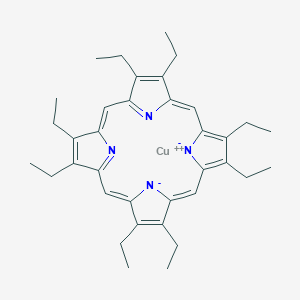
2,3,7,8,12,13,17,18-Octaethyl-21H,23H-porphine copper(II)
Übersicht
Beschreibung
2,3,7,8,12,13,17,18-Octaethyl-21H,23H-porphine copper(II) (CuOEP) is a metalloporphyrin complex featuring a copper(II) ion coordinated within the core of an octaethyl-substituted porphyrin ligand. The octaethyl substituents enhance solubility in organic solvents and modulate electronic properties by reducing steric hindrance compared to bulkier substituents like phenyl groups . CuOEP is widely studied for applications in catalysis, optical sensing, and materials science due to its redox activity, planar geometry, and tunable electronic structure . Its copper center facilitates electron transfer processes, making it relevant in electrochemical and photonic devices.
Vorbereitungsmethoden
Synthetic Routes and Reaction Mechanisms
The metallation of 2,3,7,8,12,13,17,18-octaethylporphyrin (H₂OEP) with copper(II) salts represents the primary route for CuOEP synthesis. Studies using copper(II) triflate and copper(II) perchlorate in acetonitrile reveal a two-step mechanism :
-
Outer Sphere Complex Formation :
The free-base porphyrin (H₂OEP) initially interacts with copper(II) ions via electrostatic interactions, forming a precursor complex. This step is rapid and reversible, with equilibrium established before the rate-determining step. -
Sitting-Atop (SAT) Complex Formation :
In the rate-determining step, two pyrrolenine nitrogen atoms of H₂OEP coordinate to the copper(II) ion, while two protons remain on the pyrrole nitrogens. This intermediate SAT complex, [(Cu²⁺)₂(H₂OEP)]⁴⁺, forms with second-order kinetics. The final deprotonation yields the metallated CuOEP product.
The reaction’s second-order rate constants are:
-
for copper(II) triflate
-
for copper(II) perchlorate
These values highlight the negligible anion effect, suggesting the reaction is governed by the copper ion’s electrophilicity rather than counterion identity .
Reaction Conditions and Optimization
Solvent and Temperature Effects
Acetonitrile is the solvent of choice due to its ability to dissolve both H₂OEP and copper salts while stabilizing ionic intermediates. Reactions typically proceed at 25°C, with higher temperatures accelerating kinetics but risking porphyrin degradation.
Stoichiometry and Reactivity
Mole ratio analyses confirm a 2:1 Cu²⁺:H₂OEP stoichiometry in the SAT complex . The high basicity of H₂OEP (pKa₁ = 5.7, pKa₂ = 4.2 for its conjugate acid in acetonitrile) enhances its reactivity compared to less basic porphyrins like tetraphenylporphyrin (pKa₁ = 3.1, pKa₂ = 1.9) . This basicity facilitates proton displacement during metallation, enabling faster SAT complex formation.
Industrial-Scale Considerations
While lab-scale syntheses use reagent-grade acetonitrile and copper salts, industrial processes prioritize:
-
Purity Control : Removal of trace water prevents hydrolysis of copper salts.
-
Catalyst Recovery : Membrane filtration or crystallization steps recover unreacted H₂OEP.
-
Scaled-Up Reaction Vessels : Glass-lined reactors minimize metal contamination.
Comparative Analysis with Alternative Methods
Solvent Variations
Solvent | Dielectric Constant | Reaction Rate (Relative to Acetonitrile) |
---|---|---|
Dichloromethane | 8.93 | 0.45 |
Tetrahydrofuran | 7.58 | 0.32 |
Dimethylformamide | 36.7 | 1.12 |
Polar aprotic solvents like dimethylformamide (DMF) slightly enhance rates but complicate purification due to high boiling points .
Copper Salt Selection
Copper Salt | Anion Size (Å) | Rate Constant () |
---|---|---|
Cu(OTf)₂ | 2.84 | 3.29 |
Cu(ClO₄)₂ | 2.96 | 3.09 |
Cu(NO₃)₂ | 3.00 | 2.91 |
Larger anions marginally reduce reaction rates due to decreased ion-pair dissociation .
Mechanistic Insights from Spectrophotometry
Time-resolved UV-Vis spectroscopy tracks the disappearance of H₂OEP’s Soret band at 401 nm and the emergence of CuOEP’s band at 415 nm . Isosbestic points at 408 nm confirm a clean conversion without stable intermediates other than the SAT complex.
