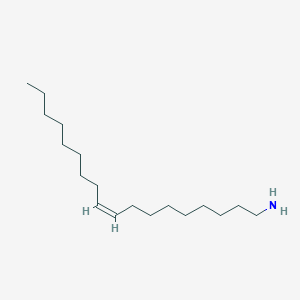
Oleylamine
Übersicht
Beschreibung
Oleylamine (OLA), a long-chain primary alkyl amine (C₁₈H₃₅NH₂), is a versatile reagent in nanomaterial synthesis, corrosion inhibition, and surface stabilization. It is liquid at room temperature, simplifying post-synthesis purification . With a high boiling point (364°C), OLA is ideal for high-temperature reactions, such as nanoparticle synthesis, where it acts as a solvent, reducing agent, and stabilizer . Its amine (-NH₂) group provides moderate binding affinity to metal surfaces, enabling controlled nucleation and growth of nanomaterials . Commercially available OLA (≥98% purity) is cost-effective but may contain impurities affecting reproducibility .
Vorbereitungsmethoden
Industrial Synthesis of Oleylamine via Chlorination-Amidation-Reduction
The most widely documented industrial method for this compound production involves a four-step sequence: chlorination, amidation, reduction, and purification. This process, patented by Gibson et al., achieves >99% purity and avoids isomerization of the olefinic bond .
Chlorination of Oleic Acid
Oleic acid (≥99% purity) undergoes chlorination with neat thionyl chloride (SOCl₂) in dichloromethane (DCM) at reflux (39–41°C). The reaction produces oleoyl chloride with 97–100% conversion efficiency :
3(\text{CH}2)7\text{CH}=\text{CH}(\text{CH}2)7\text{COOH} + \text{SOCl}2 \rightarrow \text{CH}3(\text{CH}2)7\text{CH}=\text{CH}(\text{CH}2)7\text{COCl} + \text{SO}2 + \text{HCl}
Key advantages of DCM include its low boiling point (40°C), which minimizes cis-to-trans isomerization compared to toluene (b.p. 108°C) . A typical batch uses 20 kg oleic acid and 10.11 kg SOCl₂ in 160 L DCM, yielding 186 L of oleoyl chloride solution .
Amidation with Liquid Ammonia
The oleoyl chloride solution is added to liquid ammonia (–40°C) in DCM at 0–10°C, forming oleylamide:
3(\text{CH}2)7\text{CH}=\text{CH}(\text{CH}2)7\text{COCl} + 2\text{NH}3 \rightarrow \text{CH}3(\text{CH}2)7\text{CH}=\text{CH}(\text{CH}2)7\text{CONH}2 + \text{NH}_4\text{Cl}
This exothermic reaction requires precise temperature control to prevent hydrolysis. Post-reaction washing with 1M Na₂CO₃ reduces residual oleic acid from 24.13% to 1.19% .
Reduction to this compound
Oleylamide is reduced using lithium aluminium hydride (LiAlH₄) in tetrahydrofuran (THF) at 50–60°C. A 2.5:1 molar ratio of LiAlH₄ to amide ensures complete reduction:
3(\text{CH}2)7\text{CH}=\text{CH}(\text{CH}2)7\text{CONH}2 + \text{LiAlH}4 \rightarrow \text{CH}3(\text{CH}2)7\text{CH}=\text{CH}(\text{CH}2)7\text{CH}2\text{NH}2 + \text{LiAlO}2 + \text{H}2
Extraction with tert-butyl methyl ether (TBME) instead of toluene avoids solvent contamination, yielding crude this compound at 94.2% purity .
Purification via Hydrochloride Precipitation
Crude this compound is dissolved in TBME and treated with aqueous HCl to form this compound hydrochloride, which precipitates upon adding acetonitrile:
3(\text{CH}2)7\text{CH}=\text{CH}(\text{CH}2)7\text{CH}2\text{NH}2 + \text{HCl} \rightarrow \text{CH}3(\text{CH}2)7\text{CH}=\text{CH}(\text{CH}2)7\text{CH}2\text{NH}3^+\text{Cl}^-
This step removes non-amine impurities (≤0.05% by GC) and eliminates the need for high-vacuum distillation. Neutralization with NaOH regenerates this compound at 99.5% purity .
