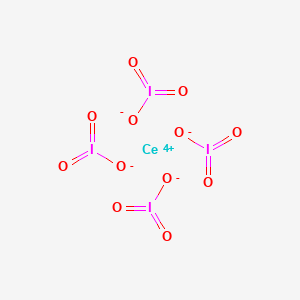
Ceric iodate
Übersicht
Beschreibung
Ceric iodate, a compound with the formula Ce(IO₃)₄, consists of cerium in its +4 oxidation state (Ce⁴⁺) and iodate (IO₃⁻) anions. It is primarily utilized in analytical and nuclear chemistry for separation and purification processes. For instance, in radiocerium determination, this compound is precipitated to isolate cerium from fission products, followed by redox cycles involving reduction to Ce³⁺ and scavenging impurities via zirconium iodate . Its strong oxidizing properties, derived from Ce⁴⁺, make it effective in redox-based purification workflows. However, its solubility is likely low, as evidenced by its use in precipitation methods .
Vorbereitungsmethoden
Ceric iodate can be synthesized through several methods, including hydrothermal synthesis. One common approach involves reacting cerium(IV) oxide with iodic acid under hydrothermal conditions. For example, cesium cerium(IV) iodate can be prepared by reacting cesium fluoride, cerium(IV) oxide, periodic acid, and iodic acid at 230°C . The resulting compound is then analyzed using techniques such as single crystal and powder X-ray diffraction, energy-dispersive X-ray spectroscopy, infrared spectroscopy, and thermal analysis .
Analyse Chemischer Reaktionen
Redox Reactions
Ceric iodate acts as a strong oxidizing agent due to the Ce⁴+/Ce³⁺ and IO₃⁻/I⁻ redox couples. Key reactions include:
Oxidation of Organic Compounds
In acidic media, this compound oxidizes organic substrates (e.g., malonic acid) via radical intermediates:
This reaction is critical in oscillating systems like the Briggs-Rauscher reaction, where Ce³⁺/Ce⁴⁺ cycling drives periodic iodine formation .
Reduction by Sulfite or Iodide
This compound undergoes stepwise reduction:
The reaction rate depends on [H⁺], with optimal activity below pH 2 .
Catalytic Decomposition of Hydrogen Peroxide
In the presence of H₂O₂, this compound facilitates oxygen evolution:
This property is exploited in redox flow batteries for energy storage .
Acid-Base Behavior
In strongly acidic solutions, this compound forms stable complexes:
Above pH 8, iodate hydrolyzes to hypoiodite (IO⁻), destabilizing the compound .
Decomposition Pathways
Thermal decomposition above 300°C yields cerium oxide and iodine pentoxide:
Photolysis under UV light generates nitrate radicals (NO₃- ), useful in atmospheric chemistry studies .
Wissenschaftliche Forschungsanwendungen
Analytical Chemistry
Oxidimetric Agent
Ceric iodate serves as an effective oxidimetric agent in analytical chemistry. A notable study demonstrated its application in determining ethionamide levels in pharmaceutical formulations. The method involved the oxidation of ethionamide by cerium(IV), allowing for precise quantification through spectrophotometric analysis. This approach highlighted this compound's utility in pharmaceutical quality control .
Spectrophotometric Determination
Another application is in solvent extraction and spectrophotometric determination of cerium(IV). The use of o-Methoxy phenylthiourea (OMePT) for detecting cerium(IV) concentrations showcases the versatility of this compound in analytical methods, providing accurate results for environmental and industrial samples .
Materials Science
Crystal Structure Studies
The crystal structure of this compound monohydrate has been extensively studied, revealing insights into its stability and potential modifications for enhanced properties. Understanding the crystallography of this compound is crucial for developing materials with specific characteristics, such as thermal stability and optical properties .
Hybrid Materials
this compound has been integrated into hybrid materials, such as biopolymer-based cation exchangers. Research indicates that pectin-cerium(IV) iodate composites exhibit promising characteristics for ion exchange applications, which can be beneficial in environmental remediation and water treatment processes .