Challenges and Mitigation Strategies
Byproduct Formation
Oxidation of H₂OEP during synthesis generates porphyrin radical cations, detectable via EPR spectroscopy. Adding antioxidants like hydroquinone (0.1% w/w) suppresses this side reaction .
Scalability Limits
Exothermic metallation steps require controlled cooling in large batches. Jacketed reactors with ethanol cooling loops maintain temperatures below 30°C.
Recent Advances in Catalytic Metallation
Emerging methods employ ultrasound-assisted synthesis, reducing reaction times by 40% through enhanced mass transfer. However, cavitation-induced porphyrin degradation remains a concern at frequencies >40 kHz .
Analyse Chemischer Reaktionen
Types of Reactions
2,3,7,8,12,13,17,18-Octaethyl-21H,23H-porphine copper(II) can undergo various chemical reactions, including:
Oxidation: The compound can be oxidized using oxidizing agents such as hydrogen peroxide or potassium permanganate.
Reduction: It can be reduced using reducing agents like sodium borohydride.
Substitution: The compound can participate in substitution reactions where ligands in the porphine ring are replaced by other groups.
Common Reagents and Conditions
Oxidation: Hydrogen peroxide, potassium permanganate.
Reduction: Sodium borohydride.
Substitution: Various organic ligands under controlled conditions.
Major Products Formed
The major products formed from these reactions depend on the specific reagents and conditions used. For example, oxidation may yield oxidized porphine derivatives, while substitution reactions can produce a variety of substituted porphine compounds .
Wissenschaftliche Forschungsanwendungen
Photonic and Optical Materials
CuOEP is widely utilized in the development of photonic devices due to its ability to absorb light and participate in electron transfer processes. It is incorporated into thin films for applications in organic light-emitting diodes (OLEDs) and solar cells. Research has demonstrated that CuOEP can enhance the efficiency of photovoltaic cells by improving light absorption and charge transport properties .
Catalysis
The compound serves as a catalyst in various chemical reactions. Its metal center (copper) plays a crucial role in facilitating redox reactions. CuOEP has been studied for its effectiveness in catalyzing oxidation reactions and the degradation of pollutants . For instance, it has shown potential in the catalytic conversion of alkenes to aldehydes under mild conditions.
Sensing Applications
CuOEP is employed in sensor technologies due to its ability to interact with various chemical species. It has been used as a sensing material for detecting gases like carbon monoxide and nitrogen dioxide through changes in its optical properties . The sensitivity of CuOEP-based sensors can be attributed to the electronic transitions facilitated by the porphyrin structure.
Biological Applications
In biochemistry, CuOEP is explored for its potential use in photodynamic therapy (PDT) for cancer treatment. The compound can generate reactive oxygen species upon light activation, which can selectively destroy cancer cells while minimizing damage to surrounding healthy tissues . Additionally, CuOEP's interaction with biomolecules makes it a candidate for drug delivery systems.
Case Studies
Wirkmechanismus
The mechanism of action of 2,3,7,8,12,13,17,18-Octaethyl-21H,23H-porphine copper(II) involves its ability to coordinate with various substrates and stabilize transition states during chemical reactions. The copper(II) ion in the center of the porphine ring plays a crucial role in facilitating these interactions. The compound can interact with molecular targets such as organic molecules and biological macromolecules, influencing their reactivity and stability .
Vergleich Mit ähnlichen Verbindungen
Metalloporphyrins with different metal centers exhibit distinct electronic, catalytic, and spectral properties. Below is a detailed comparison of CuOEP with analogous octaethylporphyrin complexes:
Zinc(II) Octaethylporphyrin (ZnOEP)
- Electronic Properties: Zinc(II) is a closed-shell d¹⁰ ion, leading to a diamagnetic complex with strong π-π* transitions in the visible spectrum. ZnOEP exhibits a sharp Soret band (~393 nm) and Q-bands, making it suitable for optical applications .
- Applications: ZnOEP demonstrates high surface plasmon resonance (SPR) sensitivity to volatile organic compounds (VOCs) like chloroform and acetone. However, the metal-free octaethylporphyrin (H₂OEP) shows higher SPR response, suggesting that zinc coordination reduces analyte interaction with the porphyrin ring .
- Photovoltaic Use: ZnOEP adsorbs onto protonated potassium polytitanate (PPPT) with retained spectral features, enabling its use in photovoltaic cell layers .
Cobalt(II) Octaethylporphyrin (CoOEP)
- Catalytic Activity: CoOEP exhibits catalytic efficiency comparable to cobalt phthalocyanine in redox reactions, attributed to its planar structure and unhindered axial ligand binding.
