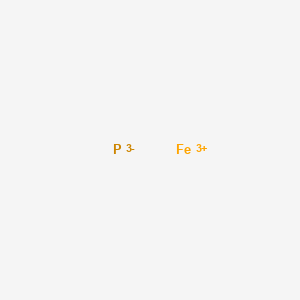
Ferriumphosphor(-3)-Anion
Übersicht
Beschreibung
Wissenschaftliche Forschungsanwendungen
Ferric phosphorus(-3) anion has a wide range of applications in scientific research:
Chemistry:
- Used as a precursor for the synthesis of other iron-phosphorus compounds.
- Employed in the study of phosphorus chemistry and its interactions with transition metals.
Biology:
- Investigated for its potential role in biological systems, particularly in iron and phosphorus metabolism.
Medicine:
- Explored for its potential use in drug delivery systems due to its unique chemical properties.
Industry:
- Utilized in the production of high-strength alloys and materials.
- Applied in the development of advanced ceramics and electronic components.
Wirkmechanismus
Target of Action
The primary targets of ferric phosphorus(-3) anion are hemoglobin, myoglobin, and specific enzyme systems . These targets are essential for the transport of oxygen in the body . In the context of soil management, phosphorus is often the most limiting plant nutrient, and the primary forms of phosphorus taken up by plants are the orthophosphates, H2PO4- and HPO42- .
Mode of Action
Ferric phosphorus(-3) anion supplies a source to elemental iron necessary to the function of hemoglobin, myoglobin, and specific enzyme systems . This allows for the transport of oxygen via hemoglobin .
Biochemical Pathways
The biochemical pathways affected by ferric phosphorus(-3) anion are those involved in the transport of oxygen via hemoglobin and myoglobin . In the context of soil management, phosphorus is involved in many plant processes, including energy transfer reactions, development of reproductive structures, crop maturity, root growth, and protein synthesis .
Pharmacokinetics
Related compounds such as ferric gluconate have a half-life elimination of bound iron of about 1 hour .
Result of Action
The result of the action of ferric phosphorus(-3) anion is the enhancement of the function of hemoglobin, myoglobin, and specific enzyme systems, thereby improving the transport of oxygen in the body . In the context of soil management, the availability of phosphorus can enhance various plant processes, including energy transfer reactions, development of reproductive structures, crop maturity, root growth, and protein synthesis .
Action Environment
The action of ferric phosphorus(-3) anion can be influenced by environmental factors. For instance, in the context of soil management, the presence of mycorrhizal fungi, which develop a symbiotic relationship with plant roots and extend threadlike hyphae into the soil, can enhance the uptake of phosphorus, especially in acidic soils that are low in phosphorus . Furthermore, the use of ferric oxyhydroxide loaded anion exchanger (FOAE) hybrid adsorbent, which consists of nanosized ferric oxyhydroxide (FO) on anion exchanger resin, has been shown to enhance the removal of phosphate from wastewater .
Biochemische Analyse
Biochemical Properties
Ferric Phosphorus(-3) Anion is integral to various biochemical processes . It exhibits paramagnetic behavior, meaning it is only magnetized in the presence of an external magnetic field, and is demagnetized when the field is removed . It interacts with various enzymes, proteins, and other biomolecules, influencing their function and activity .
Cellular Effects
The effects of Ferric Phosphorus(-3) Anion on cells are diverse. It influences cell function, including impact on cell signaling pathways, gene expression, and cellular metabolism . The specific effects can vary depending on the type of cell and the concentration of Ferric Phosphorus(-3) Anion present.
Molecular Mechanism
The molecular mechanism of Ferric Phosphorus(-3) Anion involves its interactions at the molecular level. It can bind with biomolecules, inhibit or activate enzymes, and induce changes in gene expression . The exact mechanism of action can vary depending on the specific biochemical context.
Temporal Effects in Laboratory Settings
In laboratory settings, the effects of Ferric Phosphorus(-3) Anion can change over time. It has been observed that ferric chloride can effectively inhibit the release of phosphorus from sediments . This suggests that Ferric Phosphorus(-3) Anion may have long-term effects on cellular function in in vitro or in vivo studies.
