anticancer, anti-inflammatory, and antifibrotic activities . It has been shown to prevent dimethylnitrosamine-induced loss in body and liver weight, as well as to reduce the degree of liver injury .
Action Environment
The action of ethyl caffeate can be influenced by various environmental factors. For instance, the presence of ethanol is necessary for the successful synthesis of ethyl caffeate in Escherichia coli . More research is needed to fully understand how other environmental factors influence the action, efficacy, and stability of ethyl caffeate.
Biochemische Analyse
Biochemical Properties
Ethyl caffeate is involved in the caffeic acid synthesis pathway . The genes involved in this pathway include tyrosine ammonia-lyase (TAL) and p-coumaric acid hydroxylase (HpaBC), which were introduced into Escherichia coli along with 4-coumaroyl CoA ligase (4CL) and acyltransferases (AtCAT) cloned from Arabidopsis thaliana . In the presence of ethanol, E. coli harboring these genes successfully synthesized ethyl caffeate .
Cellular Effects
Ethyl caffeate has been found to suppress NF-kappaB activation and its downstream inflammatory mediators, iNOS, COX-2, and PGE2 in vitro or in mouse skin . It also exhibits anticancer, anti-inflammatory, and antifibrotic activities .
Molecular Mechanism
Pharmacophore modeling, molecular docking, and molecular dynamics simulation studies indicate that ethyl caffeate is a potential inhibitor of the aldosterone synthase (CYP11B2), a key enzyme for the biosynthesis of aldosterone, which plays a significant role in the regulation of blood pressure .
Dosage Effects in Animal Models
Ethyl caffeate administered intraperitoneally in rats previously is able to prevent the dimethylnitrosamine-induced loss in body and liver weight, as well as to reduce the degree of liver injury
Metabolic Pathways
Ethyl caffeate is synthesized from tyrosine by deamination and hydroxylation, and needs to be activated by the attachment of coenzyme A (CoA)
Vorbereitungsmethoden
Äthylcaffeat kann durch Veresterung von Caffeinsäure mit Ethanol synthetisiert werden. Diese Reaktion erfordert typischerweise einen sauren Katalysator wie Schwefelsäure und wird unter Rückflussbedingungen durchgeführt . In industrieller Umgebung kann Äthylcaffeat auch mit Hilfe von biokatalytischen Verfahren hergestellt werden. Beispielsweise wurde die Expression von Caffeoyl-CoA-Acyltransferase in Escherichia coli zur Biosynthese von Äthylcaffeat verwendet .
Analyse Chemischer Reaktionen
Äthylcaffeat unterliegt verschiedenen chemischen Reaktionen, darunter:
Oxidation: Äthylcaffeat kann zu Chinonen und anderen Oxidationsprodukten oxidiert werden.
Reduktion: Die Reduktion von Äthylcaffeat kann zur Bildung von Dihydroderivaten führen.
Substitution: Äthylcaffeat reagiert mit Methylamin unter Bildung grüner Pigmente.
Häufige Reagenzien und Bedingungen, die in diesen Reaktionen verwendet werden, umfassen Oxidationsmittel wie Kaliumpermanganat für die Oxidation und Reduktionsmittel wie Natriumborhydrid für die Reduktion. Die Hauptprodukte, die aus diesen Reaktionen gebildet werden, hängen von den spezifischen Bedingungen und Reagenzien ab, die verwendet werden.
Wissenschaftliche Forschungsanwendungen
Äthylcaffeat hat eine breite Palette von wissenschaftlichen Forschungsanwendungen:
Chemie: Äthylcaffeat wird als Modellverbindung verwendet, um die Reaktivität von Hydroxyzimtsäurederivaten zu untersuchen.
Medizin: Äthylcaffeat hat sich als vielversprechend erwiesen, um Leberschäden vorzubeugen und Entzündungen zu reduzieren.
