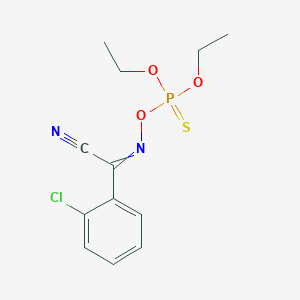
CHLORPHOXIM
Übersicht
Beschreibung
Chlorphoxim is a nitrile.
Wissenschaftliche Forschungsanwendungen
Neurotoxizitätsstudien
Chlorphoxim wurde in Neurotoxizitätsstudien verwendet, insbesondere mit Zebrafischembryonen . Es wurde festgestellt, dass die Verbindung Neurotoxizität in Zebrafischembryonen durch Aktivierung von oxidativem Stress induziert . Es wurde beobachtet, dass die Exposition gegenüber hohen Chlorphoximkonzentrationen zu Schäden am Nervensystem, Veränderungen des Schwimmverhaltens und einer Hemmung der Acetylcholinesterase (AChE) führte .
Forschung zum oxidativen Stress
this compound-Exposition erhöht den oxidativen Stress in Zebrafischembryonen signifikant, indem es antioxidative Enzyme (SOD und CAT) hemmt und reaktive Sauerstoffspezies (ROS) aktiviert . Dies hat zu einem umfassenderen Verständnis der sicheren Verwendung von Organophosphat-Pestiziden beigetragen .
Apoptoseforschung
this compound-Exposition induziert Apoptose, indem es die Expression von Apoptosegenen (Bax, Bcl2 und p53) verstärkt . Dies hat Einblicke in die Mechanismen der Apoptose geliefert, die durch Organophosphat-Pestizide induziert wird .
Pestizideffizienzstudien
this compound ist ein hochwirksames Organophosphat-Pestizid, das seit vielen Jahren in der Verteidigung gegen Kriebelmücken, Moskitos und andere landwirtschaftliche Insekten weit verbreitet ist . Seine Wirksamkeit wurde im Bereich der Schädlingsbekämpfung erforscht .
Umwelttoxikologie
Durch die breite Verwendung von this compound in der landwirtschaftlichen Praxis wird sein Rückstand häufig im Wasser nachgewiesen
Wirkmechanismus
- Chlorphoxim is an insecticide used as a crop protection active ingredient .
- It also acts as a cholinesterase inhibitor and a neurotoxin .
Target of Action
Mode of Action
Biochemical Pathways
Biochemische Analyse
Biochemical Properties
Chlorphoxim plays a significant role in biochemical reactions by interacting with various enzymes and proteins. The primary target of this compound is acetylcholinesterase, which it inhibits by phosphorylating the serine hydroxyl group in the active site of the enzyme. This interaction prevents the breakdown of acetylcholine, leading to an accumulation of this neurotransmitter in synaptic clefts. Additionally, this compound has been shown to interact with other biomolecules such as esterases, which can hydrolyze the compound, and cytochrome P450 enzymes, which are involved in its metabolism .
Cellular Effects
This compound affects various types of cells and cellular processes. In neuronal cells, the inhibition of acetylcholinesterase by this compound leads to excessive stimulation of cholinergic receptors, disrupting normal cell signaling pathways. This can result in altered gene expression and changes in cellular metabolism. In non-neuronal cells, this compound can induce oxidative stress by generating reactive oxygen species, which can damage cellular components such as lipids, proteins, and DNA .
Molecular Mechanism
The molecular mechanism of this compound involves its binding to the active site of acetylcholinesterase, where it forms a covalent bond with the serine residue. This phosphorylation event inhibits the enzyme’s activity, preventing the hydrolysis of acetylcholine. Additionally, this compound can modulate the activity of other enzymes, such as esterases and cytochrome P450s, through competitive inhibition or induction of gene expression .
Temporal Effects in Laboratory Settings
In laboratory settings, the effects of this compound can change over time due to its stability and degradation. This compound is relatively stable under normal conditions, but it can degrade in the presence of light, heat, or alkaline conditions. Long-term exposure to this compound in vitro or in vivo can lead to adaptive responses in cells, such as upregulation of detoxifying enzymes like cytochrome P450s and esterases. These adaptive responses can mitigate the toxic effects of this compound over time .
