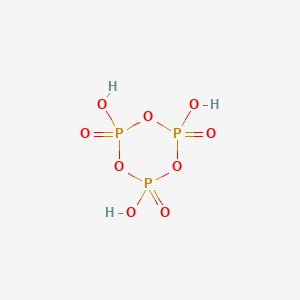
Trimetaphosphoric acid
Übersicht
Beschreibung
. Sie gehört zur Klasse der Nichtmetallphosphate und zeichnet sich durch eine zyklische Struktur aus, die aus drei miteinander verbundenen Phosphat-Einheiten besteht . Diese Verbindung ist bemerkenswert für ihre einzigartige Ringstruktur und ihre Rolle in verschiedenen chemischen und industriellen Anwendungen.
Vorbereitungsmethoden
Synthesewege und Reaktionsbedingungen: Trimetaphosphorsäure kann durch verschiedene Verfahren synthetisiert werden. Ein üblicher Ansatz beinhaltet die thermische Dehydration von Orthophosphorsäure. Dieser Prozess erfordert typischerweise das Erhitzen von Orthophosphorsäure auf hohe Temperaturen, etwa 300 °C, um die Bildung der zyklischen Trimetaphosphat-Struktur zu induzieren .
Industrielle Produktionsverfahren: Industriell wird Trinatriumtrimetaphosphat durch Erhitzen von Natriumdihydrogenphosphat auf etwa 550 °C hergestellt. Das resultierende Trimetaphosphat wird dann in Wasser gelöst und durch Zugabe von Natriumchlorid ausgefällt, wodurch die Hexahydrat-Form entsteht .
Analyse Chemischer Reaktionen
Reaktionstypen: Trimetaphosphorsäure unterliegt verschiedenen chemischen Reaktionen, darunter Hydrolyse-, Ringöffnungs- und Kondensationsreaktionen. Sie ist besonders reaktiv mit Nukleophilen, die die Phosphoratome im Ring angreifen können, was zu einer Ringöffnung und der Bildung linearer Polyphosphate führt .
Häufige Reagenzien und Bedingungen: Häufige Reagenzien, die in Reaktionen mit Trimetaphosphorsäure verwendet werden, sind Wasser, Amine und andere Nukleophile. Die Reaktionen laufen oft unter milden Bedingungen ab, wie z. B. bei Raumtemperatur oder leicht erhöhten Temperaturen .
Hauptsächlich gebildete Produkte: Die Hauptprodukte, die aus den Reaktionen von Trimetaphosphorsäure entstehen, sind lineare Polyphosphate, wie z. B. Natriumtriphosphat, und verschiedene substituierte Phosphate, abhängig vom verwendeten Nukleophil .
Wissenschaftliche Forschungsanwendungen
Trimetaphosphorsäure hat eine breite Palette von Anwendungen in der wissenschaftlichen Forschung. In der Chemie wird sie als Reagenz für die Synthese anderer Phosphorverbindungen verwendet. In der Biologie wurde sie auf ihre mögliche Rolle in der präbiotischen Chemie und dem Ursprung des Lebens untersucht . In der Medizin wird sie auf ihre potenzielle Verwendung in Medikamenten-Abgabesystemen und als Bestandteil in Zahnzementen untersucht . Industriell wird sie in der Wasseraufbereitung, in Reinigungsmitteln und als sequestierender Stoff in der Lebensmittelverarbeitung eingesetzt .
Wirkmechanismus
Der Wirkmechanismus von Trimetaphosphorsäure beinhaltet ihre Fähigkeit, stabile Komplexe mit Metallionen und anderen Kationen zu bilden. Diese Eigenschaft macht sie nützlich in Anwendungen wie der Wasseraufbereitung, wo sie Metallionen sequestieren und die Bildung von Kesselstein verhindern kann . Die zyklische Struktur der Trimetaphosphorsäure ermöglicht es ihr, mit verschiedenen molekularen Zielstrukturen, einschließlich Enzymen und anderen Proteinen, zu interagieren, wodurch ihre Aktivität und Stabilität möglicherweise beeinflusst wird .
Wirkmechanismus
The mechanism of action of trimetaphosphoric acid involves its ability to form stable complexes with metal ions and other cations. This property makes it useful in applications such as water treatment, where it can sequester metal ions and prevent scale formation . The cyclic structure of this compound allows it to interact with various molecular targets, including enzymes and other proteins, potentially influencing their activity and stability .
Vergleich Mit ähnlichen Verbindungen
Trimetaphosphorsäure ist unter den Phosphorsäuren aufgrund ihrer zyklischen Struktur einzigartig. Ähnliche Verbindungen sind Orthophosphorsäure (H₃PO₄), Pyrophosphorsäure (H₄P₂O₇) und andere Metaphosphorsäuren mit unterschiedlichen Ringgrößen . Im Vergleich zu diesen Verbindungen weist Trimetaphosphorsäure unterschiedliche Reaktivitäts- und Stabilitätseigenschaften auf, wodurch sie für bestimmte Anwendungen geeignet ist, bei denen andere Phosphorsäuren möglicherweise nicht so effektiv sind .
