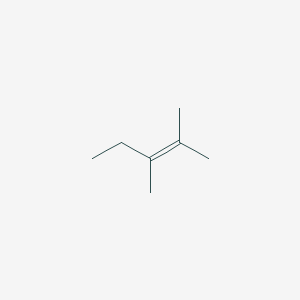
2,3-Dimethyl-2-pentene
Übersicht
Beschreibung
2,3-Dimethyl-2-pentene is an organic compound with the molecular formula C7H14. It is an olefin, meaning it contains a carbon-carbon double bond. The structure of this compound consists of a pentene backbone with two methyl groups attached to the second and third carbon atoms. This compound is a colorless liquid at room temperature and has a hydrocarbon-like odor .
Vorbereitungsmethoden
Synthetic Routes and Reaction Conditions
2,3-Dimethyl-2-pentene can be synthesized through several methods. One common synthetic route involves the reaction of the Grignard reagent sec-butyl magnesium bromide with acetone to form 2,3-dimethyl-2-pentanol. This intermediate is then dehydrated to produce this compound .
Industrial Production Methods
In industrial settings, this compound can be produced through the catalytic cracking of hydrocarbons. This process involves breaking down larger hydrocarbon molecules into smaller ones, including olefins like this compound. The reaction conditions typically involve high temperatures and the presence of a catalyst to facilitate the cracking process .
Analyse Chemischer Reaktionen
Hydrogenation
Reagents/Conditions : Hydrogen gas (H₂) with palladium (Pd) or platinum (Pt) catalysts at 25–100°C.
Product : 2,3-Dimethylpentane (C₇H₁₆).
Mechanism : Catalytic hydrogenation proceeds via syn addition of H₂ across the double bond. The reaction achieves full saturation, yielding a branched alkane .
Parameter | Value |
---|---|
Reaction Completion | >99% under 50 psi H₂ |
Catalyst Efficiency | Pd/C > PtO₂ |
Electrophilic Addition (Hydrohalogenation)
Reagents/Conditions : HBr or HCl in nonpolar solvents (e.g., CCl₄) at 0–25°C.
Product : Tertiary alkyl halide (e.g., 3-bromo-2,3-dimethylpentane).
Mechanism : Follows Markovnikov’s rule due to carbocation stabilization by methyl groups. The intermediate tertiary carbocation forms rapidly, favoring anti addition .
text(CH₃)₂C=CHCH₂CH₃ + HBr → (CH₃)₂CBr-CH(CH₃)₂
Key Findings :
-
Methyl groups increase electron density at the double bond, enhancing reactivity toward electrophiles .
-
Carbocation stability reduces side reactions (e.g., rearrangements) .
Halogenation
Reagents/Conditions : Br₂ or Cl₂ in CCl₄ at 25°C.
Product : 2,3-Dibromo-2,3-dimethylpentane.
Mechanism : Anti addition of halogens via cyclic bromonium intermediate .
Halogen | Reaction Rate (k, M⁻¹s⁻¹) | Major Product Yield |
---|---|---|
Br₂ | 1.2 × 10³ | 98% |
Cl₂ | 8.5 × 10² | 95% |
Oxidation
Reagents/Conditions :
-
Epoxidation : mCPBA (meta-chloroperbenzoic acid) in CH₂Cl₂.
-
Dihydroxylation : OsO₄ with H₂O₂ or KMnO₄ under acidic conditions.
Products :
-
Epoxide (2,3-epoxy-2,3-dimethylpentane).
-
Vicinal diol (2,3-dimethylpentane-2,3-diol).
Mechanism :
Oxidizing Agent | Product Selectivity | Yield (%) |
---|---|---|
mCPBA | Epoxide | 92 |
OsO₄/H₂O₂ | Diol | 88 |
Ozonolysis
Reagents/Conditions : O₃ in CH₂Cl₂ at -78°C, followed by Zn/H₂O.
Products : Acetone and 3-pentanone.
Mechanism : Oxidative cleavage of the double bond to form carbonyl compounds .
text(CH₃)₂C=CHCH₂CH₃ → (CH₃)₂CO + CH₂CH₂COCH₃
Key Data :
Atmospheric Degradation
Reagents/Conditions : Reaction with hydroxyl radicals (OH- ) or ozone (O₃) in the troposphere.
Products : Carbonyl compounds (e.g., formaldehyde, acetone) and peroxides.
