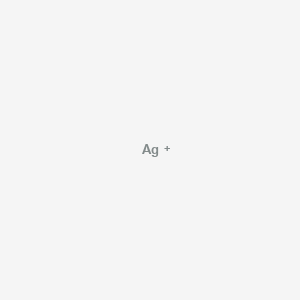
Silver cation
- Klicken Sie auf QUICK INQUIRY, um ein Angebot von unserem Expertenteam zu erhalten.
- Mit qualitativ hochwertigen Produkten zu einem WETTBEWERBSFÄHIGEN Preis können Sie sich mehr auf Ihre Forschung konzentrieren.
Übersicht
Beschreibung
The silver cation (Ag⁺) is a monovalent ion with a +1 charge, characterized by an ionic radius of ~1.15 Å . It exhibits exceptional coordination chemistry, forming stable complexes with ligands such as sulfur, nitrogen, and oxygen donors . Silver cations are widely utilized in medical applications (e.g., silver sulfadiazine for burns) due to their potent antimicrobial properties . Their pharmacokinetics, including absorption and excretion, are well-documented, enabling targeted therapeutic use . Structurally, Ag⁺ adopts linear, trigonal, or tetrahedral geometries in complexes, influenced by ligand field effects and relativistic contraction of electron orbitals .
Vorbereitungsmethoden
Synthetic Routes and Reaction Conditions: Silver cations can be prepared through several methods, including chemical reduction, electrochemical processes, and biological approaches. One common method involves the reduction of silver nitrate (AgNO₃) using reducing agents such as sodium borohydride (NaBH₄) or hydrazine (N₂H₄). The reaction typically occurs in an aqueous solution under controlled temperature and pH conditions .
Industrial Production Methods: In industrial settings, silver cations are often produced through the electrolysis of silver-containing solutions. This process involves passing an electric current through the solution, causing the silver ions to migrate to the cathode, where they are reduced to metallic silver. The metallic silver can then be oxidized back to silver cations as needed .
Types of Reactions:
Oxidation: Silver cations can undergo oxidation reactions, where they are converted to higher oxidation states. this is less common due to the stability of the Ag⁺ ion.
Substitution: Silver cations can participate in substitution reactions, where they replace other cations in compounds.
Common Reagents and Conditions:
Hydrochloric Acid (HCl): Reacts with silver nitrate to form silver chloride.
Ammonia (NH₃): Forms a complex with silver cations, which can be used to dissolve silver chloride.
Sodium Hydroxide (NaOH): Precipitates silver oxide from silver cations.
Major Products Formed:
Silver Chloride (AgCl): Formed from the reaction of silver nitrate with hydrochloric acid.
Silver Oxide (Ag₂O): Formed from the reaction of silver cations with sodium hydroxide.
Wissenschaftliche Forschungsanwendungen
Medical Applications
Antimicrobial Properties
Silver cations are renowned for their potent antimicrobial effects. They disrupt bacterial cell membranes, leading to cell death. Studies have shown that concentrations as low as 15 parts per billion can effectively kill bacteria, making silver ions significantly more toxic than silver nanoparticles .
Case Study: Wound Healing
In clinical settings, silver sulfadiazine cream, which releases silver ions, is commonly used for treating burns and preventing infections. A study highlighted that patients treated with silver dressings exhibited faster healing rates compared to those receiving standard care .
Cancer Therapy
Recent research indicates that silver cations can enhance the efficacy of chemotherapy drugs. In vitro studies demonstrated that combining silver ions with certain chemotherapeutics resulted in increased cytotoxicity against cancer cells .
Application | Mechanism of Action | Example Products |
---|---|---|
Antimicrobial | Disruption of cell membranes | Silver sulfadiazine |
Wound healing | Promotes tissue regeneration | Silver dressings |
Cancer therapy | Enhances drug efficacy | Silver ion complexes with chemotherapeutics |
Nanotechnology
Synthesis of Silver Nanoparticles (AgNPs)
Silver cations serve as precursors in the synthesis of silver nanoparticles, which have unique optical and electronic properties. Various methods, including chemical reduction and biosynthesis using plant extracts, are employed to produce AgNPs .
