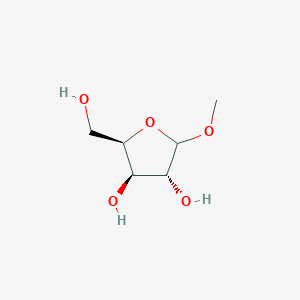
Methyl-D-xylofuranoside
Übersicht
Beschreibung
Methyl-D-xylofuranoside (CAS No. 13039-65-1) is a methylated derivative of D-xylose, where the hydroxyl group at the anomeric carbon (C1) of the furanose ring is replaced by a methoxy group. Its molecular formula is C₆H₁₂O₅, and it exists in a five-membered furanose ring conformation . This compound is critical in carbohydrate chemistry for studying glycosylation reactions and as a precursor in synthesizing complex saccharides. Structural characterization via NMR (e.g., ¹H and ¹³C) has been pivotal in confirming its stereochemistry, with distinct chemical shifts differentiating it from its pyranoside counterpart .
Vorbereitungsmethoden
Synthetic Routes and Reaction Conditions: Methyl-D-xylofuranoside can be synthesized from D-xylose through a glycosylation reaction. One common method involves the reaction of D-xylose with methanol in the presence of an acid catalyst, such as hydrochloric acid or sulfuric acid. The reaction typically proceeds under reflux conditions, and the product is purified through crystallization or chromatography.
Industrial Production Methods: Industrial production of this compound follows similar synthetic routes but on a larger scale. The process involves the use of industrial-grade methanol and acid catalysts, with optimized reaction conditions to maximize yield and purity. The product is then subjected to rigorous purification processes to meet industrial standards.
Types of Reactions:
Oxidation: this compound can undergo oxidation reactions, where the hydroxyl groups are oxidized to form corresponding carbonyl compounds. Common oxidizing agents include potassium permanganate and chromium trioxide.
Reduction: Reduction reactions can convert the carbonyl groups back to hydroxyl groups. Sodium borohydride and lithium aluminum hydride are typical reducing agents used.
Substitution: The methoxy group can be substituted with other functional groups through nucleophilic substitution reactions. For example, treatment with strong nucleophiles like sodium methoxide can lead to the formation of different glycosides.
Common Reagents and Conditions:
Oxidation: Potassium permanganate in aqueous solution under mild heating.
Reduction: Sodium borohydride in methanol at room temperature.
Substitution: Sodium methoxide in methanol under reflux conditions.
Major Products:
Oxidation: Formation of this compound derivatives with carbonyl groups.
Reduction: Regeneration of hydroxyl groups, leading to the formation of reduced this compound.
Substitution: Formation of various glycosides depending on the nucleophile used.
Wissenschaftliche Forschungsanwendungen
Medicinal Chemistry Applications
MDF has potential therapeutic applications, particularly in the context of cardiovascular health. Recent research indicates that methyl β-D-xylopyranoside exhibits cardioprotective effects by targeting specific hub genes associated with myocardial infarction. In-silico studies have demonstrated its binding affinity to proteins involved in key signaling pathways related to heart health .
Case Study: Cardioprotection
- Research Focus : The study investigated the molecular docking of MDF against hub genes such as CASP3 and STAT3.
- Findings : MDF showed promising results in modulating pathways linked to cardiac protection, suggesting its potential as a therapeutic agent for heart diseases .
Enzyme Induction
MDF has been identified as an effective inducer of xylanase production in certain microbial strains. For example, a study demonstrated that the presence of MDF enhanced xylanase synthesis in Cellulomonas flavigena, indicating its role as a substrate for enzyme induction .
Case Study: Xylanase Production
- Research Focus : The influence of MDF on xylanase induction.
- Findings : The study concluded that MDF significantly increased enzyme yields when used with appropriate substrates such as sugarcane bagasse .
Material Science Applications
In material science, MDF is explored for its utility in developing biodegradable polymers and as a protective group in organic synthesis. Its chemical properties allow it to serve as a versatile protecting group for pentopyranosides during chemical reactions .
Case Study: Protective Group Utility
- Research Focus : The use of MDF as a protecting group in synthetic chemistry.
- Findings : The compound facilitated efficient synthesis routes, enhancing the yield and purity of target compounds during organic transformations .
Wirkmechanismus
The mechanism of action of methyl-D-xylofuranoside involves its interaction with specific enzymes and receptors in biological systems. As a glycoside, it can be hydrolyzed by glycosidases to release D-xylose and methanol. The released D-xylose can then enter metabolic pathways, such as the pentose phosphate pathway, contributing to cellular energy production and biosynthesis.
Vergleich Mit ähnlichen Verbindungen
Structural Isomers and Derivatives
Methyl-D-xylofuranoside belongs to the family of methyl pentofuranosides. Key structural analogs include:
Methyl-β-D-ribofuranoside (CAS 7473-45-2)
- Molecular Formula: C₆H₁₂O₅ (identical to this compound).