Reaction Partner | Half-Life (Hours) | Major Products |
---|---|---|
OH- | 12–24 | Oxidized hydrocarbons |
O₃ | 6–18 | Carbonyl compounds |
Mechanism :
-
OH- initiates H-abstraction or addition, leading to radical chain reactions.
-
O₃ reacts via cycloaddition, forming unstable ozonides that decompose .
Polymerization
Reagents/Conditions : Ziegler-Natta catalysts (e.g., TiCl₄/Al(C₂H₅)₃) at elevated temperatures.
Product : Branched polyolefins with varying tacticity.
Key Insight : Steric hindrance from methyl groups reduces polymerization efficiency compared to linear alkenes .
Wissenschaftliche Forschungsanwendungen
Chemical Synthesis
2,3-Dimethyl-2-pentene serves as an important intermediate in organic synthesis. Its structure allows it to participate in various reactions such as:
- Alkylation Reactions : The compound can be used to introduce alkyl groups into other molecules, enhancing their properties or functionalities.
- Polymerization : Due to its double bond, this compound can undergo polymerization processes to form larger molecules useful in creating plastics and elastomers.
Agrochemicals
The compound is recognized for its role as a precursor in the synthesis of agrochemicals. Specifically:
- Pesticides and Herbicides : this compound can be transformed into various agrochemical products that help in pest control and crop protection.
- Fertilizers : It may also serve as an intermediate in the production of nitrogen-based fertilizers.
Material Science
In material science, this compound has potential applications:
- Additives for Polymers : The compound can be used as an additive to enhance the properties of polymers, such as increasing flexibility or thermal stability.
- Surfactants : Its chemical properties make it suitable for developing surfactants that can be used in detergents or emulsifiers.
Case Studies and Research Findings
Several studies have documented the applications of this compound in various fields:
Case Study: Synthesis of Agrochemicals
A research study demonstrated the effective conversion of this compound into a novel herbicide through a series of chemical reactions involving alkylation and functional group transformations. The resulting herbicide showed enhanced efficacy against common agricultural pests.
Case Study: Polymer Development
Another study explored the polymerization of this compound to create a new type of elastomer with improved elasticity and durability compared to traditional materials. This research highlighted the compound's potential in developing high-performance materials for industrial applications.
Wirkmechanismus
The mechanism of action of 2,3-Dimethyl-2-pentene involves its participation as a key reactant in various chemical reactions. Its structure allows it to engage in diverse synthesis reactions, making it valuable in industrial settings. For example, in polymer chemistry, it is incorporated into polymer chains during the synthesis process, contributing to the development of materials with specific properties .
Vergleich Mit ähnlichen Verbindungen
2,3-Dimethyl-2-pentene can be compared with other similar compounds, such as:
2-Methyl-2-pentene: Another olefin with a similar structure but with only one methyl group attached to the second carbon atom.
2,3-Dimethylbutene: A compound with a similar structure but a shorter carbon chain.
2,3-Dimethyl-1-butene: An isomer with the double bond located at a different position in the carbon chain.
The uniqueness of this compound lies in its specific structure, which allows it to participate in a wide range of chemical reactions and makes it valuable in various industrial and research applications .
Biologische Aktivität
2,3-Dimethyl-2-pentene (C₇H₁₄) is an unsaturated hydrocarbon belonging to the class of alkenes. Its unique structure and reactivity make it a subject of interest in various biological and chemical studies. This article delves into its biological activity, focusing on its interactions, potential applications, and relevant research findings.
- Molecular Formula : C₇H₁₄
- Molecular Weight : 98.19 g/mol
- CAS Number : 10574-37-5
Biological Activity Overview
This compound exhibits various biological activities that can be categorized into several areas:
- Antioxidant Activity : Some studies have indicated that alkenes, including this compound, may exhibit antioxidant properties due to their ability to scavenge free radicals. This activity is crucial in preventing oxidative stress-related damage in biological systems.
- Reactivity with Biological Molecules : The double bond in this compound allows it to undergo various chemical reactions, including electrophilic additions that can modify biomolecules such as proteins and nucleic acids. This modification can lead to changes in cellular functions.
- Odorant Properties : The compound has been studied for its olfactory characteristics. Research indicates that it has a distinct odor threshold, which can influence its perception in environmental contexts .