Table 1: Key Parameters in Industrial this compound Synthesis
Step | Conditions | Yield | Purity |
---|---|---|---|
Chlorination | 39–41°C, 3 h, DCM | 97–100% | >98% |
Amidation | 0–10°C, NH₃/DCM | 80–85% | 98% |
Reduction | 50–60°C, LiAlH₄/THF | 94.2% | 94.2% |
Hydrochloride Purification | TBME/HCl, acetonitrile | 95–97% | 99.5% |
Grade (Sigma-Aldrich) | Primary Amine Content | Common Impurities |
---|---|---|
70% Technical | 68–72% | Oleic acid, oleamide |
≥98% Primary Amine | 98–99% | Trace aldehydes, moisture |
Distilled (Lab-Scale) | 99.5% | None detected by GC-MS |
Impact of Purity on Materials Synthesis
This compound purity directly influences nanostructure morphology and perovskite crystallinity. For example:
-
BaZrS₃ Perovskites : Using 3 g of 70% technical this compound yields low-temperature (LT) BaZrS₃ with impurities (Sample SI-F), whereas ≥98% this compound produces phase-pure high-temperature (HT) BaZrS₃ (Sample SI-A) .
-
Gold Nanoparticles : Technical-grade this compound results in polydisperse nanoparticles (20–100 nm), while purified this compound yields monodisperse 5 nm particles .
Comparative Analysis of Synthesis Routes
Table 3: Industrial vs. Laboratory Methods
Parameter | Industrial Process | Laboratory Purification |
---|---|---|
Scale | 20–100 kg batches | 1–100 g |
Time | 24–48 h | 6–12 h |
Purity | 99.5% | 98–99.5% |
Key Advantage | High yield, scalability | Removes luminescent impurities |
Key Limitation | Requires hazardous reagents | Low recovery (70–80%) |
Analyse Chemischer Reaktionen
Reaktionstypen
Oleylamin durchläuft verschiedene chemische Reaktionen, darunter:
Oxidation: Oleylamin kann oxidiert werden, um entsprechende Oxide oder andere sauerstoffhaltige Verbindungen zu bilden.
Reduktion: Es kann reduziert werden, um gesättigte Amine zu bilden.
Substitution: Oleylamin kann an Substitutionsreaktionen teilnehmen, bei denen die Aminogruppe durch andere funktionelle Gruppen ersetzt wird.
Kondensation: Es kann mit Carbonsäuren unter Abspaltung eines Wassermoleküls zu Amiden reagieren.
Gängige Reagenzien und Bedingungen
Oxidation: Gängige Oxidationsmittel umfassen Wasserstoffperoxid und Kaliumpermanganat.
Reduktion: Reduktionsmittel wie Lithiumaluminiumhydrid können verwendet werden.
Substitution: Verschiedene Halogenierungsmittel können für Substitutionsreaktionen eingesetzt werden.
Kondensation: Carbonsäuren und Säurechloride sind häufig verwendete Reagenzien.
Hauptprodukte
Oxidation: Oxide und andere sauerstoffhaltige Verbindungen.
Reduktion: Gesättigte Amine.
Substitution: Halogenierte Amine.
Kondensation: Amide.
Wissenschaftliche Forschungsanwendungen
Oleylamin hat eine breite Palette von Anwendungen in der wissenschaftlichen Forschung, darunter:
Chemie: Es wird als Tensid und Stabilisator bei der Synthese von Nanopartikeln verwendet.
Biologie: Oleylamin wird bei der Herstellung verschiedener biologischer Materialien und als Stabilisator für biologische Nanopartikel verwendet.
Industrie: Oleylamin wird bei der Herstellung von Tensiden, Schmiermitteln und anderen Industriechemikalien verwendet.
Wirkmechanismus
Oleylamin entfaltet seine Wirkung durch verschiedene Mechanismen, darunter:
Koordination mit Metallionen: Oleylamin kann mit Metallionen koordinieren und so die Bildung und Stabilisierung von Nanopartikeln beeinflussen.
Oberflächenpassivierung: Es wirkt als Oberflächenpassivierungsmittel, das die Aggregation von Nanopartikeln verhindert und ihre Stabilität erhöht.