- Structural Differences: The hydroxyl groups on the furanose ring are positioned at C2, C3, and C4 in ribofuranoside, whereas this compound has hydroxyls at C2, C3, and C5. This positional variance alters hydrogen-bonding patterns and reactivity .
Methyl-2-deoxy-β-D-ribofuranoside
- Molecular Formula : C₆H₁₂O₄.
- Key Difference: Lacks a hydroxyl group at C2, reducing steric hindrance and enhancing nucleophilic reactivity at the anomeric center. This derivative is widely used in nucleoside synthesis .
Methyl-D-xylopyranoside
- Ring Form: Six-membered pyranose ring vs. furanose.
- NMR Data: Distinct ¹H and ¹³C shifts arise from ring size differences. For instance, the anomeric proton in the furanoside form typically resonates at δ 5.2–5.4 ppm, whereas the pyranoside form appears at δ 4.5–4.7 ppm .
Spectroscopic and Physical Properties
NMR Chemical Shifts
Compound | ¹H NMR (δ, ppm) | ¹³C NMR (δ, ppm) | Reference |
---|---|---|---|
This compound | 5.3 (anomeric H) | 105.2 (C1) | |
Methyl-D-xylopyranoside | 4.6 (anomeric H) | 97.8 (C1) | |
Methyl-β-D-ribofuranoside | 5.1 (anomeric H) | 103.5 (C1) |
The furanoside form exhibits downfield shifts for the anomeric proton due to the ring’s increased electron-withdrawing effect compared to pyranosides .
Molecular Weight and Solubility
Compound | Molecular Weight | Solubility (in Water) |
---|---|---|
This compound | 164.16 g/mol | Moderate |
Methyl-β-D-ribofuranoside | 164.16 g/mol | High |
Methyl-2-deoxy-β-D-ribofuranoside | 148.16 g/mol | Low |
Ribofuranosides generally exhibit higher solubility due to favorable hydroxyl group orientation .
Research Findings and Key Insights
- Ring Conformation: The furanose ring in this compound adopts a twist conformation, influencing its reactivity compared to pyranosides .
- Thermodynamic Stability: Pyranoside forms are generally more stable, but furanosides dominate under kinetic control during synthesis .
- Derivative Utility: Acetylation at specific hydroxyl groups (e.g., C5 in gulofuranosides) modifies solubility and crystallinity, aiding purification .
Table 1: Structural Comparison of Methyl Pentofuranosides
Compound | CAS No. | Hydroxyl Positions | Ring Type |
---|---|---|---|
This compound | 13039-65-1 | C2, C3, C5 | Furanose |
Methyl-β-D-ribofuranoside | 7473-45-2 | C2, C3, C4 | Furanose |
Methyl-D-xylopyranoside | - | C2, C3, C4, C5 | Pyranose |
Table 2: Key Physical Properties
Compound | Molecular Weight | Anomeric ¹H Shift (ppm) |
---|---|---|
This compound | 164.16 | 5.3 |
Methyl-β-D-ribofuranoside | 164.16 | 5.1 |
Methyl-D-xylopyranoside | 164.16 | 4.6 |
Biologische Aktivität
Methyl-D-xylofuranoside (MDF) is a furanoside derivative of D-xylose, recognized for its potential biological activities and applications in medicinal chemistry. This article provides a comprehensive overview of the biological activity of MDF, including its immunomodulatory effects, cytotoxicity against cancer cells, and implications in drug development.
Chemical Structure and Properties
This compound is characterized by its furanose ring structure, which is a five-membered cyclic form of the sugar. The compound's structure influences its biological interactions, making it a subject of interest in various pharmacological studies.
Immunomodulatory Effects
Recent research has highlighted the immunomodulatory properties of MDF, particularly in the context of mycobacterial infections. A study focusing on lipoarabinomannan (LAM) from Mycobacterium tuberculosis revealed that residues similar to MDF can modulate immune responses. Specifically, synthetic disaccharides containing 5-deoxy-5-methylthio-xylofuranose residues exhibited modest inhibitory effects on cytokine production (TNF-alpha and IL-12p70) when induced by interferon-gamma and Staphylococcus aureus . This suggests that MDF or its derivatives may play a role in regulating immune responses during infections.
Pharmacological Applications
The pharmacological potential of MDF extends beyond immunomodulation and cytotoxicity. In silico studies have identified MDF as a candidate for drug development due to its ability to interact with key proteins involved in myocardial infarction pathways. Molecular docking studies suggest that MDF can bind to hub genes such as CASP3, STAT3, and HSP90AA1, indicating its potential role in cardioprotection . This highlights the importance of further investigating the therapeutic applications of MDF in cardiovascular health.