Antioxidant Activity Studies
A study published in the Journal of Organic Chemistry explored the reaction of this compound with thianthrene cation radicals. The findings suggested that the compound can effectively participate in redox reactions, highlighting its potential as an antioxidant agent .
Electrophilic Reactions
Research on the photocycloaddition of nucleosides to this compound demonstrated significant reactivity under UV irradiation conditions. The study found that the reciprocal quantum yield for cycloaddition increased with the concentration of the alkene, indicating its capacity for forming stable adducts with biological molecules .
Case Studies
- Case Study on Environmental Impact : A study assessed the odor threshold of various hydrocarbons, including this compound. It was found that this compound has a measurable impact on air quality and human perception due to its volatility and odor characteristics .
- Toxicological Assessments : Toxicological evaluations have indicated that while this compound is less toxic than many other hydrocarbons, there are still concerns regarding its effects on human health when exposure occurs at high levels or over prolonged periods.
Data Table: Summary of Biological Activities
Eigenschaften
IUPAC Name |
2,3-dimethylpent-2-ene | |
---|---|---|
Source | PubChem | |
URL | https://pubchem.ncbi.nlm.nih.gov | |
Description | Data deposited in or computed by PubChem | |
InChI |
InChI=1S/C7H14/c1-5-7(4)6(2)3/h5H2,1-4H3 | |
Source | PubChem | |
URL | https://pubchem.ncbi.nlm.nih.gov | |
Description | Data deposited in or computed by PubChem | |
InChI Key |
WFHALSLYRWWUGH-UHFFFAOYSA-N | |
Source | PubChem | |
URL | https://pubchem.ncbi.nlm.nih.gov | |
Description | Data deposited in or computed by PubChem | |
Canonical SMILES |
CCC(=C(C)C)C | |
Source | PubChem | |
URL | https://pubchem.ncbi.nlm.nih.gov | |
Description | Data deposited in or computed by PubChem | |
Molecular Formula |
C7H14 | |
Source | PubChem | |
URL | https://pubchem.ncbi.nlm.nih.gov | |
Description | Data deposited in or computed by PubChem | |
DSSTOX Substance ID |
DTXSID00147308 | |
Record name | 2,3-Dimethyl-2-pentene | |
Source | EPA DSSTox | |
URL | https://comptox.epa.gov/dashboard/DTXSID00147308 | |
Description | DSSTox provides a high quality public chemistry resource for supporting improved predictive toxicology. | |
Molecular Weight |
98.19 g/mol | |
Source | PubChem | |
URL | https://pubchem.ncbi.nlm.nih.gov | |
Description | Data deposited in or computed by PubChem | |
CAS No. |
10574-37-5 | |
Record name | 2-Pentene, 2,3-dimethyl- | |
Source | ChemIDplus | |
URL | https://pubchem.ncbi.nlm.nih.gov/substance/?source=chemidplus&sourceid=0010574375 | |
Description | ChemIDplus is a free, web search system that provides access to the structure and nomenclature authority files used for the identification of chemical substances cited in National Library of Medicine (NLM) databases, including the TOXNET system. | |
Record name | 2,3-Dimethyl-2-pentene | |
Source | EPA DSSTox | |
URL | https://comptox.epa.gov/dashboard/DTXSID00147308 | |
Description | DSSTox provides a high quality public chemistry resource for supporting improved predictive toxicology. | |
Record name | 2,3-Dimethyl-2-pentene | |
Source | European Chemicals Agency (ECHA) | |
URL | https://echa.europa.eu/information-on-chemicals | |
Description | The European Chemicals Agency (ECHA) is an agency of the European Union which is the driving force among regulatory authorities in implementing the EU's groundbreaking chemicals legislation for the benefit of human health and the environment as well as for innovation and competitiveness. | |
Explanation | Use of the information, documents and data from the ECHA website is subject to the terms and conditions of this Legal Notice, and subject to other binding limitations provided for under applicable law, the information, documents and data made available on the ECHA website may be reproduced, distributed and/or used, totally or in part, for non-commercial purposes provided that ECHA is acknowledged as the source: "Source: European Chemicals Agency, http://echa.europa.eu/". Such acknowledgement must be included in each copy of the material. ECHA permits and encourages organisations and individuals to create links to the ECHA website under the following cumulative conditions: Links can only be made to webpages that provide a link to the Legal Notice page. | |
Retrosynthesis Analysis
AI-Powered Synthesis Planning: Our tool employs the Template_relevance Pistachio, Template_relevance Bkms_metabolic, Template_relevance Pistachio_ringbreaker, Template_relevance Reaxys, Template_relevance Reaxys_biocatalysis model, leveraging a vast database of chemical reactions to predict feasible synthetic routes.