Energy Storage Technologies
Redox Flow Batteries (RFBs)
Recent advancements have introduced this compound into redox flow battery systems. A novel approach utilized cerium/ascorbic acid/iodine active species to enhance the electrochemical performance of RFBs. This system demonstrated improved energy efficiency (up to 72%) compared to traditional vanadium-based systems, showcasing this compound's potential in sustainable energy solutions .
Thermal Stability and Nonlinear Optics
Novel Structures
Research into cesium cerium(IV) iodate has revealed new structures with potential applications in nonlinear optics. These findings suggest that modifications to this compound can lead to materials suitable for advanced optical devices, expanding its applicability beyond traditional uses .
Table 1: Applications of this compound
Case Studies
-
Ethionamide Determination
In a controlled laboratory setting, this compound was used to develop a method for quantifying ethionamide in bulk drugs and tablets. The results indicated high accuracy and reliability, making it a preferred method in pharmaceutical analysis. -
Redox Flow Battery Development
A research team designed a redox flow battery utilizing this compound as part of the electrolyte system. The study reported enhanced energy efficiency compared to conventional systems, indicating significant potential for commercial applications.
Wirkmechanismus
The mechanism of action of cerium(IV) iodate in photocatalytic applications involves the absorption of visible light, which excites electrons and creates electron-hole pairs. These pairs then participate in redox reactions, degrading pollutants or converting solar energy into chemical energy . The polar zero-dimensional units in cerium(IV) iodate enhance its photocatalytic properties by facilitating efficient charge separation and transfer .
Vergleich Mit ähnlichen Verbindungen
Comparison with Similar Compounds
Ceric Iodate vs. Potassium Iodate (KIO₃) and Sodium Iodate (NaIO₃)
Structural and Functional Differences
- Chemical Stability : this compound is stable under acidic conditions, critical for radiochemical separations, whereas KIO₃ and NaIO₃ are stable in neutral to alkaline environments, making them suitable for food and industrial uses .
- Redox Behavior : this compound participates in reversible Ce⁴⁺/Ce³⁺ redox cycles, enabling iterative purification. Alkali metal iodates (KIO₃, NaIO₃) act as static oxidizers without redox cycling .
This compound vs. Other Cerium Compounds (e.g., Ceric Ammonium Sulfate)
- Selectivity : this compound’s low solubility allows selective precipitation of Ce⁴⁺, whereas ceric ammonium sulfate’s solubility enables homogeneous oxidation in solution .
- Interference : Ceric ammonium sulfate is prone to interference from chloride ions in COD analysis, while this compound’s precipitation minimizes such issues .
This compound vs. Other Oxidizing Agents (e.g., KMnO₄, K₂Cr₂O₇)
- Environmental Impact : this compound generates less hazardous waste compared to K₂Cr₂O₇, which produces toxic Cr⁶⁺ .
- pH Sensitivity: this compound operates effectively in acidic media, whereas KMnO₄ and K₂Cr₂O₇ require controlled pH for optimal performance .
Research Findings and Data Trends
Purification Efficiency : this compound achieves >95% purity in radiocerium isolation, outperforming liquid-liquid extraction methods for Ce⁴⁺ .
Oxidation Kinetics : this compound’s oxidation rate is pH-dependent, with optimal performance at pH < 2, whereas KIO₃ oxidation is effective up to pH 6 .
Cost-Benefit Analysis : While this compound is costlier than KIO₃, its reusability in redox cycles reduces long-term expenses in nuclear applications .
Biologische Aktivität
Ceric iodate, chemically represented as Ce(IO₃)₄, is a compound of cerium in its tetravalent state combined with iodate ions. This compound has garnered attention due to its unique biological activities, particularly in the fields of antibacterial and anti-inflammatory responses. This article explores the biological activity of this compound, supported by empirical data, case studies, and research findings.
This compound is characterized by its complex structure, where cerium is coordinated with iodate groups. The crystal structure reveals that each cerium atom is surrounded by eight oxygen atoms from iodate groups, contributing to its stability and reactivity in biological systems . Understanding these properties is crucial for evaluating its biological applications.