Wirkmechanismus
Oleylamine exerts its effects through various mechanisms, including:
Coordination with Metal Ions: this compound can coordinate with metal ions, affecting the formation and stabilization of nanoparticles.
Surface Passivation: It acts as a surface passivating agent, preventing the aggregation of nanoparticles and enhancing their stability.
Reduction: This compound can act as a reducing agent, facilitating the reduction of metal ions to form nanoparticles.
Vergleich Mit ähnlichen Verbindungen
Oleylamine vs. Trioctylphosphine (TOP)
TOP (C₂₄H₅₁P), a phosphine-based ligand, shares functional roles with OLA in nanoparticle synthesis but exhibits distinct chemical behaviors:
Key Findings :
- OLA’s amine group enables faster reaction kinetics due to higher proton affinity, favoring monodisperse nanocrystals .
- TOP’s phosphine group stabilizes smaller nanoparticles via stronger electron interactions, critical for quantum dot synthesis .
This compound vs. Oleic Acid
Oleic acid (carboxylic acid ligand) is often paired with OLA in nanoparticle synthesis:
Key Findings :
- Replacing OLA with oleic acid in CZTSe synthesis shifts morphology from nanowires to nanoparticles, highlighting OLA’s stronger stabilizing capability .
- OLA’s weaker binding to metal oxides necessitates co-surfactants like oleic acid for optimal nanoparticle dispersion .
This compound vs. Octadecylamine
Octadecylamine (C₁₈H₃₇NH₂), a saturated analog of OLA, shares structural similarities but differs in applications:
Key Findings :
- OLA’s unsaturated chain enhances fluidity and interaction with metal surfaces, improving corrosion inhibition .
- Octadecylamine is a minor impurity in commercial OLA but lacks its versatility in nanoparticle synthesis .
This compound vs. N-Oleoylethanolamine
N-Oleoylethanolamine (a bioactive lipid) differs fundamentally from OLA:
Property | This compound (OLA) | N-Oleoylethanolamine |
---|---|---|
Structure | Primary amine | Ethanolamine derivative (amide bond) |
Applications | Nanomaterials, corrosion inhibition | Lipid signaling, biomedical uses |
Data Tables
Table 1: Functional Group Impact on Nanoparticle Properties
Table 2: Application-Specific Performance
Application | This compound Efficiency | Competing Compound Efficiency |
---|---|---|
Corrosion Inhibition | 95% at 100 ppm | Octadecylamine: <85% |
Quantum Dot Synthesis | High monodispersity | TOP: Smaller but polydisperse |
Biologische Aktivität
Oleylamine (OLA) is a long-chain primary amine with the chemical formula CHN. It has garnered significant attention in various fields, including nanotechnology, materials science, and biomedicine due to its unique properties and biological activities. This article provides a comprehensive overview of the biological activity of this compound, supported by research findings, data tables, and case studies.
This compound is primarily used as a surfactant and stabilizing agent in the synthesis of nanoparticles and other materials. Its amphiphilic nature allows it to interact with both polar and non-polar substances, making it an effective agent in various chemical reactions.
Table 1: Key Properties of this compound
Property | Value |
---|---|
Molecular Weight | 281.5 g/mol |
Boiling Point | 360 °C |
Density | 0.818 g/cm³ |
Solubility | Soluble in organic solvents |
Antimicrobial Properties
Research indicates that this compound exhibits antimicrobial activity against various pathogens. In a study focusing on its efficacy against bacterial strains, this compound demonstrated significant inhibitory effects on Gram-positive and Gram-negative bacteria, including Staphylococcus aureus and Escherichia coli. The mechanism appears to involve disruption of the bacterial cell membrane, leading to cell lysis.
Cytotoxicity and Cell Viability
This compound's cytotoxic effects have been evaluated in several cell lines. A notable study assessed its impact on human cancer cell lines (e.g., HeLa and MCF-7). The results indicated that this compound induces apoptosis in these cells, as evidenced by increased markers of apoptosis such as caspase activation and DNA fragmentation.
Case Study: this compound in Cancer Therapy
In a recent investigation, this compound was incorporated into lipid-based nanoparticles for targeted drug delivery in breast cancer treatment. The study found that this compound-enhanced nanoparticles significantly improved the bioavailability of chemotherapeutic agents while reducing systemic toxicity. This suggests potential applications in developing more effective cancer therapies.