Applications in Electronics
Silver nanoparticles are utilized in creating conductive inks for printed electronics and sensors due to their excellent conductivity and stability. They are also used in photovoltaic cells to enhance efficiency by improving light absorption .
Case Study: Antibacterial Coatings
AgNPs are incorporated into coatings for medical devices and textiles to prevent biofilm formation and reduce infection rates. A study showed that catheters coated with silver nanoparticles significantly reduced the incidence of catheter-associated urinary tract infections .
Application | Method of Synthesis | Benefits |
---|---|---|
Electronics | Chemical reduction | Improved conductivity |
Antibacterial coatings | Biosynthesis or chemical methods | Reduced infection rates |
Environmental Applications
Water Purification
Silver cations are effective in water treatment processes due to their ability to kill bacteria and viruses. They can be used in filters or as additives in water purification systems .
Case Study: Wastewater Treatment
In wastewater treatment plants, silver ions have been tested as a biocide to control microbial growth in sludge systems. Results indicated a significant reduction in microbial load, enhancing overall treatment efficiency .
Application | Mechanism | Effectiveness |
---|---|---|
Water purification | Disinfection of pathogens | High efficacy against bacteria |
Wastewater treatment | Control of microbial growth | Enhanced treatment efficiency |
Wirkmechanismus
The antimicrobial activity of silver cations is primarily due to their ability to disrupt bacterial cell membranes. Silver cations bind to proteins and enzymes in the bacterial cell membrane, causing structural damage and inhibiting essential cellular processes. This leads to the formation of reactive oxygen species (ROS), which further damage cellular components and ultimately result in cell death . Additionally, silver cations can bind to bacterial DNA, inhibiting replication and transcription .
Vergleich Mit ähnlichen Verbindungen
Comparison with Similar Cations
Alkali Metal Cations (Na⁺, K⁺)
Ionic Radius and Collision Cross-Section (CCS):
In ion mobility spectrometry, CCS values of cation adducts generally increase with ionic radius (H⁺ < Li⁺ < Na⁺ < Ag⁺ < Cs⁺). However, Ag⁺ exhibits exceptions: For rutin (RUT), [RUT + Ag]⁺ adducts show CCS values (181.9–195.2 Ų) lower than [RUT + Li]⁺ (187.4 Ų) in certain conformations, highlighting Ag⁺’s unique binding geometry .
Solubility: Silver salts like AgCl are typically insoluble, contrasting with soluble alkali metal salts (e.g., NaCl). Exceptions include AgNO₃, which is highly soluble, enabling its use in aqueous formulations .
Medical Applications:
Ag⁺-based compounds (e.g., silver nitrate) are preferred for topical antimicrobial applications, whereas Na⁺/K⁺ salts (e.g., NaCl) lack biocidal efficacy .
Table 1: Ionic Radius and CCS Trends
Cation | Ionic Radius (Å) | Average CCS (Ų) | Notable Exceptions |
---|---|---|---|
H⁺ | 0.0001 | 160.8 | – |
Li⁺ | 0.76 | 162.1 | – |
Na⁺ | 0.95 | 165.0 | – |
Ag⁺ | 1.15 | 181.9–195.2 | Lower CCS than Li⁺ in RUT |
Cs⁺ | 1.67 | 192.8 | – |
Transition Metal Cations (Cu²⁺, Zn²⁺)
Coordination Chemistry:
Ag⁺ prefers linear coordination (e.g., [Ag(NH₃)₂]⁺), whereas Cu²⁺ and Zn²⁺ favor square planar or tetrahedral geometries. Silver’s relativistic effects enhance 4d-orbital stabilization, reducing redox activity compared to Cu²⁺, which participates in Fenton reactions .