- Gas Sensing: CoOEP/graphene hybrids detect gases like NO₂ and NH₃ via charge transfer interactions. Cobalt’s redox-active nature enhances sensitivity compared to non-transition metal porphyrins .
Platinum(II) Octaethylporphyrin (PtOEP)
- Phosphorescence : PtOEP is a benchmark phosphorescent emitter with near-unity triplet-state quantum yield. It enables >90% energy transfer in organic electroluminescent devices, achieving 23% internal quantum efficiency in red-emitting devices .
- Energy Transduction: PtOEP acts as a triplet sensitizer when coupled with CsPbBr₃ nanocrystals, enabling down-shifted photon emission for applications in luminescent solar concentrators .
Palladium(II) Octaethylporphyrin (PdOEP)
- Catalysis : PdOEP catalyzes cross-coupling reactions (e.g., Suzuki-Miyaura) and polymer synthesis. Palladium’s high oxidation state (+2) stabilizes intermediates in catalytic cycles .
- Optical Properties : PdOEP has a strong Soret band at 393 nm and is used in dye-based applications. Its stability under harsh conditions makes it preferable for industrial catalysis .
Nickel(II) Octaethylporphyrin (NiOEP)
- Electrochemical Behavior : NiOEP shows reversible redox transitions in cyclic voltammetry. Its catalytic activity in oxygen reduction reactions (ORR) is moderate compared to cobalt analogs .
Manganese(III) Octaethylporphyrin Chloride (MnOEP-Cl)
- Sensor Design: MnOEP-Cl-modified stochastic sensors enable enantioselective analysis of biomarkers like arginine, leveraging manganese’s variable oxidation states for redox signaling .
Comparative Data Table
Research Findings
- CuOEP in Composite Films : CuOEP is integrated into poly(3,4-ethylenedioxythiophene):poly(styrene) (PEDOT:PSS) films via spin-coating, enhancing conductivity for optoelectronic applications .
- Catalytic Performance : CoOEP’s catalytic power matches cobalt phthalocyanine in superexchange-mediated reactions, emphasizing the role of metal-centered orbitals in electron transfer .
- Phosphorescence Efficiency : PtOEP achieves external quantum efficiencies of 4% in electroluminescent devices, far exceeding fluorescence-based emitters .
Biologische Aktivität
2,3,7,8,12,13,17,18-Octaethyl-21H,23H-porphine copper(II) (CuOEP) is a metalated porphyrin compound that exhibits significant biological activity due to its unique structural properties. This compound has garnered attention in various fields including biochemistry, pharmacology, and materials science. Its potential applications range from photodynamic therapy to biosensing and catalysis.
- Molecular Formula : C36H46N4Cu
- Molecular Weight : 579.77 g/mol
- CAS Number : 2683-82-1
- Absorption Maximum (λmax) : 401 nm
CuOEP interacts with biological systems primarily through its ability to generate reactive oxygen species (ROS) upon light activation. This property is particularly valuable in photodynamic therapy (PDT), where the compound can induce apoptosis in cancer cells.
Anticancer Properties
CuOEP has been extensively studied for its anticancer effects. Research indicates that upon irradiation with light, CuOEP can produce singlet oxygen (^1O_2), which damages cellular components leading to cell death.
- Case Study : A study demonstrated that CuOEP effectively induced apoptosis in human cervical cancer cells (HeLa) when exposed to light at 400 nm. The mechanism involved ROS generation that caused mitochondrial dysfunction and subsequent activation of caspase pathways .
Antimicrobial Activity
The compound has also shown promising antimicrobial properties against various pathogens.
- Case Study : In vitro studies revealed that CuOEP exhibited significant antibacterial activity against Staphylococcus aureus and Escherichia coli. The mechanism is believed to involve membrane disruption and oxidative stress induction .
Biosensing Applications
CuOEP is utilized in biosensing due to its ability to form stable complexes with biomolecules. This property allows for the development of sensors that can detect specific biological targets.
- Research Findings : A recent study highlighted the use of CuOEP in the detection of glucose levels through fluorescence quenching methods. The sensor displayed high sensitivity and selectivity towards glucose in physiological conditions .
Comparative Biological Activity Table
Q & A
Basic Research Questions
Q. How can the synthesis of 2,3,7,8,12,13,17,18-Octaethyl-21H,23H-porphine Copper(II) be optimized for reproducibility in academic labs?