Role in Nanoparticle Synthesis
This compound is widely used as a capping agent in the synthesis of metal nanoparticles. Its ability to stabilize nanoparticles during formation is crucial for controlling size and shape, which directly influences their biological activity.
Table 2: this compound in Nanoparticle Synthesis
Nanoparticle Type | Size (nm) | Synthesis Method | Biological Application |
---|---|---|---|
Gold Nanoparticles | 10-50 | Chemical reduction | Drug delivery |
Silver Nanoparticles | 20-100 | Green synthesis | Antimicrobial agents |
Iron Oxide Nanoparticles | 5-20 | Co-precipitation | Magnetic resonance imaging |
Mechanistic Insights
The biological mechanisms underlying this compound's activity are complex and multifaceted. Studies employing spectroscopic techniques such as FTIR have shown that this compound can form hydrogen bonds with other molecules, influencing their reactivity and stability. For instance, in a hybrid system with acetone, this compound facilitated the formation of nanoscale aggregates that exhibited unique photoluminescent properties, suggesting applications in biosensing technologies.
Aggregation-Induced Emission Behavior
Recent research highlighted the aggregation-induced emission (AIE) behavior of this compound when mixed with acetone. The study revealed that as the concentration of acetone increased, the emission color shifted from blue to red, indicating changes in molecular interactions and potential applications in optoelectronic devices.
Q & A
Basic Research Questions
Q. What is the role of oleylamine in nanoparticle synthesis, and how does it influence particle morphology?
this compound acts as a surfactant, stabilizer, and reducing agent in nanoparticle synthesis. Its long hydrocarbon chain prevents aggregation by steric stabilization, while its amine group facilitates metal ion reduction. For example, in FePt nanoparticle synthesis, this compound controls monodispersity (3–10 nm diameter, ±5% deviation) and enables self-assembly into superlattices . To replicate this, dissolve platinum acetylacetonate and iron pentacarbonyl in this compound/oleic acid mixtures under inert gas, followed by thermal decomposition at 200–300°C.
Q. How do impurities in technical-grade this compound (70%) affect experimental reproducibility?
Technical-grade this compound may contain unsaturated isomers or oxidation byproducts, altering reaction kinetics. Purify via vacuum distillation (boiling point: 330–340°C) or column chromatography using silica gel. Verify purity via gas chromatography-mass spectrometry (GC-MS) and adjust stoichiometry in protocols to account for active amine content .
Q. What safety protocols are critical when handling this compound in laboratory settings?
Use nitrile gloves, chemical goggles, and fume hoods to avoid dermal/ocular exposure. Store in airtight containers at –20°C to prevent oxidation. In case of spills, absorb with inert materials (e.g., vermiculite) and neutralize with dilute acetic acid. Refer to SDS guidelines for emergency procedures .
Q. Which solvents are compatible with this compound for colloidal synthesis?
this compound is miscible with nonpolar solvents (hexane, toluene) but immiscible with polar solvents (water, ethanol). For phase transfer, use ethanol as an antisolvent to precipitate nanoparticles. Solubility tests should precede synthesis to avoid phase separation .
Q. How can researchers purify this compound-contaminated reaction byproducts?
Separate nanoparticles via centrifugation (10,000–15,000 rpm, 20 min) and wash repeatedly with ethanol/hexane mixtures. Analyze supernatant purity using UV-Vis spectroscopy to confirm this compound removal .
Advanced Research Questions
Q. How to resolve contradictory reports on this compound’s role in controlling nanoparticle size distributions?
Discrepancies arise from varying precursor-to-oleylamine ratios, heating rates, or oxidation levels. Systematically vary this compound concentration (0.1–0.5 M) and monitor size via TEM. For FePt, a 1:1 molar ratio of Fe:Pt with 20% this compound yields 5 nm particles (±0.25 nm). Cross-validate with dynamic light scattering (DLS) and small-angle X-ray scattering (SAXS) .
Q. What advanced techniques characterize this compound’s surface-binding mechanisms in nanocrystal synthesis?
Use Fourier-transform infrared spectroscopy (FTIR) to identify amine-metal coordination (peaks at 1,640 cm⁻¹ for C=N stretching). X-ray photoelectron spectroscopy (XPS) quantifies surface ligand density, while thermogravimetric analysis (TGA) measures decomposition kinetics (weight loss at 250–400°C) .