Antimicrobial Mechanisms: Ag⁺ disrupts bacterial membranes via thiol-group binding, while Cu²⁺ generates reactive oxygen species (ROS). Zinc compounds (e.g., ZnO) act primarily as physical barriers .
Other Group 11 Cations (Au⁺)
Electronic Structure:
Ag⁺ and Au⁺ share a +1 charge, but Au⁺ exhibits stronger relativistic effects, leading to higher electronegativity and affinity for soft ligands (e.g., thiols). Gold complexes (e.g., Au(I)-thiolates) are more stable than Ag⁺ analogues .
Catalytic Activity:
Ag⁺ is less catalytically active than Au⁺ in oxidation reactions. For example, gold/silver composites under ultrastrong coupling conditions show Au-dominated water oxidation activity .
Structural and Crystallographic Comparisons
Schottky Defects:
Ag⁺’s large ionic radius limits Schottky defect formation in crystals like AgI (Ag⁺: 1.15 Å, I⁻: 2.20 Å), unlike NaCl (Na⁺: 0.95 Å, Cl⁻: 1.81 Å), which readily forms defects .
Nanocrystal Interactions: In AgBiS₂ nanocrystals, Ag⁺ exhibits weaker interaction with sulfur after bismuth incorporation, evidenced by a 0.3 eV shift in S 2p binding energy compared to Ag₂S .
Data Tables
Table 3: Solubility Comparison
Salt | Solubility in Water | Notes |
---|---|---|
AgNO₃ | Soluble | Common precursor in synthesis |
AgCl | Insoluble | Precipitates in halide tests |
NaCl | Soluble | Baseline for alkali metals |
CuSO₄ | Soluble | Transition metal exception |
Biologische Aktivität
Silver cations (Ag+) have garnered significant attention in biomedical research due to their broad-spectrum antimicrobial properties and diverse biological activities. This article explores the mechanisms of action, case studies, and research findings related to the biological activity of silver cations, supported by data tables and relevant studies.
Silver cations exert their antimicrobial effects through multiple mechanisms:
- Cell Membrane Disruption : Silver ions interact with bacterial cell membranes, leading to increased permeability and eventual cell lysis. This interaction can alter membrane proteins involved in cell wall synthesis, disrupting cellular integrity .
- Reactive Oxygen Species (ROS) Generation : Silver cations promote the production of ROS within bacterial cells, which can damage cellular components such as DNA and proteins. This oxidative stress contributes to bacterial cell death .
- Binding to Cellular Components : Silver ions can bind to thiol groups in proteins, disrupting metabolic processes and inhibiting essential enzymatic functions. This binding affects various metabolic pathways, including glycolysis and respiration .
- Iron Homeostasis Disruption : Silver cations interfere with iron-sulfur clusters in bacteria, leading to the release of free iron (Fe2+), which is essential for various cellular processes .
Antimicrobial Efficacy Against Gram-Negative Bacteria
A study demonstrated that silver enhances the efficacy of existing antibiotics against Gram-negative bacteria. The research showed that silver disrupts disulfide bond formation and metabolism in bacteria, increasing their susceptibility to antibiotics .
- Table 1: Effect of Silver on Antibiotic Susceptibility
Antibiotic | Bacterial Strain | Minimum Inhibitory Concentration (MIC) with Silver (µg/mL) | MIC without Silver (µg/mL) |
---|---|---|---|
Ampicillin | E. coli | 0.5 | 8 |
Gentamicin | Pseudomonas aeruginosa | 1 | 32 |
Tetracycline | Klebsiella pneumoniae | 0.25 | 4 |
Toxicity Studies
Research indicates that silver ions are significantly more toxic to bacteria than silver nanoparticles. A study found that ionic silver at concentrations as low as 15 parts per billion could effectively kill bacteria, while silver nanoparticles were much less toxic at similar concentrations .