Biologische Aktivität
Trimetaphosphoric acid (TMPA), a cyclic phosphate compound, has garnered attention for its diverse biological activities, particularly in dental health and mineralization processes. This article delves into the biological effects of TMPA, supported by relevant research findings, case studies, and data tables to provide a comprehensive overview.
This compound is represented by the chemical formula and is known for its ability to form complexes with metal ions, which can influence various biological processes. Its structure allows it to interact with biological systems effectively, making it a subject of interest in biomedical research.
Biological Activity Overview
1. Dental Health:
TMPA has been studied for its role in enhancing the remineralization of dental enamel. Research indicates that incorporating TMPA into fluoride toothpaste can significantly improve the remineralization capacity of treated enamel surfaces.
-
Case Study:
A clinical trial involving 4196 children assessed the efficacy of fluoride toothpastes with and without TMPA. The study found no significant difference in caries increment between toothpaste formulations containing TMPA and those without it, suggesting that while TMPA may not enhance fluoride efficacy significantly, it remains a valuable component in dental health products . - Data Table: Efficacy of Toothpaste Formulations
2. Interaction with Hydroxyapatite:
Research has shown that sodium trimetaphosphate (a salt form of TMPA) influences the structure and dissolution properties of carbonated hydroxyapatite (CHA), a key component in bone and dental structures.
- Study Findings:
In experiments where CHA was treated with varying concentrations of TMPA and fluoride, higher calcium-to-phosphorus ratios were observed at specific TMP concentrations. This suggests that TMPA can enhance the stability and mineral content of CHA, which is crucial for dental applications .
The biological activity of TMPA can be attributed to several mechanisms:
- Mineralization Enhancement: TMPA promotes the deposition of minerals in dental enamel, aiding in the repair of early carious lesions.
- Complex Formation: It forms stable complexes with calcium and phosphate ions, facilitating their incorporation into the mineral matrix.
- pH Modulation: By influencing local pH levels during mineralization processes, TMPA can enhance the solubility and bioavailability of essential minerals.
Toxicological Considerations
While TMPA is generally regarded as safe for use in dental products, there are considerations regarding its toxicity at high concentrations. Studies indicate that exposure to phosphoric acid derivatives can lead to irritation or adverse effects in sensitive individuals . Therefore, appropriate safety assessments are necessary when formulating products containing TMPA.
Eigenschaften
IUPAC Name |
2,4,6-trihydroxy-1,3,5,2λ5,4λ5,6λ5-trioxatriphosphinane 2,4,6-trioxide | |
---|---|---|
Details | Computed by Lexichem TK 2.7.0 (PubChem release 2021.10.14) | |
Source | PubChem | |
URL | https://pubchem.ncbi.nlm.nih.gov | |
Description | Data deposited in or computed by PubChem | |
InChI |
InChI=1S/H3O9P3/c1-10(2)7-11(3,4)9-12(5,6)8-10/h(H,1,2)(H,3,4)(H,5,6) | |
Details | Computed by InChI 1.0.6 (PubChem release 2021.10.14) | |
Source | PubChem | |
URL | https://pubchem.ncbi.nlm.nih.gov | |
Description | Data deposited in or computed by PubChem | |
InChI Key |
AZSFNUJOCKMOGB-UHFFFAOYSA-N | |
Details | Computed by InChI 1.0.6 (PubChem release 2021.10.14) | |
Source | PubChem | |
URL | https://pubchem.ncbi.nlm.nih.gov | |
Description | Data deposited in or computed by PubChem | |
Canonical SMILES |
OP1(=O)OP(=O)(OP(=O)(O1)O)O | |
Details | Computed by OEChem 2.3.0 (PubChem release 2021.10.14) | |
Source | PubChem | |
URL | https://pubchem.ncbi.nlm.nih.gov | |
Description | Data deposited in or computed by PubChem | |
Molecular Formula |
H3O9P3 | |
Details | Computed by PubChem 2.2 (PubChem release 2021.10.14) | |
Source | PubChem | |
URL | https://pubchem.ncbi.nlm.nih.gov | |
Description | Data deposited in or computed by PubChem | |
Related CAS |
37267-86-0 (cpd with unknown MF), 7785-84-4 (tri-hydrochloride salt) | |
Record name | Trimetaphosphoric acid | |
Source | ChemIDplus | |
URL | https://pubchem.ncbi.nlm.nih.gov/substance/?source=chemidplus&sourceid=0013566251 | |
Description | ChemIDplus is a free, web search system that provides access to the structure and nomenclature authority files used for the identification of chemical substances cited in National Library of Medicine (NLM) databases, including the TOXNET system. | |
DSSTOX Substance ID |
DTXSID40159485 | |
Record name | Trimetaphosphoric acid | |
Source | EPA DSSTox | |
URL | https://comptox.epa.gov/dashboard/DTXSID40159485 | |