Wirkmechanismus
Äthylcaffeat entfaltet seine Wirkungen über verschiedene molekulare Zielstrukturen und Signalwege. Es unterdrückt die Aktivierung von NF-kappaB und seinen nachgeschalteten Entzündungsmediatoren wie iNOS, COX-2 und PGE2 . Zusätzlich hemmt Äthylcaffeat den Arylhydrocarbon-Rezeptor (AhR)-Signalweg, der mit seiner antiallergischen Aktivität verbunden ist . Es wirkt auch als Inhibitor der Aldosteron-Synthase und reguliert so den Blutdruck .
Vergleich Mit ähnlichen Verbindungen
Äthylcaffeat ähnelt anderen Hydroxyzimtsäurederivaten, wie z. B.:
Caffeinsäure: Die Stammverbindung von Äthylcaffeat, bekannt für ihre antioxidativen Eigenschaften.
Chlorogensäure: Ein Konjugat von Caffeinsäure und Chinasäure, das häufig in Kaffee vorkommt und für seine gesundheitlichen Vorteile bekannt ist.
Ferulasäure: Ein weiteres Hydroxyzimtsäurederivat mit antioxidativen und entzündungshemmenden Eigenschaften.
Äthylcaffeat ist einzigartig aufgrund seiner Esterbindung mit Ethanol, die im Vergleich zu seiner Stammverbindung Caffeinsäure unterschiedliche biologische Aktivitäten und chemische Reaktivität verleiht .
Eigenschaften
IUPAC Name |
ethyl (E)-3-(3,4-dihydroxyphenyl)prop-2-enoate | |
---|---|---|
Source | PubChem | |
URL | https://pubchem.ncbi.nlm.nih.gov | |
Description | Data deposited in or computed by PubChem | |
InChI |
InChI=1S/C11H12O4/c1-2-15-11(14)6-4-8-3-5-9(12)10(13)7-8/h3-7,12-13H,2H2,1H3/b6-4+ | |
Source | PubChem | |
URL | https://pubchem.ncbi.nlm.nih.gov | |
Description | Data deposited in or computed by PubChem | |
InChI Key |
WDKYDMULARNCIS-GQCTYLIASA-N | |
Source | PubChem | |
URL | https://pubchem.ncbi.nlm.nih.gov | |
Description | Data deposited in or computed by PubChem | |
Canonical SMILES |
CCOC(=O)C=CC1=CC(=C(C=C1)O)O | |
Source | PubChem | |
URL | https://pubchem.ncbi.nlm.nih.gov | |
Description | Data deposited in or computed by PubChem | |
Isomeric SMILES |
CCOC(=O)/C=C/C1=CC(=C(C=C1)O)O | |
Source | PubChem | |
URL | https://pubchem.ncbi.nlm.nih.gov | |
Description | Data deposited in or computed by PubChem | |
Molecular Formula |
C11H12O4 | |
Source | PubChem | |
URL | https://pubchem.ncbi.nlm.nih.gov | |
Description | Data deposited in or computed by PubChem | |
DSSTOX Substance ID |
DTXSID70876375 | |
Record name | Ethyl 3,4-dihydroxycinnamate | |
Source | EPA DSSTox | |
URL | https://comptox.epa.gov/dashboard/DTXSID70876375 | |
Description | DSSTox provides a high quality public chemistry resource for supporting improved predictive toxicology. | |
Molecular Weight |
208.21 g/mol | |
Source | PubChem | |
URL | https://pubchem.ncbi.nlm.nih.gov | |
Description | Data deposited in or computed by PubChem | |
CAS No. |
66648-50-8, 102-37-4 | |
Record name | Ethyl trans-caffeate | |
Source | CAS Common Chemistry | |
URL | https://commonchemistry.cas.org/detail?cas_rn=66648-50-8 | |
Description | CAS Common Chemistry is an open community resource for accessing chemical information. Nearly 500,000 chemical substances from CAS REGISTRY cover areas of community interest, including common and frequently regulated chemicals, and those relevant to high school and undergraduate chemistry classes. This chemical information, curated by our expert scientists, is provided in alignment with our mission as a division of the American Chemical Society. | |
Explanation | The data from CAS Common Chemistry is provided under a CC-BY-NC 4.0 license, unless otherwise stated. | |
Record name | Ethyl caffeate | |
Source | ChemIDplus | |