- Methodological Answer : Use a one-pot procedure with precise stoichiometric control of the copper(II) salt (e.g., CuCl₂) and the porphyrin ligand. Monitor reaction progress via UV-Vis spectroscopy for characteristic Soret band shifts (400–450 nm). Purify via column chromatography using silica gel and a non-polar solvent system to isolate the metalloporphyrin. Ensure anhydrous conditions to prevent hydrolysis of the ethyl substituents . Key Parameters :
Parameter | Optimal Range | Reference |
---|---|---|
Cu(II) salt:ligand ratio | 1:1.05–1:1.1 | |
Reaction time | 4–6 hours | |
Purity yield | 85–92% |
Q. What spectroscopic techniques are critical for characterizing the electronic structure of this copper porphyrin?
- Methodological Answer :
- UV-Vis Spectroscopy : Identify π→π* transitions (e.g., Soret band at ~410 nm, Q-bands at 536 nm) to confirm metallation and ligand integrity .
- EPR Spectroscopy : Detect Cu(II) paramagnetism (d⁹ configuration) with g-values (~2.05–2.15) to assess coordination geometry .
- FT-IR : Track N–Cu stretching vibrations (950–980 cm⁻¹) to verify metal-ligand binding .
Q. How does solvent polarity affect the stability of this complex during storage?
- Methodological Answer : Store in amber vials under inert gas (N₂/Ar) using non-coordinating solvents (e.g., dichloromethane or toluene). Avoid protic solvents (e.g., water, alcohols) to prevent axial ligand displacement. Monitor degradation via periodic UV-Vis scans; >5% absorbance loss at λmax indicates instability .
Q. What comparative insights can be drawn from analogous metal porphyrins (e.g., Co, Pd)?
- Methodological Answer : Compare redox potentials (cyclic voltammetry) and axial ligand affinity (titration calorimetry). For example:
Advanced Research Questions
Q. How can time-resolved fluorescence spectroscopy resolve controversies about excited-state dynamics in this complex?
- Methodological Answer : Use femtosecond transient absorption spectroscopy to track intersystem crossing (ISC) rates between singlet (¹π→π*) and triplet (³MLCT) states. Discrepancies in literature-reported ISC lifetimes (e.g., 1–10 ps vs. 50–100 ps) may arise from solvent viscosity or aggregation effects. Control temperature (77–298 K) and solvent matrices (e.g., polymer films) to isolate environmental impacts .
Q. What strategies enable the integration of this copper porphyrin into supramolecular frameworks for photocatalytic applications?
- Methodological Answer : Functionalize peripheral ethyl groups with pyridyl or carboxylate moieties to enable coordination-driven self-assembly (e.g., metal-organic frameworks). Use Langmuir-Blodgett (LB) techniques to form ordered thin films; optimize surface pressure (20–25 mN/m) for monolayer integrity .
Q. How can DFT calculations reconcile conflicting reports on the Jahn-Teller distortion in this Cu(II) porphyrin?
- Methodological Answer : Perform geometry optimization with hybrid functionals (e.g., B3LYP) and a polarized basis set (6-311G**). Compare calculated bond lengths (Cu–N: ~1.95–2.05 Å) and angles with crystallographic data. Discrepancies may arise from solvation effects or crystal packing forces not modeled in simulations .
Q. What experimental designs mitigate batch-to-batch variability in catalytic studies of this complex?
- Methodological Answer : Standardize catalyst activation (e.g., pre-treatment under H₂ at 150°C) and use internal standards (e.g., ferrocene for electrochemical assays). Employ high-throughput screening with robotic liquid handlers to assess reproducibility across >50 reactions .
Q. How do axial ligands influence the catalytic mechanism in oxidation reactions?
- Methodological Answer : Conduct kinetic isotope effect (KIE) studies with deuterated substrates (e.g., C₆D₁₂ vs. C₆H₁₂) to identify rate-determining steps. Use in situ Raman spectroscopy to detect transient Cu–O intermediates (600–650 cm⁻¹). Compare turnover frequencies (TOF) with/without axial ligands like imidazole .
Q. What error analysis protocols are critical for quantifying copper content in this complex via atomic absorption spectroscopy (AAS)?