Metabolic Pathways
Ferric Phosphorus(-3) Anion is involved in various metabolic pathways. It interacts with enzymes and cofactors, and can influence metabolic flux or metabolite levels . The specific pathways and effects can vary depending on the biochemical context.
Transport and Distribution
Ferric Phosphorus(-3) Anion is transported and distributed within cells and tissues. It can interact with transporters or binding proteins, influencing its localization or accumulation . The specific transport and distribution mechanisms can vary depending on the type of cell and the biochemical context.
Subcellular Localization
The subcellular localization of Ferric Phosphorus(-3) Anion can influence its activity or function. It may be directed to specific compartments or organelles by targeting signals or post-translational modifications . The specific localization and effects can vary depending on the type of cell and the biochemical context.
Vorbereitungsmethoden
Synthetic Routes and Reaction Conditions: Ferric phosphorus(-3) anion can be synthesized through several methods. One common method involves the direct combination of iron and phosphorus at high temperatures. The reaction is typically carried out in an inert atmosphere to prevent oxidation:
2Fe+P4→2FeP
Another method involves the reduction of ferric phosphate with a reducing agent such as hydrogen gas:
FePO4+4H2→FeP+4H2O
Industrial Production Methods: In industrial settings, ferric phosphorus(-3) anion is often produced through the carbothermal reduction of ferric phosphate. This process involves heating a mixture of ferric phosphate and carbon in a high-temperature furnace:
FePO4+3C→FeP+3CO
The reaction is carried out at temperatures above 1000°C to ensure complete reduction.
Analyse Chemischer Reaktionen
Types of Reactions: Ferric phosphorus(-3) anion undergoes various chemical reactions, including oxidation, reduction, and substitution reactions.
Oxidation: Ferric phosphorus(-3) anion can be oxidized to form ferric phosphate:
2FeP+5O2→2FePO4
Reduction: Ferric phosphorus(-3) anion can be reduced to elemental iron and phosphorus:
FeP+H2→Fe+PH3
Substitution: Ferric phosphorus(-3) anion can undergo substitution reactions with halogens to form ferric halides and phosphorus halides:
FeP+3Cl2→FeCl3+PCl3
Common Reagents and Conditions:
- Oxidizing agents: Oxygen, chlorine
- Reducing agents: Hydrogen gas, carbon
- Reaction conditions: High temperatures, inert atmosphere
Major Products:
- Ferric phosphate (FePO_4)
- Elemental iron (Fe)
- Phosphine (PH_3)
- Ferric chloride (FeCl_3)
- Phosphorus trichloride (PCl_3)
Vergleich Mit ähnlichen Verbindungen
- Ferric phosphate (FePO_4)
- Ferrous phosphide (Fe_2P)
- Iron phosphide (Fe_3P)
Biologische Aktivität
Ferric phosphorus(-3) anion, also known as ferric phosphate, is a compound of significant interest in biological and environmental chemistry due to its roles in various biochemical processes and its applications in wastewater treatment. This article explores the biological activity of ferric phosphorus(-3) anion, focusing on its mechanisms of action, effects on microbial communities, and implications for phosphorus removal in environmental systems.
Ferric phosphorus(-3) anion plays a crucial role in iron transport and metabolism, particularly in microbial systems. The interaction between ferric ions (Fe³⁺) and phosphate ions (PO₄³⁻) is essential for various biological processes:
- Iron Complexation : Ferric binding proteins (Fbp) facilitate the transport of Fe³⁺ across bacterial membranes. The presence of phosphate enhances the stability of these complexes, which is vital for iron sequestration and transport in Gram-negative bacteria .
- Reactive Oxygen Species (ROS) Generation : The Fenton reaction, involving Fe²⁺ and hydrogen peroxide (H₂O₂), can lead to the production of highly reactive hydroxyl radicals (•OH). These ROS play a dual role in cellular signaling and oxidative stress, impacting cell survival and function .