Summary of Research Findings
Case Studies
- Immunomodulatory Role : The study on synthetic disaccharides derived from LAM showed that while they did not induce pro-inflammatory cytokines, they inhibited their production under certain conditions. This suggests that MDF may have a protective role in inflammatory responses associated with infections .
- Cytotoxicity Assessment : Research involving methyl β-D-xylopyranoside indicated significant cytotoxicity against various cancer cell lines, suggesting that structural modifications could enhance these effects .
- Cardiovascular Implications : In silico analyses demonstrated that MDF interacts with critical genes involved in heart disease pathways, warranting further exploration into its therapeutic potential for cardiovascular conditions .
Q & A
Basic Research Questions
Q. What are the established synthetic routes for Methyl-D-xylofuranoside, and how can researchers optimize yield and purity?
this compound is typically synthesized via acid-catalyzed methanolysis of D-xylose. Optimization involves:
- Temperature control : Elevated temperatures (60–80°C) improve reaction rates but may increase side products.
- Catalyst selection : HCl or H₂SO₄ at 0.1–0.5 M concentrations are common; neutralization with ion-exchange resins post-reaction minimizes degradation.
- Purification : Column chromatography (silica gel, ethyl acetate/methanol eluent) or recrystallization ensures high purity (>95%). Validate purity via TLC (Rf comparison) and NMR (absence of anomeric proton splitting) .
Q. What spectroscopic techniques are essential for characterizing this compound, and how should data be interpreted?
Key techniques include:
- NMR : ¹H NMR (anomeric proton at δ 4.8–5.2 ppm), ¹³C NMR (C1 at ~105 ppm for furanoside), and 2D COSY/HSQC to confirm ring conformation.
- IR : O-H stretches (3200–3500 cm⁻¹) and C-O-C vibrations (1100 cm⁻¹).
- Mass spectrometry : ESI-MS ([M+Na]⁺ ≈ 197 m/z) for molecular weight confirmation. Cross-reference with databases like SciFinder® for spectral validation .
Q. How should researchers design stability studies for this compound under different storage conditions?
Follow ICH Q1A guidelines:
- Accelerated testing : Expose samples to 40°C/75% RH for 6 months; monitor degradation via HPLC (C18 column, 0.1% H₃PO₃ mobile phase).
- Kinetic analysis : Plot degradation rates vs. Arrhenius models to predict shelf life. Include controls for hygroscopicity and photostability .
Advanced Research Questions
Q. How can computational modeling predict the glycosidic bond stability of this compound under varying pH conditions?
- Density Functional Theory (DFT) : Calculate bond dissociation energies for the glycosidic linkage at different protonation states.
- Molecular Dynamics (MD) : Simulate solvation effects in aqueous buffers (pH 2–10) to identify hydrolysis-prone conformers.
- Validation : Compare with experimental kinetic data (HPLC-monitored hydrolysis rates) to refine computational parameters .
Q. What strategies resolve contradictions in reported bioactivity data of this compound across studies?
- Meta-analysis framework : Use EFSA’s systematic review methodology to assess variables:
Q. What in silico approaches predict the interaction of this compound with carbohydrate-binding proteins?
- Molecular docking : Use AutoDock Vina to screen against lectins (e.g., concanavalin A) with flexible side-chain sampling.
- Binding free energy : Calculate ΔG via MM-PBSA/GBSA workflows in AMBER.
- Experimental validation : Surface Plasmon Resonance (SPR) or Isothermal Titration Calorimetry (ITC) for affinity measurements .
Q. How to assess the environmental impact of this compound using life cycle assessment (LCA) methodologies?
- Inventory phase : Quantify solvent waste (methanol, HCl) and energy use during synthesis.
- Impact assessment : Use SimaPro software with ReCiPe endpoints (ecotoxicity, global warming potential).
- Mitigation : Propose green chemistry alternatives (e.g., enzymatic catalysis) to reduce E-factor scores .
Q. Methodological Guidance
Q. How to conduct a comprehensive literature review on this compound using academic databases?
- Search strategy :
- PubMed/Web of Science : Combine terms "this compound" AND ("synthesis" OR "metabolism") with Boolean operators.
- SciFinder® : Use CAS Registry Number (13039-65-1) for precise indexing. Exclude patents and non-English studies.
- Screening : Apply PRISMA flow diagrams to filter irrelevant hits. Prioritize studies with full spectral data .
Q. What protocols ensure reproducibility in this compound bioactivity assays?
- Standardization :
- Cell culture : Use authenticated lines (e.g., ATCC-certified) with mycoplasma testing.
- Dose-response curves : Include positive controls (e.g., methyl-α-D-glucopyranoside) and triplicate replicates.
- Data reporting : Adhere to MIAME/MIAPE guidelines for metadata (e.g., incubation time, passage number) .