Dosage Effects in Animal Models
The effects of this compound vary with different dosages in animal models. At low doses, this compound can cause mild symptoms such as tremors and hyperactivity due to its cholinergic effects. At higher doses, it can lead to severe toxicity, including convulsions, respiratory distress, and death. Threshold effects have been observed, where a certain dose is required to elicit a toxic response. Additionally, chronic exposure to sublethal doses of this compound can result in long-term health effects, such as neurobehavioral changes and oxidative stress .
Metabolic Pathways
This compound is metabolized through various pathways, primarily involving cytochrome P450 enzymes. These enzymes can oxidize this compound to form more water-soluble metabolites, which are then excreted from the body. Esterases can also hydrolyze this compound to produce less toxic metabolites. The metabolic flux of this compound can be influenced by factors such as enzyme induction, genetic polymorphisms, and co-exposure to other chemicals .
Transport and Distribution
This compound is transported and distributed within cells and tissues through passive diffusion and active transport mechanisms. It can interact with transporters such as P-glycoprotein, which can affect its localization and accumulation in specific tissues. This compound tends to accumulate in fatty tissues due to its lipophilic nature, and it can cross the blood-brain barrier, leading to central nervous system effects .
Subcellular Localization
The subcellular localization of this compound is influenced by its chemical properties and interactions with cellular components. This compound can localize to the endoplasmic reticulum, where it interacts with cytochrome P450 enzymes involved in its metabolism. It can also be found in the cytoplasm, where it interacts with esterases and other detoxifying enzymes. Post-translational modifications, such as phosphorylation, can affect the targeting and activity of this compound within cells .
Eigenschaften
CAS-Nummer |
14816-20-7 |
---|---|
Molekularformel |
C12H14ClN2O3PS |
Molekulargewicht |
332.74 g/mol |
IUPAC-Name |
(1E)-2-chloro-N-diethoxyphosphinothioyloxybenzenecarboximidoyl cyanide |
InChI |
InChI=1S/C12H14ClN2O3PS/c1-3-16-19(20,17-4-2)18-15-12(9-14)10-7-5-6-8-11(10)13/h5-8H,3-4H2,1-2H3/b15-12- |
InChI-Schlüssel |
GQKRUMZWUHSLJF-QINSGFPZSA-N |
SMILES |
CCOP(=S)(OCC)ON=C(C#N)C1=CC=CC=C1Cl |
Isomerische SMILES |
CCOP(=S)(OCC)O/N=C(/C#N)\C1=CC=CC=C1Cl |
Kanonische SMILES |
CCOP(=S)(OCC)ON=C(C#N)C1=CC=CC=C1Cl |
Key on ui other cas no. |
14816-20-7 |
Piktogramme |
Acute Toxic |
Löslichkeit |
5.11e-06 M |
Synonyme |
Bay 78182 chlorphoxim o-chlorophenylglyoxylonitrile oxime O,O-diethyl phosphorothioate |
Herkunft des Produkts |
United States |
Retrosynthesis Analysis
AI-Powered Synthesis Planning: Our tool employs the Template_relevance Pistachio, Template_relevance Bkms_metabolic, Template_relevance Pistachio_ringbreaker, Template_relevance Reaxys, Template_relevance Reaxys_biocatalysis model, leveraging a vast database of chemical reactions to predict feasible synthetic routes.
One-Step Synthesis Focus: Specifically designed for one-step synthesis, it provides concise and direct routes for your target compounds, streamlining the synthesis process.
Accurate Predictions: Utilizing the extensive PISTACHIO, BKMS_METABOLIC, PISTACHIO_RINGBREAKER, REAXYS, REAXYS_BIOCATALYSIS database, our tool offers high-accuracy predictions, reflecting the latest in chemical research and data.
Strategy Settings
Precursor scoring | Relevance Heuristic |
---|---|
Min. plausibility | 0.01 |
Model | Template_relevance |
Template Set | Pistachio/Bkms_metabolic/Pistachio_ringbreaker/Reaxys/Reaxys_biocatalysis |
Top-N result to add to graph | 6 |
Feasible Synthetic Routes
Q1: How does Chlorphoxim exert its insecticidal effect?
A1: this compound acts by inhibiting acetylcholinesterase (AChE), an enzyme crucial for nerve impulse transmission. [] This inhibition leads to the accumulation of acetylcholine at nerve synapses, causing overstimulation and ultimately paralysis and death in insects. []
Q2: Are there any other biological effects of this compound observed in research?
A2: Beyond AChE inhibition, studies have shown that this compound exposure can induce oxidative stress in zebrafish embryos, leading to neurotoxicity. [] This toxicity is characterized by nervous system damage, altered swimming behavior, and abnormal expression of neural-related genes. []
Q3: What is the molecular formula and weight of this compound?