2. Effects on Microbial Communities
The addition of ferric ions can significantly alter microbial dynamics within ecosystems:
- Phosphorus Removal Mechanisms : In activated sludge systems, prolonged exposure to Fe(III) can inhibit phosphorus removal efficiency. High concentrations of Fe(III) lead to changes in microbial community structure, favoring certain phosphorus-accumulating organisms while inhibiting others . For instance, the abundance of Candidatus Accumulibacter, a key player in enhanced biological phosphorus removal (EBPR), was affected by Fe(III) stress.
- Metabolic Shifts : Studies indicate that under Fe(III) stress, microorganisms may adapt their metabolic pathways to cope with altered phosphorus availability. This includes changes in the activities of key enzymes involved in polyphosphate metabolism .
3.1 Phosphorus Removal Efficacy
A study investigating the efficacy of ferric ions in removing total phosphorus (T-P) from wastewater found that ferric chloride (FeCl₃) demonstrated superior T-P removal efficiency compared to ferrous solutions (Fe²⁺). Specifically:
Chemical | T-P Removal Efficiency (%) |
---|---|
FeCl₃ | 37.4 |
Fe(NO₃)₃ | 34.4 |
FeCl₂ | 6.8 |
FeSO₄ | 9.5 |
This highlights the importance of ferric ions over their ferrous counterparts in enhancing phosphorus removal processes .
3.2 Impact on Microbial Metabolism
Research has shown that chronic exposure to high levels of Fe(III) leads to a decrease in intracellular phosphorus storage within activated sludge systems. For example:
- Intracellular phosphorus levels decreased from 7.67 mg/g VSS to as low as 3.11 mg/g VSS under high Fe(III) conditions.
- Functional gene abundance associated with polyphosphate metabolism decreased, indicating a shift in microbial metabolic strategies to adapt to iron-induced stress .
4. Conclusion
Ferric phosphorus(-3) anion plays a multifaceted role in biological systems, influencing iron transport, microbial community dynamics, and phosphorus removal mechanisms. Its ability to generate reactive oxygen species further complicates its biological activity, impacting both cellular signaling and oxidative stress responses.
Future research should focus on elucidating the precise biochemical pathways influenced by ferric phosphorus(-3) anion and exploring its potential applications in bioremediation and wastewater treatment technologies.
Eigenschaften
IUPAC Name |
iron(3+);phosphorus(3-) | |
---|---|---|
Source | PubChem | |
URL | https://pubchem.ncbi.nlm.nih.gov | |
Description | Data deposited in or computed by PubChem | |
InChI |
InChI=1S/Fe.P/q+3;-3 | |
Source | PubChem | |
URL | https://pubchem.ncbi.nlm.nih.gov | |
Description | Data deposited in or computed by PubChem | |
InChI Key |
JNRQHASIGICJBU-UHFFFAOYSA-N | |
Source | PubChem | |
URL | https://pubchem.ncbi.nlm.nih.gov | |
Description | Data deposited in or computed by PubChem | |
Canonical SMILES |
[P-3].[Fe+3] | |
Source | PubChem | |
URL | https://pubchem.ncbi.nlm.nih.gov | |
Description | Data deposited in or computed by PubChem | |
Molecular Formula |
FeP | |
Source | PubChem | |
URL | https://pubchem.ncbi.nlm.nih.gov | |
Description | Data deposited in or computed by PubChem | |
Molecular Weight |
86.82 g/mol | |
Source | PubChem | |
URL | https://pubchem.ncbi.nlm.nih.gov | |
Description | Data deposited in or computed by PubChem | |
Physical Description |
Dry Powder | |
Record name | Iron phosphide | |
Source | EPA Chemicals under the TSCA | |
URL | https://www.epa.gov/chemicals-under-tsca | |
Description | EPA Chemicals under the Toxic Substances Control Act (TSCA) collection contains information on chemicals and their regulations under TSCA, including non-confidential content from the TSCA Chemical Substance Inventory and Chemical Data Reporting. | |
CAS No. |
12023-53-9, 12751-22-3 | |
Record name | Iron phosphide (Fe3P) | |
Source | ChemIDplus | |
URL | https://pubchem.ncbi.nlm.nih.gov/substance/?source=chemidplus&sourceid=0012023539 | |
Description | ChemIDplus is a free, web search system that provides access to the structure and nomenclature authority files used for the identification of chemical substances cited in National Library of Medicine (NLM) databases, including the TOXNET system. | |
Record name | Iron phosphide | |
Source | ChemIDplus | |