Description | DSSTox provides a high quality public chemistry resource for supporting improved predictive toxicology. | |
Molecular Weight |
239.94 g/mol | |
Details | Computed by PubChem 2.2 (PubChem release 2021.10.14) | |
Source | PubChem | |
URL | https://pubchem.ncbi.nlm.nih.gov | |
Description | Data deposited in or computed by PubChem | |
CAS No. |
13566-25-1 | |
Record name | Trimetaphosphoric acid | |
Source | CAS Common Chemistry | |
URL | https://commonchemistry.cas.org/detail?cas_rn=13566-25-1 | |
Description | CAS Common Chemistry is an open community resource for accessing chemical information. Nearly 500,000 chemical substances from CAS REGISTRY cover areas of community interest, including common and frequently regulated chemicals, and those relevant to high school and undergraduate chemistry classes. This chemical information, curated by our expert scientists, is provided in alignment with our mission as a division of the American Chemical Society. | |
Explanation | The data from CAS Common Chemistry is provided under a CC-BY-NC 4.0 license, unless otherwise stated. | |
Record name | Trimetaphosphoric acid | |
Source | ChemIDplus | |
URL | https://pubchem.ncbi.nlm.nih.gov/substance/?source=chemidplus&sourceid=0013566251 | |
Description | ChemIDplus is a free, web search system that provides access to the structure and nomenclature authority files used for the identification of chemical substances cited in National Library of Medicine (NLM) databases, including the TOXNET system. | |
Record name | Trimetaphosphoric acid | |
Source | EPA DSSTox | |
URL | https://comptox.epa.gov/dashboard/DTXSID40159485 | |
Description | DSSTox provides a high quality public chemistry resource for supporting improved predictive toxicology. | |
Record name | TRIMETAPHOSPHORIC ACID | |
Source | FDA Global Substance Registration System (GSRS) | |
URL | https://gsrs.ncats.nih.gov/ginas/app/beta/substances/H6C6T4285H | |
Description | The FDA Global Substance Registration System (GSRS) enables the efficient and accurate exchange of information on what substances are in regulated products. Instead of relying on names, which vary across regulatory domains, countries, and regions, the GSRS knowledge base makes it possible for substances to be defined by standardized, scientific descriptions. | |
Explanation | Unless otherwise noted, the contents of the FDA website (www.fda.gov), both text and graphics, are not copyrighted. They are in the public domain and may be republished, reprinted and otherwise used freely by anyone without the need to obtain permission from FDA. Credit to the U.S. Food and Drug Administration as the source is appreciated but not required. | |
Retrosynthesis Analysis
AI-Powered Synthesis Planning: Our tool employs the Template_relevance Pistachio, Template_relevance Bkms_metabolic, Template_relevance Pistachio_ringbreaker, Template_relevance Reaxys, Template_relevance Reaxys_biocatalysis model, leveraging a vast database of chemical reactions to predict feasible synthetic routes.
One-Step Synthesis Focus: Specifically designed for one-step synthesis, it provides concise and direct routes for your target compounds, streamlining the synthesis process.
Accurate Predictions: Utilizing the extensive PISTACHIO, BKMS_METABOLIC, PISTACHIO_RINGBREAKER, REAXYS, REAXYS_BIOCATALYSIS database, our tool offers high-accuracy predictions, reflecting the latest in chemical research and data.
Strategy Settings
Precursor scoring | Relevance Heuristic |
---|---|
Min. plausibility | 0.01 |
Model | Template_relevance |
Template Set | Pistachio/Bkms_metabolic/Pistachio_ringbreaker/Reaxys/Reaxys_biocatalysis |
Top-N result to add to graph | 6 |
Feasible Synthetic Routes
Haftungsausschluss und Informationen zu In-Vitro-Forschungsprodukten
Bitte beachten Sie, dass alle Artikel und Produktinformationen, die auf BenchChem präsentiert werden, ausschließlich zu Informationszwecken bestimmt sind. Die auf BenchChem zum Kauf angebotenen Produkte sind speziell für In-vitro-Studien konzipiert, die außerhalb lebender Organismen durchgeführt werden. In-vitro-Studien, abgeleitet von dem lateinischen Begriff "in Glas", beinhalten Experimente, die in kontrollierten Laborumgebungen unter Verwendung von Zellen oder Geweben durchgeführt werden. Es ist wichtig zu beachten, dass diese Produkte nicht als Arzneimittel oder Medikamente eingestuft sind und keine Zulassung der FDA für die Vorbeugung, Behandlung oder Heilung von medizinischen Zuständen, Beschwerden oder Krankheiten erhalten haben. Wir müssen betonen, dass jede Form der körperlichen Einführung dieser Produkte in Menschen oder Tiere gesetzlich strikt untersagt ist. Es ist unerlässlich, sich an diese Richtlinien zu halten, um die Einhaltung rechtlicher und ethischer Standards in Forschung und Experiment zu gewährleisten.