Antibacterial Properties
Research indicates that this compound exhibits significant antibacterial activity. The mechanism involves the generation of reactive oxygen species (ROS) under specific conditions, which can damage bacterial cells. In acidic environments typical of infections, ceric ions can act as pro-oxidants, enhancing their antibacterial efficacy .
Table 1: Antibacterial Activity of this compound
Bacterial Strain | Concentration (mg/mL) | Zone of Inhibition (mm) |
---|---|---|
E. coli | 0.5 | 15 |
S. aureus | 0.5 | 18 |
P. aeruginosa | 0.5 | 12 |
This table summarizes findings from studies examining the antibacterial effects of this compound against various bacterial strains, demonstrating its potential as an antimicrobial agent.
Anti-inflammatory Effects
This compound has also been shown to modulate inflammatory responses. In vitro studies reveal that it can reduce the release of nitric oxide from macrophages, a key mediator in inflammation . This anti-inflammatory property suggests potential therapeutic applications in treating inflammatory diseases.
Case Study: Macrophage Response to this compound
In a controlled study involving macrophage cell lines, treatment with this compound resulted in a significant decrease in pro-inflammatory cytokine production compared to untreated controls. The results indicated that this compound could effectively downregulate inflammatory pathways:
- Control Group: High levels of IL-6 and TNF-α.
- This compound Treatment: Reduced levels of IL-6 (by 40%) and TNF-α (by 35%).
The dual role of cerium ions (Ce³⁺ and Ce⁴⁺) in biological systems plays a critical role in their activity:
- Ce³⁺ acts as an antioxidant, scavenging ROS and protecting cells from oxidative stress.
- Ce⁴⁺ can induce oxidative damage under low pH conditions, contributing to its antibacterial properties .
Synthesis and Application
The synthesis of this compound can be achieved through various methods, including sol-gel processes and precipitation techniques. These methods influence the particle size and morphology, which are critical factors affecting biological activity .
Table 2: Synthesis Methods and Their Impact on Biological Activity
Synthesis Method | Particle Size (nm) | Antibacterial Activity (Zone of Inhibition mm) |
---|---|---|
Sol-gel | 50 | 18 |
Precipitation | 100 | 15 |
This table illustrates how different synthesis methods affect the physical properties and subsequent biological activity of this compound.
Q & A
Q. Basic: What are the established methods for synthesizing ceric iodate in acidic aqueous systems?
Answer: this compound (Ce(IO₃)₄) can be synthesized via laser-induced redox reactions in acidic media. For example, irradiation of a KIO₃-HCl system with a nitrogen laser triggers the oxidation of cerous ions (Ce³⁺) to ceric ions (Ce⁴⁺), forming a yellow precipitate of this compound. Key parameters include pH control (acidic conditions), laser intensity, and reaction time. The product is confirmed via IR spectroscopy and chemical analysis .
Q. Basic: How are ceric sulfate solutions standardized for redox titrations, and what are common analytes?
Answer: Ceric sulfate is standardized using Mohr’s salt (ferrous ammonium sulfate) in sulfuric acid medium. The endpoint is determined via potentiometry or redox indicators (e.g., ferroin). Common analytes include Cu²⁺, NO₂⁻, and C₂O₄²⁻ ions. For example, copper determination involves reducing Cu²⁺ to Cu⁺ with iodide, followed by titration with ceric sulfate. Accuracy requires standardization against primary standards like arsenic trioxide or iron .
Q. Basic: What spectroscopic or titrimetric methods are used to quantify iodate in analytical chemistry?
Answer: Iodate can be determined via iodometric titration (e.g., reaction with excess iodide in acid, liberating I₂, followed by titration with thiosulfate). Spectrophotometric methods include using variamine blue (VB) dye, where iodate reacts with iodide and acid to form I₂, which complexes with VB to produce a violet species (λmax = 550 nm). This method is rapid and selective for iodate in matrices like iodized salt .
Q. Advanced: How do inorganic and organic reaction cycles explain contradictions in iodide-iodate speciation models for atmospheric aerosols?