Q. Tables
Table 1. Key Spectral Peaks for this compound Validation
Technique | Key Peaks/Features | Reference Range |
---|---|---|
¹H NMR (D₂O) | δ 4.92 (d, J=3.5 Hz, H1) | |
¹³C NMR | 105.2 ppm (C1), 82.1 ppm (C4) | |
IR | 3400 cm⁻¹ (O-H), 1075 cm⁻¹ (C-O-C) |
Table 2. Common Contaminants in Synthesis and Mitigation Strategies
Contaminant | Source | Removal Method |
---|---|---|
Xylose residues | Incomplete reaction | Prolonged methanolysis |
Methyl esters | Over-acidification | Neutralization (NaHCO₃) |
Eigenschaften
IUPAC Name |
(2R,3R,4R)-2-(hydroxymethyl)-5-methoxyoxolane-3,4-diol | |
---|---|---|
Source | PubChem | |
URL | https://pubchem.ncbi.nlm.nih.gov | |
Description | Data deposited in or computed by PubChem | |
InChI |
InChI=1S/C6H12O5/c1-10-6-5(9)4(8)3(2-7)11-6/h3-9H,2H2,1H3/t3-,4+,5-,6?/m1/s1 | |
Source | PubChem | |
URL | https://pubchem.ncbi.nlm.nih.gov | |
Description | Data deposited in or computed by PubChem | |
InChI Key |
NALRCAPFICWVAQ-IANNHFEVSA-N | |
Source | PubChem | |
URL | https://pubchem.ncbi.nlm.nih.gov | |
Description | Data deposited in or computed by PubChem | |
Canonical SMILES |
COC1C(C(C(O1)CO)O)O | |
Source | PubChem | |
URL | https://pubchem.ncbi.nlm.nih.gov | |
Description | Data deposited in or computed by PubChem | |
Isomeric SMILES |
COC1[C@@H]([C@H]([C@H](O1)CO)O)O | |
Source | PubChem | |
URL | https://pubchem.ncbi.nlm.nih.gov | |
Description | Data deposited in or computed by PubChem | |
Molecular Formula |
C6H12O5 | |
Source | PubChem | |
URL | https://pubchem.ncbi.nlm.nih.gov | |
Description | Data deposited in or computed by PubChem | |
Molecular Weight |
164.16 g/mol | |
Source | PubChem | |
URL | https://pubchem.ncbi.nlm.nih.gov | |
Description | Data deposited in or computed by PubChem | |
Retrosynthesis Analysis
AI-Powered Synthesis Planning: Our tool employs the Template_relevance Pistachio, Template_relevance Bkms_metabolic, Template_relevance Pistachio_ringbreaker, Template_relevance Reaxys, Template_relevance Reaxys_biocatalysis model, leveraging a vast database of chemical reactions to predict feasible synthetic routes.
One-Step Synthesis Focus: Specifically designed for one-step synthesis, it provides concise and direct routes for your target compounds, streamlining the synthesis process.
Accurate Predictions: Utilizing the extensive PISTACHIO, BKMS_METABOLIC, PISTACHIO_RINGBREAKER, REAXYS, REAXYS_BIOCATALYSIS database, our tool offers high-accuracy predictions, reflecting the latest in chemical research and data.
Strategy Settings
Precursor scoring | Relevance Heuristic |
---|---|
Min. plausibility | 0.01 |
Model | Template_relevance |
Template Set | Pistachio/Bkms_metabolic/Pistachio_ringbreaker/Reaxys/Reaxys_biocatalysis |
Top-N result to add to graph | 6 |
Feasible Synthetic Routes
Haftungsausschluss und Informationen zu In-Vitro-Forschungsprodukten
Bitte beachten Sie, dass alle Artikel und Produktinformationen, die auf BenchChem präsentiert werden, ausschließlich zu Informationszwecken bestimmt sind. Die auf BenchChem zum Kauf angebotenen Produkte sind speziell für In-vitro-Studien konzipiert, die außerhalb lebender Organismen durchgeführt werden. In-vitro-Studien, abgeleitet von dem lateinischen Begriff "in Glas", beinhalten Experimente, die in kontrollierten Laborumgebungen unter Verwendung von Zellen oder Geweben durchgeführt werden. Es ist wichtig zu beachten, dass diese Produkte nicht als Arzneimittel oder Medikamente eingestuft sind und keine Zulassung der FDA für die Vorbeugung, Behandlung oder Heilung von medizinischen Zuständen, Beschwerden oder Krankheiten erhalten haben. Wir müssen betonen, dass jede Form der körperlichen Einführung dieser Produkte in Menschen oder Tiere gesetzlich strikt untersagt ist. Es ist unerlässlich, sich an diese Richtlinien zu halten, um die Einhaltung rechtlicher und ethischer Standards in Forschung und Experiment zu gewährleisten.