URL | https://pubchem.ncbi.nlm.nih.gov/substance/?source=chemidplus&sourceid=0012751223 | |
Description | ChemIDplus is a free, web search system that provides access to the structure and nomenclature authority files used for the identification of chemical substances cited in National Library of Medicine (NLM) databases, including the TOXNET system. | |
Record name | Iron phosphide | |
Source | EPA Chemicals under the TSCA | |
URL | https://www.epa.gov/chemicals-under-tsca | |
Description | EPA Chemicals under the Toxic Substances Control Act (TSCA) collection contains information on chemicals and their regulations under TSCA, including non-confidential content from the TSCA Chemical Substance Inventory and Chemical Data Reporting. | |
Record name | Iron phosphide | |
Source | European Chemicals Agency (ECHA) | |
URL | https://echa.europa.eu/substance-information/-/substanceinfo/100.032.531 | |
Description | The European Chemicals Agency (ECHA) is an agency of the European Union which is the driving force among regulatory authorities in implementing the EU's groundbreaking chemicals legislation for the benefit of human health and the environment as well as for innovation and competitiveness. | |
Explanation | Use of the information, documents and data from the ECHA website is subject to the terms and conditions of this Legal Notice, and subject to other binding limitations provided for under applicable law, the information, documents and data made available on the ECHA website may be reproduced, distributed and/or used, totally or in part, for non-commercial purposes provided that ECHA is acknowledged as the source: "Source: European Chemicals Agency, http://echa.europa.eu/". Such acknowledgement must be included in each copy of the material. ECHA permits and encourages organisations and individuals to create links to the ECHA website under the following cumulative conditions: Links can only be made to webpages that provide a link to the Legal Notice page. | |
Record name | Triiron phosphide | |
Source | European Chemicals Agency (ECHA) | |
URL | https://echa.europa.eu/substance-information/-/substanceinfo/100.031.517 | |
Description | The European Chemicals Agency (ECHA) is an agency of the European Union which is the driving force among regulatory authorities in implementing the EU's groundbreaking chemicals legislation for the benefit of human health and the environment as well as for innovation and competitiveness. | |
Explanation | Use of the information, documents and data from the ECHA website is subject to the terms and conditions of this Legal Notice, and subject to other binding limitations provided for under applicable law, the information, documents and data made available on the ECHA website may be reproduced, distributed and/or used, totally or in part, for non-commercial purposes provided that ECHA is acknowledged as the source: "Source: European Chemicals Agency, http://echa.europa.eu/". Such acknowledgement must be included in each copy of the material. ECHA permits and encourages organisations and individuals to create links to the ECHA website under the following cumulative conditions: Links can only be made to webpages that provide a link to the Legal Notice page. | |
Haftungsausschluss und Informationen zu In-Vitro-Forschungsprodukten
Bitte beachten Sie, dass alle Artikel und Produktinformationen, die auf BenchChem präsentiert werden, ausschließlich zu Informationszwecken bestimmt sind. Die auf BenchChem zum Kauf angebotenen Produkte sind speziell für In-vitro-Studien konzipiert, die außerhalb lebender Organismen durchgeführt werden. In-vitro-Studien, abgeleitet von dem lateinischen Begriff "in Glas", beinhalten Experimente, die in kontrollierten Laborumgebungen unter Verwendung von Zellen oder Geweben durchgeführt werden. Es ist wichtig zu beachten, dass diese Produkte nicht als Arzneimittel oder Medikamente eingestuft sind und keine Zulassung der FDA für die Vorbeugung, Behandlung oder Heilung von medizinischen Zuständen, Beschwerden oder Krankheiten erhalten haben. Wir müssen betonen, dass jede Form der körperlichen Einführung dieser Produkte in Menschen oder Tiere gesetzlich strikt untersagt ist. Es ist unerlässlich, sich an diese Richtlinien zu halten, um die Einhaltung rechtlicher und ethischer Standards in Forschung und Experiment zu gewährleisten.