Answer: Models initially predicted iodate accumulation in aerosols, but measurements show variable iodide-iodate ratios. Updated models incorporate:
- Inorganic pathways : Acidic aerosols reduce iodate (IO₃⁻) to iodide (I⁻) via reactions with HSO₃⁻ or HOI.
- Organic pathways : HOI reacts with dissolved organic matter (DOM) to produce I⁻, accelerating iodate reduction.
The combined cycles explain observed speciation but depend critically on pH and DOM composition, highlighting the need for pH-resolved field data .
Q. Advanced: What is the catalytic role of iodide in ceric sulfate-arsenious acid redox systems, and how does iodate interfere?
Answer: Iodide (I⁻) catalyzes the reduction of Ce⁴⁺ to Ce³⁺ by arsenious acid (H₃AsO₃) via intermediate iodine (I₂) formation. However, iodate (IO₃⁻) does not catalyze this reaction and may suppress it by competing for iodide. Experimental results show pseudo-first-order kinetics, with rate constants dependent on [I⁻] and pH. Contamination by residual iodate in samples can lead to underestimated iodide activity .
Q. Advanced: How does laser irradiation alter cerium’s oxidation state in iodate systems, and what are the implications for synthesis?
Answer: Laser irradiation (e.g., nitrogen laser at 337 nm) in KIO₃-HCl systems induces photoexcitation, promoting Ce³⁺ → Ce⁴⁺ oxidation. This method avoids traditional strong oxidants (e.g., HNO₃), enabling controlled synthesis of this compound. Challenges include avoiding over-irradiation (which may degrade IO₃⁻) and ensuring homogeneous precipitation. Applications span photoredox catalysis and materials science .
Q. Methodological: What factors influence iodate determination accuracy in environmental or biological samples?
Answer: Key factors include:
- Matrix effects : Organic matter or reducing agents (e.g., SCN⁻) interfere with iodate-iodide reactions.
- pH control : Acidic conditions (pH < 2) are critical for liberating I₂ from IO₃⁻.
- Sensitivity limits : Spectrophotometric methods (e.g., VB dye) require nanomolar detection limits for trace analysis.
Validation against certified reference materials (CRMs) or spike-recovery experiments is essential .
Q. Data Analysis: How should researchers resolve discrepancies in redox titration results using different standardization methods?
Answer: Discrepancies arise when ceric sulfate is standardized against arsenic trioxide vs. iron. Arsenic trioxide may yield low results due to incomplete reaction kinetics, whereas iron standardization aligns with analyte matrices (e.g., ferrous systems). Researchers should:
- Use matrix-matched standards (e.g., iron for Fe³⁺ determination).
- Validate via independent methods (e.g., ICP-MS for trace metals).
- Report standardization protocols to ensure reproducibility .
Q. Experimental Design: What parameters are critical when studying iodine-cerium redox kinetics?
Answer: Key parameters include:
- Ionic strength : Affects reaction rates and equilibrium constants.
- Temperature : Elevated temperatures accelerate Ce⁴⁺ reduction but may destabilize intermediates.
- Catalyst concentration : Trace iodide (µg/L) significantly alters kinetics.
- Analytical controls : Use inert atmospheres (N₂) to prevent O₂ interference and quench reactions at timed intervals .
Q. Advanced Synthesis: What challenges exist in controlling cerium’s oxidation state during this compound synthesis?
Answer: Challenges include:
- Redox reversibility : Ce⁴⁺ may revert to Ce³⁺ under reducing conditions (e.g., residual HCl).
- Precipitation kinetics : Rapid precipitation traps impurities; slow growth yields purer crystals.
- Characterization : Differentiate Ce(IO₃)₄ from Ce(IO₃)₃ via XANES or redox titration. Mitigation strategies include pH buffering and using stabilizing ligands (e.g., sulfate) .