A3: The molecular formula of this compound is C12H15ClN3O3PS, and its molecular weight is 363.8 g/mol.
Q4: How does the structure of this compound contribute to its insecticidal activity?
A5: The presence of a phosphorus atom with a double bond to either an oxygen or sulfur atom is characteristic of organophosphate insecticides like this compound. [] This structural feature is crucial for binding to and inhibiting AChE. Modifications to the structure, particularly around the phosphorus center, can significantly impact the potency and selectivity of the insecticide. []
Q5: Has resistance to this compound been observed in insect populations?
A6: Yes, resistance to this compound has been documented in Simulium damnosum, the black fly vector of onchocerciasis. This resistance is often seen in conjunction with resistance to temephos, another organophosphate insecticide. [, ]
Q6: What are the potential mechanisms behind this resistance?
A7: Research suggests that increased esterase activity in resistant strains might contribute to both temephos and this compound resistance. [] This increased activity could lead to faster detoxification of the insecticides, rendering them less effective.
Q7: What are the known toxicological effects of this compound?
A8: this compound, like other organophosphates, is a neurotoxin that primarily targets AChE. [, ] Studies on zebrafish embryos highlight its potential to induce oxidative stress and apoptosis, leading to neurotoxicity. []
Q8: What research models have been used to study the efficacy of this compound?
A8: Research on this compound utilizes a variety of models, including:
- Laboratory bioassays: To assess its toxicity on different insect species, such as Simulium larvae [, , ] and Attagenus larvae. []
- Field trials: Open-field trials have been conducted to evaluate this compound's effectiveness in controlling fleas on rodents. [, ]
- Zebrafish embryos: Used to study this compound-induced neurotoxicity and the potential protective effects of antioxidants. []
Q9: How is this compound analyzed in various matrices?
A10: Gas-liquid chromatography (GLC) has been employed for the analysis of this compound residues in water and fish. [, ] This method involves extraction and often derivatization to enhance detection sensitivity.
Q10: What is the environmental fate of this compound?
A11: While specific data on this compound's degradation kinetics may be limited, research suggests organophosphates, in general, undergo hydrolysis and photolysis in the environment. []
Q11: Are there any alternatives to this compound for insect control?
A11: The search for this compound alternatives is ongoing, especially due to emerging resistance. Researchers are exploring:
- Other organophosphates: Compounds like temephos, pirimiphos-methyl, and chlorpyrifos have been investigated. [, , , ]
- Pyrethroids: These insecticides, such as permethrin and deltamethrin, offer a different mode of action. [, , , ]
- Carbamates: Propoxur is one carbamate insecticide considered as a potential alternative. [, , ]
- Biological control agents: Bacillus thuringiensis H-14 is a bacterial larvicide explored for Simulium control. [, ]
Q12: What factors drive the search for this compound alternatives?
A13: * Resistance development: The emergence of this compound resistance in insect populations necessitates the identification of effective alternatives. []* Environmental concerns: The potential for non-target effects on aquatic organisms drives the search for insecticides with reduced environmental impact. [, ]
Haftungsausschluss und Informationen zu In-Vitro-Forschungsprodukten
Bitte beachten Sie, dass alle Artikel und Produktinformationen, die auf BenchChem präsentiert werden, ausschließlich zu Informationszwecken bestimmt sind. Die auf BenchChem zum Kauf angebotenen Produkte sind speziell für In-vitro-Studien konzipiert, die außerhalb lebender Organismen durchgeführt werden. In-vitro-Studien, abgeleitet von dem lateinischen Begriff "in Glas", beinhalten Experimente, die in kontrollierten Laborumgebungen unter Verwendung von Zellen oder Geweben durchgeführt werden. Es ist wichtig zu beachten, dass diese Produkte nicht als Arzneimittel oder Medikamente eingestuft sind und keine Zulassung der FDA für die Vorbeugung, Behandlung oder Heilung von medizinischen Zuständen, Beschwerden oder Krankheiten erhalten haben. Wir müssen betonen, dass jede Form der körperlichen Einführung dieser Produkte in Menschen oder Tiere gesetzlich strikt untersagt ist. Es ist unerlässlich, sich an diese Richtlinien zu halten, um die Einhaltung rechtlicher und ethischer Standards in Forschung und Experiment zu gewährleisten.