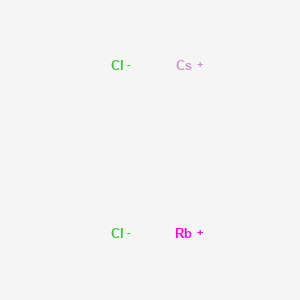
Cesium rubidium chloride
- Klicken Sie auf QUICK INQUIRY, um ein Angebot von unserem Expertenteam zu erhalten.
- Mit qualitativ hochwertigen Produkten zu einem WETTBEWERBSFÄHIGEN Preis können Sie sich mehr auf Ihre Forschung konzentrieren.
Übersicht
Beschreibung
Cesium rubidium chloride is a compound that contains cesium, rubidium, and chloride . Cesium is a very soft, ductile, silvery white metal and is the most reactive of all metals . Rubidium chloride is an alkali metal halide salt and an excellent water-soluble crystalline rubidium source .
Synthesis Analysis
Cesium-incorporated rubidium tin chloride-defect perovskite nanomaterials have been synthesized for photocatalytic applications . These materials are useful for the degradation of dyes under UV irradiation due to their superior properties such as non-toxicity, low cost, simple preparation methods, and long-term thermal stability .Molecular Structure Analysis
The introduction of cesium in the form of cesium chloride during the major steps of protein treatment in crystallography has been investigated . Rubidium and cesium chlorites have been prepared and studied by single crystal and powder X-ray diffraction .Chemical Reactions Analysis
Cesium and rubidium have been researched for their photocatalytic applications . They have been used in the photocatalytic degradation of methylene blue dye under UV light radiation .Physical And Chemical Properties Analysis
Cesium is the most reactive of all metals and has the lowest hardness among all metals . The molecular weight of cesium rubidium chloride is 289.28 g/mol .Wissenschaftliche Forschungsanwendungen
Separation and Extraction from Salt Lake Brine : Cesium and rubidium play important roles in high-tech fields like aerospace engineering, arms development, and perovskite solar cell fabrication. Efficiently separating and extracting these elements from salt lake brine is crucial for their industrial application (Gao et al., 2020).
Diffusivity Measurement : The diffusivity of cesium in aqueous solutions of rubidium chloride is crucial to understand the transport mechanism of Cs ion in the RbCl–H2O system (Chakrabarti & Kanjilal, 2010).
Trace Analysis in High Purity Cesium Chloride : The detection of trace amounts of rubidium in high purity cesium chloride using electrothermal atomic absorption spectrometry is significant for quality control in industrial applications (Dash et al., 2007).
Application in Sodium-ion Batteries : Adding rubidium and cesium ions as electrolyte additives in sodium-ion batteries can significantly improve the ionic conductivity and stability of solid electrolyte interphase on hard carbon surfaces (Che et al., 2017).
Activity Coefficients in Methanol–Water Mixtures : Research on activity coefficients of rubidium chloride and cesium chloride in methanol–water mixtures helps in understanding the behavior of these salts in various solvent systems (Cui et al., 2007).
Agricultural Research : Rubidium chloride and cesium chloride have been used for marking insects like Diatraea grandiosella in ecological studies, demonstrating their utility in agricultural research (Qureshi et al., 2004).
Oceanographic Studies : Determining the concentration of rubidium, cesium, and barium in oceanic profiles helps in understanding the distribution of these elements in marine environments (Bolter et al., 1964).
Pharmacological Research : The effect of cesium, lithium, and rubidium chlorides on the analgesic action of morphine has been investigated, highlighting their potential pharmacological applications (Bulaev & Ostrovskaya, 1978).
Wirkmechanismus
Safety and Hazards
Cesium chloride can cause irregular heartbeats, also called arrhythmias . There have been reports of humans experiencing serious heart problems after taking CsCl . Rubidium and cesium are only slightly toxic on an acute toxicologic basis and would pose an acute health hazard only when ingested in large quantities .
Zukünftige Richtungen
Rubidium and cesium play increasingly important roles in high-tech fields such as aerospace engineering, arms development, and perovskite solar cell fabrication owing to their unique physical and chemical properties . Efficiently separating and extracting these resources is crucial for their industrial application .
Eigenschaften
IUPAC Name |
cesium;rubidium(1+);dichloride |
Source
|
---|---|---|
Source | PubChem | |
URL | https://pubchem.ncbi.nlm.nih.gov | |
Description | Data deposited in or computed by PubChem | |
InChI |
InChI=1S/2ClH.Cs.Rb/h2*1H;;/q;;2*+1/p-2 |
Source
|
Source | PubChem | |
URL | https://pubchem.ncbi.nlm.nih.gov | |
Description | Data deposited in or computed by PubChem | |
InChI Key |
ZGANVVAGMVHDBD-UHFFFAOYSA-L |
Source
|
Source | PubChem | |
URL | https://pubchem.ncbi.nlm.nih.gov | |
Description | Data deposited in or computed by PubChem | |
Canonical SMILES |
[Cl-].[Cl-].[Rb+].[Cs+] |
Source
|
Source | PubChem | |
URL | https://pubchem.ncbi.nlm.nih.gov | |
Description | Data deposited in or computed by PubChem | |
Molecular Formula |
Cl2CsRb |
Source
|
Source | PubChem | |
URL | https://pubchem.ncbi.nlm.nih.gov | |
Description | Data deposited in or computed by PubChem | |
DSSTOX Substance ID |
DTXSID00914779 |
Source
|
Record name | Caesium rubidium chloride (1/1/2) | |
Source | EPA DSSTox | |
URL | https://comptox.epa.gov/dashboard/DTXSID00914779 | |
Description | DSSTox provides a high quality public chemistry resource for supporting improved predictive toxicology. | |
Molecular Weight |
289.28 g/mol |
Source
|
Source | PubChem | |
URL | https://pubchem.ncbi.nlm.nih.gov | |
Description | Data deposited in or computed by PubChem | |
Product Name |
Cesium rubidium chloride | |
CAS RN |
95860-63-2 |
Source
|
Record name | Caesium rubidium chloride (1/1/2) | |
Source | EPA DSSTox | |
URL | https://comptox.epa.gov/dashboard/DTXSID00914779 | |
Description | DSSTox provides a high quality public chemistry resource for supporting improved predictive toxicology. | |
Haftungsausschluss und Informationen zu In-Vitro-Forschungsprodukten
Bitte beachten Sie, dass alle Artikel und Produktinformationen, die auf BenchChem präsentiert werden, ausschließlich zu Informationszwecken bestimmt sind. Die auf BenchChem zum Kauf angebotenen Produkte sind speziell für In-vitro-Studien konzipiert, die außerhalb lebender Organismen durchgeführt werden. In-vitro-Studien, abgeleitet von dem lateinischen Begriff "in Glas", beinhalten Experimente, die in kontrollierten Laborumgebungen unter Verwendung von Zellen oder Geweben durchgeführt werden. Es ist wichtig zu beachten, dass diese Produkte nicht als Arzneimittel oder Medikamente eingestuft sind und keine Zulassung der FDA für die Vorbeugung, Behandlung oder Heilung von medizinischen Zuständen, Beschwerden oder Krankheiten erhalten haben. Wir müssen betonen, dass jede Form der körperlichen Einführung dieser Produkte in Menschen oder Tiere gesetzlich strikt untersagt ist. Es ist unerlässlich, sich an diese Richtlinien zu halten, um die Einhaltung rechtlicher und ethischer Standards in Forschung und Experiment zu gewährleisten.