Q. How to experimentally distinguish this compound’s catalytic vs. templating roles in anisotropic nanoparticle growth?
Conduct control experiments with alternative surfactants (e.g., octadecylamine) and track growth kinetics via in-situ UV-Vis. For gold nanorods, this compound’s selective binding to {100} facets induces anisotropic growth, confirmed by high-resolution TEM (HRTEM) and electron diffraction .
Q. What methodologies assess this compound’s thermal stability in high-temperature syntheses?
Perform TGA under nitrogen to determine decomposition onset (typically 200°C). Couple with differential scanning calorimetry (DSC) to identify exothermic/endothermic events. For prolonged stability, use reflux systems with condensers to recycle evaporated this compound .
Q. How to optimize this compound-based reaction conditions using design of experiments (DOE)?
Apply a fractional factorial design to test variables: temperature (180–300°C), time (1–24 h), and this compound concentration (0.05–0.5 M). Use response surface methodology (RSM) to model particle size and crystallinity. Validate with X-ray diffraction (XRD) and energy-dispersive X-ray spectroscopy (EDS) .
Eigenschaften
IUPAC Name |
(Z)-octadec-9-en-1-amine | |
---|---|---|
Source | PubChem | |
URL | https://pubchem.ncbi.nlm.nih.gov | |
Description | Data deposited in or computed by PubChem | |
InChI |
InChI=1S/C18H37N/c1-2-3-4-5-6-7-8-9-10-11-12-13-14-15-16-17-18-19/h9-10H,2-8,11-19H2,1H3/b10-9- | |
Source | PubChem | |
URL | https://pubchem.ncbi.nlm.nih.gov | |
Description | Data deposited in or computed by PubChem | |
InChI Key |
QGLWBTPVKHMVHM-KTKRTIGZSA-N | |
Source | PubChem | |
URL | https://pubchem.ncbi.nlm.nih.gov | |
Description | Data deposited in or computed by PubChem | |
Canonical SMILES |
CCCCCCCCC=CCCCCCCCCN | |
Source | PubChem | |
URL | https://pubchem.ncbi.nlm.nih.gov | |
Description | Data deposited in or computed by PubChem | |
Isomeric SMILES |
CCCCCCCC/C=C\CCCCCCCCN | |
Source | PubChem | |
URL | https://pubchem.ncbi.nlm.nih.gov | |
Description | Data deposited in or computed by PubChem | |
Molecular Formula |
C18H37N | |
Source | PubChem | |
URL | https://pubchem.ncbi.nlm.nih.gov | |
Description | Data deposited in or computed by PubChem | |
DSSTOX Substance ID |
DTXSID8026933 | |
Record name | (Z)-9-Octadecenylamine | |
Source | EPA DSSTox | |
URL | https://comptox.epa.gov/dashboard/DTXSID8026933 | |
Description | DSSTox provides a high quality public chemistry resource for supporting improved predictive toxicology. | |
Molecular Weight |
267.5 g/mol | |
Source | PubChem | |
URL | https://pubchem.ncbi.nlm.nih.gov | |
Description | Data deposited in or computed by PubChem | |
Physical Description |
Liquid, Liquid; mp = 10-20 deg C; [IUCLID] Light yellow pasty liquid with an amine-like odor (mp = 15-30 deg C); [ECHA] Slightly beige paste; [MSDSonline] | |
Record name | 9-Octadecen-1-amine, (9Z)- | |
Source | EPA Chemicals under the TSCA | |
URL | https://www.epa.gov/chemicals-under-tsca | |
Description | EPA Chemicals under the Toxic Substances Control Act (TSCA) collection contains information on chemicals and their regulations under TSCA, including non-confidential content from the TSCA Chemical Substance Inventory and Chemical Data Reporting. | |
Record name | cis-9-Octadecenylamine | |
Source | Haz-Map, Information on Hazardous Chemicals and Occupational Diseases | |
URL | https://haz-map.com/Agents/4437 | |
Description | Haz-Map® is an occupational health database designed for health and safety professionals and for consumers seeking information about the adverse effects of workplace exposures to chemical and biological agents. | |
Explanation | Copyright (c) 2022 Haz-Map(R). All rights reserved. Unless otherwise indicated, all materials from Haz-Map are copyrighted by Haz-Map(R). No part of these materials, either text or image may be used for any purpose other than for personal use. Therefore, reproduction, modification, storage in a retrieval system or retransmission, in any form or by any means, electronic, mechanical or otherwise, for reasons other than personal use, is strictly prohibited without prior written permission. | |