URL | https://pubchem.ncbi.nlm.nih.gov/substance/?source=chemidplus&sourceid=0000102374 | |
Description | ChemIDplus is a free, web search system that provides access to the structure and nomenclature authority files used for the identification of chemical substances cited in National Library of Medicine (NLM) databases, including the TOXNET system. | |
Record name | Ethyl 3,4-dihydroxycinnamate | |
Source | EPA DSSTox | |
URL | https://comptox.epa.gov/dashboard/DTXSID70876375 | |
Description | DSSTox provides a high quality public chemistry resource for supporting improved predictive toxicology. | |
Record name | Caffeic acid ethyl ester | |
Source | Human Metabolome Database (HMDB) | |
URL | http://www.hmdb.ca/metabolites/HMDB0301712 | |
Description | The Human Metabolome Database (HMDB) is a freely available electronic database containing detailed information about small molecule metabolites found in the human body. | |
Explanation | HMDB is offered to the public as a freely available resource. Use and re-distribution of the data, in whole or in part, for commercial purposes requires explicit permission of the authors and explicit acknowledgment of the source material (HMDB) and the original publication (see the HMDB citing page). We ask that users who download significant portions of the database cite the HMDB paper in any resulting publications. | |
Retrosynthesis Analysis
AI-Powered Synthesis Planning: Our tool employs the Template_relevance Pistachio, Template_relevance Bkms_metabolic, Template_relevance Pistachio_ringbreaker, Template_relevance Reaxys, Template_relevance Reaxys_biocatalysis model, leveraging a vast database of chemical reactions to predict feasible synthetic routes.
One-Step Synthesis Focus: Specifically designed for one-step synthesis, it provides concise and direct routes for your target compounds, streamlining the synthesis process.
Accurate Predictions: Utilizing the extensive PISTACHIO, BKMS_METABOLIC, PISTACHIO_RINGBREAKER, REAXYS, REAXYS_BIOCATALYSIS database, our tool offers high-accuracy predictions, reflecting the latest in chemical research and data.
Strategy Settings
Precursor scoring | Relevance Heuristic |
---|---|
Min. plausibility | 0.01 |
Model | Template_relevance |
Template Set | Pistachio/Bkms_metabolic/Pistachio_ringbreaker/Reaxys/Reaxys_biocatalysis |
Top-N result to add to graph | 6 |
Feasible Synthetic Routes
ANone: Ethyl caffeate exhibits its effects through various mechanisms:
- Direct binding to enzymes: Research suggests Ethyl caffeate directly binds to the active site of enzymes like human pancreatic α-amylase [], HIV protease [], and 5-lipoxygenase (5-LOX) []. This binding inhibits their activity, leading to downstream effects like reduced carbohydrate digestion [] and suppression of pro-inflammatory leukotriene B4 production [].
- Modulation of signaling pathways: Ethyl caffeate can suppress the PI3K, ERK1/2, and p38 kinase activities by directly binding to these kinases []. This leads to the downstream inhibition of AP1 and NF-κB transactivation, both crucial in inflammatory responses and carcinogenesis.
- Interaction with Aryl hydrocarbon receptor (AhR): Ethyl caffeate inhibits AhR signaling, impacting the expression of genes like CYP1A1 []. This inhibition is linked to reduced mast cell activation and allergic responses.
A:
- Spectroscopic Data: Ethyl caffeate's structure has been elucidated using various spectroscopic techniques, including 1H-NMR, 13C-NMR, and electron ionization mass spectroscopy (EI-MS) [].