- Table 2: Comparative Toxicity of Silver Forms
Form of Silver | Concentration (ppm) | Toxicity Level |
---|---|---|
Silver Ions | 0.015 | Highly Toxic |
Silver Nanoparticles | 195 | Non-toxic |
Research Findings
Recent studies have elucidated the biological activity of silver cations through various experimental approaches:
- A study utilizing transmission electron microscopy (TEM) revealed that silver ions enter bacterial cells within 30 minutes, causing morphological changes such as cell lysis and cytoplasmic leakage .
- Another investigation demonstrated that silver cations could induce an "active but nonculturable" state in bacteria, where metabolic activity continues but growth halts due to stress from silver exposure .
Q & A
Q. What experimental methods are most reliable for detecting silver cations (Ag⁺) in aqueous solutions, and how do their detection limits and interferences compare?
(Basic)
Methodological Answer:
The detection of Ag⁺ in aqueous solutions commonly employs:
- Atomic Absorption Spectroscopy (AAS): Offers high sensitivity (detection limit ~0.1 ppb) but requires calibration with standard solutions and may suffer from matrix interferences in complex samples .
- Ion-Selective Electrodes (ISE): Provides real-time measurement (detection limit ~1 ppm) but is sensitive to competing ions like Hg²⁺ or Cu²⁺ .
- Colorimetric Methods (e.g., dithizone complexation): Cost-effective with visible color changes (detection limit ~10 ppm), but pH and ligand concentration must be tightly controlled to avoid false positives .
Table 1: Comparison of Detection Methods
Method | Detection Limit | Key Interferences | Optimal Use Case |
---|---|---|---|
AAS | 0.1 ppb | High salt matrices | Low-concentration samples |
ISE | 1 ppm | Hg²⁺, Cu²⁺ | Field measurements |
Colorimetric (dithizone) | 10 ppm | pH fluctuations, organic ligands | Qualitative screening |
Best Practice: Validate results with a secondary method (e.g., AAS + ISE) to mitigate method-specific biases .
Q. How should researchers design experiments to investigate the stability of silver cations under varying environmental conditions (e.g., pH, ionic strength)?
(Advanced)
Methodological Answer:
To study Ag⁺ stability:
Controlled Variables: Use buffered solutions (e.g., acetate buffers for pH 3–5, phosphate buffers for pH 6–8) to isolate pH effects. Maintain ionic strength with inert electrolytes like KNO₃ to avoid unintended complexation .
Time-Resolved Monitoring: Employ techniques like UV-Vis spectroscopy to track Ag⁺ speciation over time, especially in systems prone to oxidation (Ag⁺ → Ag⁰) .
Validation: Compare experimental results with thermodynamic models (e.g., using software like PHREEQC) to identify discrepancies between theoretical and observed stability .
Key Consideration: Document all protocol details (e.g., buffer composition, temperature) to ensure reproducibility .
Q. What methodological strategies resolve contradictions in reported stability constants (log K) of silver-ligand complexes?
(Advanced)
Methodological Answer:
Discrepancies in log K values often arise from:
- Ligand Purity: Impurities in organic ligands (e.g., humic acids) can skew results. Use HPLC-purified ligands and verify purity via NMR or mass spectrometry .
- Experimental Conditions: Variations in ionic strength or temperature alter complexation kinetics. Standardize conditions (e.g., I = 0.1 M NaCl, 25°C) across studies .
- Data Interpretation: Apply multivariate statistical analysis (e.g., principal component analysis) to isolate confounding factors in datasets .
Example: A 2020 study reconciled conflicting log K values for Ag⁺-EDTA complexes by re-evaluating data under uniform ionic strength, reducing variability from 0.5 to 0.1 log units .
Q. How can computational models complement experimental studies of silver cation interactions with biomolecules?
(Advanced)
Methodological Answer:
- Molecular Dynamics (MD) Simulations: Predict binding sites of Ag⁺ with proteins (e.g., albumin) by modeling electrostatic interactions and solvation effects. Validate predictions with spectroscopic data (e.g., circular dichroism) .