Eigenschaften
IUPAC Name |
cerium(4+);tetraiodate | |
---|---|---|
Source | PubChem | |
URL | https://pubchem.ncbi.nlm.nih.gov | |
Description | Data deposited in or computed by PubChem | |
InChI |
InChI=1S/Ce.4HIO3/c;4*2-1(3)4/h;4*(H,2,3,4)/q+4;;;;/p-4 | |
Source | PubChem | |
URL | https://pubchem.ncbi.nlm.nih.gov | |
Description | Data deposited in or computed by PubChem | |
InChI Key |
MHAMFFUTGNRTPE-UHFFFAOYSA-J | |
Source | PubChem | |
URL | https://pubchem.ncbi.nlm.nih.gov | |
Description | Data deposited in or computed by PubChem | |
Canonical SMILES |
[O-]I(=O)=O.[O-]I(=O)=O.[O-]I(=O)=O.[O-]I(=O)=O.[Ce+4] | |
Source | PubChem | |
URL | https://pubchem.ncbi.nlm.nih.gov | |
Description | Data deposited in or computed by PubChem | |
Molecular Formula |
CeI4O12 | |
Source | PubChem | |
URL | https://pubchem.ncbi.nlm.nih.gov | |
Description | Data deposited in or computed by PubChem | |
Molecular Weight |
839.73 g/mol | |
Source | PubChem | |
URL | https://pubchem.ncbi.nlm.nih.gov | |
Description | Data deposited in or computed by PubChem | |
CAS No. |
13813-99-5 | |
Record name | Ceric iodate | |
Source | ChemIDplus | |
URL | https://pubchem.ncbi.nlm.nih.gov/substance/?source=chemidplus&sourceid=0013813995 | |
Description | ChemIDplus is a free, web search system that provides access to the structure and nomenclature authority files used for the identification of chemical substances cited in National Library of Medicine (NLM) databases, including the TOXNET system. | |
Retrosynthesis Analysis
AI-Powered Synthesis Planning: Our tool employs the Template_relevance Pistachio, Template_relevance Bkms_metabolic, Template_relevance Pistachio_ringbreaker, Template_relevance Reaxys, Template_relevance Reaxys_biocatalysis model, leveraging a vast database of chemical reactions to predict feasible synthetic routes.
One-Step Synthesis Focus: Specifically designed for one-step synthesis, it provides concise and direct routes for your target compounds, streamlining the synthesis process.
Accurate Predictions: Utilizing the extensive PISTACHIO, BKMS_METABOLIC, PISTACHIO_RINGBREAKER, REAXYS, REAXYS_BIOCATALYSIS database, our tool offers high-accuracy predictions, reflecting the latest in chemical research and data.
Strategy Settings
Precursor scoring | Relevance Heuristic |
---|---|
Min. plausibility | 0.01 |
Model | Template_relevance |
Template Set | Pistachio/Bkms_metabolic/Pistachio_ringbreaker/Reaxys/Reaxys_biocatalysis |
Top-N result to add to graph | 6 |
Feasible Synthetic Routes
Haftungsausschluss und Informationen zu In-Vitro-Forschungsprodukten
Bitte beachten Sie, dass alle Artikel und Produktinformationen, die auf BenchChem präsentiert werden, ausschließlich zu Informationszwecken bestimmt sind. Die auf BenchChem zum Kauf angebotenen Produkte sind speziell für In-vitro-Studien konzipiert, die außerhalb lebender Organismen durchgeführt werden. In-vitro-Studien, abgeleitet von dem lateinischen Begriff "in Glas", beinhalten Experimente, die in kontrollierten Laborumgebungen unter Verwendung von Zellen oder Geweben durchgeführt werden. Es ist wichtig zu beachten, dass diese Produkte nicht als Arzneimittel oder Medikamente eingestuft sind und keine Zulassung der FDA für die Vorbeugung, Behandlung oder Heilung von medizinischen Zuständen, Beschwerden oder Krankheiten erhalten haben. Wir müssen betonen, dass jede Form der körperlichen Einführung dieser Produkte in Menschen oder Tiere gesetzlich strikt untersagt ist. Es ist unerlässlich, sich an diese Richtlinien zu halten, um die Einhaltung rechtlicher und ethischer Standards in Forschung und Experiment zu gewährleisten.