Vapor Pressure |
0.0001 [mmHg] | |
Record name | cis-9-Octadecenylamine | |
Source | Haz-Map, Information on Hazardous Chemicals and Occupational Diseases | |
URL | https://haz-map.com/Agents/4437 | |
Description | Haz-Map® is an occupational health database designed for health and safety professionals and for consumers seeking information about the adverse effects of workplace exposures to chemical and biological agents. | |
Explanation | Copyright (c) 2022 Haz-Map(R). All rights reserved. Unless otherwise indicated, all materials from Haz-Map are copyrighted by Haz-Map(R). No part of these materials, either text or image may be used for any purpose other than for personal use. Therefore, reproduction, modification, storage in a retrieval system or retransmission, in any form or by any means, electronic, mechanical or otherwise, for reasons other than personal use, is strictly prohibited without prior written permission. | |
CAS No. |
112-90-3, 1838-19-3 | |
Record name | Oleylamine | |
Source | CAS Common Chemistry | |
URL | https://commonchemistry.cas.org/detail?cas_rn=112-90-3 | |
Description | CAS Common Chemistry is an open community resource for accessing chemical information. Nearly 500,000 chemical substances from CAS REGISTRY cover areas of community interest, including common and frequently regulated chemicals, and those relevant to high school and undergraduate chemistry classes. This chemical information, curated by our expert scientists, is provided in alignment with our mission as a division of the American Chemical Society. | |
Explanation | The data from CAS Common Chemistry is provided under a CC-BY-NC 4.0 license, unless otherwise stated. | |
Record name | cis-9-Octadecenylamine | |
Source | ChemIDplus | |
URL | https://pubchem.ncbi.nlm.nih.gov/substance/?source=chemidplus&sourceid=0000112903 | |
Description | ChemIDplus is a free, web search system that provides access to the structure and nomenclature authority files used for the identification of chemical substances cited in National Library of Medicine (NLM) databases, including the TOXNET system. | |
Record name | 9-Octadecenylamine | |
Source | DTP/NCI | |
URL | https://dtp.cancer.gov/dtpstandard/servlet/dwindex?searchtype=NSC&outputformat=html&searchlist=66461 | |
Description | The NCI Development Therapeutics Program (DTP) provides services and resources to the academic and private-sector research communities worldwide to facilitate the discovery and development of new cancer therapeutic agents. | |
Explanation | Unless otherwise indicated, all text within NCI products is free of copyright and may be reused without our permission. Credit the National Cancer Institute as the source. | |
Record name | 9-Octadecen-1-amine, (9Z)- | |
Source | EPA Chemicals under the TSCA | |
URL | https://www.epa.gov/chemicals-under-tsca | |
Description | EPA Chemicals under the Toxic Substances Control Act (TSCA) collection contains information on chemicals and their regulations under TSCA, including non-confidential content from the TSCA Chemical Substance Inventory and Chemical Data Reporting. | |
Record name | (Z)-9-Octadecenylamine | |
Source | EPA DSSTox | |
URL | https://comptox.epa.gov/dashboard/DTXSID8026933 | |
Description | DSSTox provides a high quality public chemistry resource for supporting improved predictive toxicology. | |
Record name | (Z)-octadec-9-enylamine | |
Source | European Chemicals Agency (ECHA) | |
URL | https://echa.europa.eu/substance-information/-/substanceinfo/100.003.650 | |
Description | The European Chemicals Agency (ECHA) is an agency of the European Union which is the driving force among regulatory authorities in implementing the EU's groundbreaking chemicals legislation for the benefit of human health and the environment as well as for innovation and competitiveness. | |