ANone:
- Stability: Ethyl caffeate demonstrates stability under acidic conditions (pH 2-5) []. It shows better stability in whole blood at 0°C compared to 25°C []. Additionally, it exhibits higher stability in acidified plasma than in unacidified plasma at 25°C [].
- Applications: The stability of Ethyl caffeate under specific conditions makes it suitable for various applications:
ANone: While Ethyl caffeate exhibits biological activities, the provided research focuses primarily on its role as an enzyme inhibitor rather than a catalyst. Therefore, information regarding its catalytic properties, reaction mechanisms, and selectivity is limited within the provided context.
ANone: Yes, molecular docking studies have been performed to investigate the interaction of Ethyl caffeate with its target proteins. For instance:
- HIV protease: Molecular docking simulations with energy minimization and molecular dynamic (MD) simulation revealed that Ethyl caffeate binds to the active site of HIV protease, potentially explaining its inhibitory effects [].
- EGFR: Molecular docking studies showed that Ethyl caffeate exhibits high binding energy to EGFR, suggesting a potential mechanism for its synergistic antifungal effect with Fluconazole [].
ANone: While specific SAR studies focusing solely on Ethyl caffeate are not extensively detailed in the provided papers, research comparing the activities of caffeic acid and its derivatives, including Ethyl caffeate, offers some insights:
- Antioxidant activity: Ethyl caffeate generally demonstrates stronger antioxidant activity compared to caffeic acid [].
- α-amylase inhibition: Ethyl caffeate displays a distinct mode of inhibition on human α-amylase compared to the flavonol myricetin, causing disorder in the active site binding cleft [].
ANone: While a detailed historical timeline is not provided, the research highlights that Ethyl caffeate has been a subject of scientific interest, particularly regarding its potential health benefits and pharmacological activities:
- Early research: Early studies investigated Ethyl caffeate's antioxidant properties and its potential applications in food preservation [].
- Expanding research focus: Subsequent research has explored its diverse biological activities, including anti-inflammatory [, , ], antifungal [], anti-HIV [], and anticancer effects [, ].
ANone: Yes, research on Ethyl caffeate spans multiple disciplines, including:
- Chemistry: Isolation and structural characterization from natural sources [, , , , , , , , , , ], chemical synthesis [], and analysis of its stability [].
- Biology: Investigating its biological activities, such as antioxidant [, , ], anti-inflammatory [, , ], antifungal [], anti-HIV [], and anticancer effects [, ].
- Pharmacology: Exploring its pharmacokinetics [], target identification and validation [], and potential for drug development [, ].
- Food Science: Evaluating its potential use as a natural preservative [, ].
Haftungsausschluss und Informationen zu In-Vitro-Forschungsprodukten
Bitte beachten Sie, dass alle Artikel und Produktinformationen, die auf BenchChem präsentiert werden, ausschließlich zu Informationszwecken bestimmt sind. Die auf BenchChem zum Kauf angebotenen Produkte sind speziell für In-vitro-Studien konzipiert, die außerhalb lebender Organismen durchgeführt werden. In-vitro-Studien, abgeleitet von dem lateinischen Begriff "in Glas", beinhalten Experimente, die in kontrollierten Laborumgebungen unter Verwendung von Zellen oder Geweben durchgeführt werden. Es ist wichtig zu beachten, dass diese Produkte nicht als Arzneimittel oder Medikamente eingestuft sind und keine Zulassung der FDA für die Vorbeugung, Behandlung oder Heilung von medizinischen Zuständen, Beschwerden oder Krankheiten erhalten haben. Wir müssen betonen, dass jede Form der körperlichen Einführung dieser Produkte in Menschen oder Tiere gesetzlich strikt untersagt ist. Es ist unerlässlich, sich an diese Richtlinien zu halten, um die Einhaltung rechtlicher und ethischer Standards in Forschung und Experiment zu gewährleisten.