- Density Functional Theory (DFT): Calculate thermodynamic parameters (e.g., Gibbs free energy) for Ag⁺-DNA adduct formation, guiding experimental focus on energetically favorable pathways .
Best Practice: Cross-validate computational results with experimental techniques like ITC (isothermal titration calorimetry) to ensure accuracy .
Q. What are the critical considerations for designing a study on the antibacterial mechanisms of silver cations?
(Advanced)
Methodological Answer:
Model Organisms: Use standardized bacterial strains (e.g., E. coli ATCC 25922) to ensure comparability. Include controls for biofilm vs. planktonic cell responses .
Mechanistic Probes: Combine transcriptomics (RNA sequencing) and proteomics to identify Ag⁺-induced stress pathways. Pair with ROS (reactive oxygen species) assays to link molecular changes to physiological outcomes .
Concentration Gradients: Test sub-inhibitory to lethal Ag⁺ concentrations to differentiate bacteriostatic vs. bactericidal effects .
Pitfall Avoidance: Account for Ag⁺ nanoparticle dissolution kinetics if using colloidal silver, as free Ag⁺ concentration dictates antimicrobial activity .
Q. How can researchers address challenges in quantifying this compound speciation in heterogeneous environmental samples?
(Advanced)
Methodological Answer:
- Sequential Extraction: Use Tessier’s protocol to fractionate Ag⁺ bound to organic matter, sulfides, or mineral oxides .
- Synchrotron-Based XANES: Characterize Ag⁺ redox states (e.g., Ag⁺ vs. Ag⁰) in soil or sediment matrices with minimal sample preparation .
- Quality Control: Spike samples with isotopically enriched ¹⁰⁷Ag to correct for recovery losses during extraction .
Data Interpretation: Normalize results to total Ag content (via ICP-MS) to differentiate between soluble and particulate phases .
Eigenschaften
CAS-Nummer |
14701-21-4 |
---|---|
Molekularformel |
Ag+ |
Molekulargewicht |
107.868 g/mol |
IUPAC-Name |
silver(1+) |
InChI |
InChI=1S/Ag/q+1 |
InChI-Schlüssel |
FOIXSVOLVBLSDH-UHFFFAOYSA-N |
SMILES |
[Ag+] |
Kanonische SMILES |
[Ag+] |
melting_point |
960.5 °C |
Key on ui other cas no. |
14701-21-4 7440-22-4 |
Physikalische Beschreibung |
Solid |
Synonyme |
Silver, ion |
Herkunft des Produkts |
United States |
Haftungsausschluss und Informationen zu In-Vitro-Forschungsprodukten
Bitte beachten Sie, dass alle Artikel und Produktinformationen, die auf BenchChem präsentiert werden, ausschließlich zu Informationszwecken bestimmt sind. Die auf BenchChem zum Kauf angebotenen Produkte sind speziell für In-vitro-Studien konzipiert, die außerhalb lebender Organismen durchgeführt werden. In-vitro-Studien, abgeleitet von dem lateinischen Begriff "in Glas", beinhalten Experimente, die in kontrollierten Laborumgebungen unter Verwendung von Zellen oder Geweben durchgeführt werden. Es ist wichtig zu beachten, dass diese Produkte nicht als Arzneimittel oder Medikamente eingestuft sind und keine Zulassung der FDA für die Vorbeugung, Behandlung oder Heilung von medizinischen Zuständen, Beschwerden oder Krankheiten erhalten haben. Wir müssen betonen, dass jede Form der körperlichen Einführung dieser Produkte in Menschen oder Tiere gesetzlich strikt untersagt ist. Es ist unerlässlich, sich an diese Richtlinien zu halten, um die Einhaltung rechtlicher und ethischer Standards in Forschung und Experiment zu gewährleisten.