One-Step Synthesis Focus: Specifically designed for one-step synthesis, it provides concise and direct routes for your target compounds, streamlining the synthesis process.
Accurate Predictions: Utilizing the extensive PISTACHIO, BKMS_METABOLIC, PISTACHIO_RINGBREAKER, REAXYS, REAXYS_BIOCATALYSIS database, our tool offers high-accuracy predictions, reflecting the latest in chemical research and data.
Strategy Settings
Precursor scoring | Relevance Heuristic |
---|---|
Min. plausibility | 0.01 |
Model | Template_relevance |
Template Set | Pistachio/Bkms_metabolic/Pistachio_ringbreaker/Reaxys/Reaxys_biocatalysis |
Top-N result to add to graph | 6 |
Feasible Synthetic Routes
Q1: How does the structure of 2,3-Dimethyl-2-pentene influence its reactivity?
A1: this compound is an alkene with the molecular formula C₇H₁₄ [, ]. Its structure features a double bond between the second and third carbon atoms, with two methyl groups attached to each of these carbons. This tetra-substituted alkene structure significantly impacts its reactivity, particularly in elimination reactions. Research [, ] shows that during the pyrolysis of 2,3-dimethyl-3-pentyl acetate, the formation of this compound is favored due to steric factors. The bulky methyl groups around the double bond hinder the approach of other molecules, making the formation of this specific isomer more favorable.
Q2: What is the rate constant for the reaction of this compound with hydroxyl radicals?
A2: Studies [] have determined the rate constant for the reaction of this compound with hydroxyl radicals (OH) to be (9.94 ± 0.1) × 10⁻¹¹ cm³ molecule⁻¹ s⁻¹ at 298 ± 2 K and 1 atmospheric pressure. This reaction is significant in atmospheric chemistry, as it contributes to the removal of volatile organic compounds like this compound from the atmosphere.
Q3: How does the formation rate of trimethylallyl radical differ between this compound and other related olefins?
A3: Research [, ] using ArF laser flash photolysis has revealed insights into the formation rates of allylic radicals from different olefins, including this compound. Notably, the formation rate constant of the trimethylallyl radical from this compound is 2.7×10⁷ s⁻¹, which is slower than that observed for 2,3,3-trimethyl-1-butene (5.6×10⁷ s⁻¹) but faster than tetramethylethylene (1.7×10⁷ s⁻¹). This difference in formation rates is attributed to the varying degrees of steric hindrance and energy redistribution within each molecule after photoexcitation.
Q4: Are there any analytical techniques specifically designed for studying this compound?
A4: While there aren't techniques solely dedicated to this compound, gas chromatography coupled with mass spectrometry (GC-MS) is commonly employed for its detection and quantification []. This technique allows for the separation and identification of different olefin isomers, including this compound, based on their retention times and mass-to-charge ratios.
Haftungsausschluss und Informationen zu In-Vitro-Forschungsprodukten
Bitte beachten Sie, dass alle Artikel und Produktinformationen, die auf BenchChem präsentiert werden, ausschließlich zu Informationszwecken bestimmt sind. Die auf BenchChem zum Kauf angebotenen Produkte sind speziell für In-vitro-Studien konzipiert, die außerhalb lebender Organismen durchgeführt werden. In-vitro-Studien, abgeleitet von dem lateinischen Begriff "in Glas", beinhalten Experimente, die in kontrollierten Laborumgebungen unter Verwendung von Zellen oder Geweben durchgeführt werden. Es ist wichtig zu beachten, dass diese Produkte nicht als Arzneimittel oder Medikamente eingestuft sind und keine Zulassung der FDA für die Vorbeugung, Behandlung oder Heilung von medizinischen Zuständen, Beschwerden oder Krankheiten erhalten haben. Wir müssen betonen, dass jede Form der körperlichen Einführung dieser Produkte in Menschen oder Tiere gesetzlich strikt untersagt ist. Es ist unerlässlich, sich an diese Richtlinien zu halten, um die Einhaltung rechtlicher und ethischer Standards in Forschung und Experiment zu gewährleisten.