Explanation | Use of the information, documents and data from the ECHA website is subject to the terms and conditions of this Legal Notice, and subject to other binding limitations provided for under applicable law, the information, documents and data made available on the ECHA website may be reproduced, distributed and/or used, totally or in part, for non-commercial purposes provided that ECHA is acknowledged as the source: "Source: European Chemicals Agency, http://echa.europa.eu/". Such acknowledgement must be included in each copy of the material. ECHA permits and encourages organisations and individuals to create links to the ECHA website under the following cumulative conditions: Links can only be made to webpages that provide a link to the Legal Notice page. | |
Record name | OLEAMINE | |
Source | FDA Global Substance Registration System (GSRS) | |
URL | https://gsrs.ncats.nih.gov/ginas/app/beta/substances/ZDQ1JWQ8DT | |
Description | The FDA Global Substance Registration System (GSRS) enables the efficient and accurate exchange of information on what substances are in regulated products. Instead of relying on names, which vary across regulatory domains, countries, and regions, the GSRS knowledge base makes it possible for substances to be defined by standardized, scientific descriptions. | |
Explanation | Unless otherwise noted, the contents of the FDA website (www.fda.gov), both text and graphics, are not copyrighted. They are in the public domain and may be republished, reprinted and otherwise used freely by anyone without the need to obtain permission from FDA. Credit to the U.S. Food and Drug Administration as the source is appreciated but not required. | |
Record name | CIS-9-OCTADECENYLAMINE | |
Source | Hazardous Substances Data Bank (HSDB) | |
URL | https://pubchem.ncbi.nlm.nih.gov/source/hsdb/5579 | |
Description | The Hazardous Substances Data Bank (HSDB) is a toxicology database that focuses on the toxicology of potentially hazardous chemicals. It provides information on human exposure, industrial hygiene, emergency handling procedures, environmental fate, regulatory requirements, nanomaterials, and related areas. The information in HSDB has been assessed by a Scientific Review Panel. | |
Synthesis routes and methods I
Procedure details
Synthesis routes and methods II
Procedure details
Retrosynthesis Analysis
AI-Powered Synthesis Planning: Our tool employs the Template_relevance Pistachio, Template_relevance Bkms_metabolic, Template_relevance Pistachio_ringbreaker, Template_relevance Reaxys, Template_relevance Reaxys_biocatalysis model, leveraging a vast database of chemical reactions to predict feasible synthetic routes.
One-Step Synthesis Focus: Specifically designed for one-step synthesis, it provides concise and direct routes for your target compounds, streamlining the synthesis process.
Accurate Predictions: Utilizing the extensive PISTACHIO, BKMS_METABOLIC, PISTACHIO_RINGBREAKER, REAXYS, REAXYS_BIOCATALYSIS database, our tool offers high-accuracy predictions, reflecting the latest in chemical research and data.
Strategy Settings
Precursor scoring | Relevance Heuristic |
---|---|
Min. plausibility | 0.01 |
Model | Template_relevance |
Template Set | Pistachio/Bkms_metabolic/Pistachio_ringbreaker/Reaxys/Reaxys_biocatalysis |
Top-N result to add to graph | 6 |
Feasible Synthetic Routes
Haftungsausschluss und Informationen zu In-Vitro-Forschungsprodukten
Bitte beachten Sie, dass alle Artikel und Produktinformationen, die auf BenchChem präsentiert werden, ausschließlich zu Informationszwecken bestimmt sind. Die auf BenchChem zum Kauf angebotenen Produkte sind speziell für In-vitro-Studien konzipiert, die außerhalb lebender Organismen durchgeführt werden. In-vitro-Studien, abgeleitet von dem lateinischen Begriff "in Glas", beinhalten Experimente, die in kontrollierten Laborumgebungen unter Verwendung von Zellen oder Geweben durchgeführt werden. Es ist wichtig zu beachten, dass diese Produkte nicht als Arzneimittel oder Medikamente eingestuft sind und keine Zulassung der FDA für die Vorbeugung, Behandlung oder Heilung von medizinischen Zuständen, Beschwerden oder Krankheiten erhalten haben. Wir müssen betonen, dass jede Form der körperlichen Einführung dieser Produkte in Menschen oder Tiere gesetzlich strikt untersagt ist. Es ist unerlässlich, sich an diese Richtlinien zu halten, um die Einhaltung rechtlicher und ethischer Standards in Forschung und Experiment zu gewährleisten.