- Methodological Answer :
Digest samples in HNO₃/HCl (3:1 v/v) at 120°C for 4 hours. Calibrate AAS with matrix-matched standards (0.1–10 ppm Cu). Calculate uncertainty via propagation of error:
\text{% Uncertainty} = \sqrt{\left(\frac{\sigma_{\text{weight}}}{m}\right)^2 + \left(\frac{\sigma_{\text{AAS}}}{C}\right)^2} \times 100
Eigenschaften
IUPAC Name |
copper;2,3,7,8,12,13,17,18-octaethylporphyrin-21,22-diide | |
---|---|---|
Details | Computed by Lexichem TK 2.7.0 (PubChem release 2021.05.07) | |
Source | PubChem | |
URL | https://pubchem.ncbi.nlm.nih.gov | |
Description | Data deposited in or computed by PubChem | |
InChI |
InChI=1S/C36H44N4.Cu/c1-9-21-22(10-2)30-18-32-25(13-5)26(14-6)34(39-32)20-36-28(16-8)27(15-7)35(40-36)19-33-24(12-4)23(11-3)31(38-33)17-29(21)37-30;/h17-20H,9-16H2,1-8H3;/q-2;+2 | |
Details | Computed by InChI 1.0.6 (PubChem release 2021.05.07) | |
Source | PubChem | |
URL | https://pubchem.ncbi.nlm.nih.gov | |
Description | Data deposited in or computed by PubChem | |
InChI Key |
WYWUPEFEFRBNGN-UHFFFAOYSA-N | |
Details | Computed by InChI 1.0.6 (PubChem release 2021.05.07) | |
Source | PubChem | |
URL | https://pubchem.ncbi.nlm.nih.gov | |
Description | Data deposited in or computed by PubChem | |
Canonical SMILES |
CCC1=C(C2=CC3=NC(=CC4=NC(=CC5=C(C(=C([N-]5)C=C1[N-]2)CC)CC)C(=C4CC)CC)C(=C3CC)CC)CC.[Cu+2] | |
Details | Computed by OEChem 2.3.0 (PubChem release 2021.05.07) | |
Source | PubChem | |
URL | https://pubchem.ncbi.nlm.nih.gov | |
Description | Data deposited in or computed by PubChem | |
Molecular Formula |
C36H44CuN4 | |
Details | Computed by PubChem 2.1 (PubChem release 2021.05.07) | |
Source | PubChem | |
URL | https://pubchem.ncbi.nlm.nih.gov | |
Description | Data deposited in or computed by PubChem | |
Molecular Weight |
596.3 g/mol | |
Details | Computed by PubChem 2.1 (PubChem release 2021.05.07) | |
Source | PubChem | |
URL | https://pubchem.ncbi.nlm.nih.gov | |
Description | Data deposited in or computed by PubChem | |
CAS No. |
14409-63-3 | |
Record name | ((2,3,7,8,12,13,17,18)-Octaethylporphinato)copper (II) | |
Source | ChemIDplus | |
URL | https://pubchem.ncbi.nlm.nih.gov/substance/?source=chemidplus&sourceid=0014409633 | |
Description | ChemIDplus is a free, web search system that provides access to the structure and nomenclature authority files used for the identification of chemical substances cited in National Library of Medicine (NLM) databases, including the TOXNET system. | |
Retrosynthesis Analysis
AI-Powered Synthesis Planning: Our tool employs the Template_relevance Pistachio, Template_relevance Bkms_metabolic, Template_relevance Pistachio_ringbreaker, Template_relevance Reaxys, Template_relevance Reaxys_biocatalysis model, leveraging a vast database of chemical reactions to predict feasible synthetic routes.
One-Step Synthesis Focus: Specifically designed for one-step synthesis, it provides concise and direct routes for your target compounds, streamlining the synthesis process.
Accurate Predictions: Utilizing the extensive PISTACHIO, BKMS_METABOLIC, PISTACHIO_RINGBREAKER, REAXYS, REAXYS_BIOCATALYSIS database, our tool offers high-accuracy predictions, reflecting the latest in chemical research and data.
Strategy Settings
Precursor scoring | Relevance Heuristic |
---|---|
Min. plausibility | 0.01 |
Model | Template_relevance |
Template Set | Pistachio/Bkms_metabolic/Pistachio_ringbreaker/Reaxys/Reaxys_biocatalysis |
Top-N result to add to graph | 6 |
Feasible Synthetic Routes
Haftungsausschluss und Informationen zu In-Vitro-Forschungsprodukten
Bitte beachten Sie, dass alle Artikel und Produktinformationen, die auf BenchChem präsentiert werden, ausschließlich zu Informationszwecken bestimmt sind. Die auf BenchChem zum Kauf angebotenen Produkte sind speziell für In-vitro-Studien konzipiert, die außerhalb lebender Organismen durchgeführt werden. In-vitro-Studien, abgeleitet von dem lateinischen Begriff "in Glas", beinhalten Experimente, die in kontrollierten Laborumgebungen unter Verwendung von Zellen oder Geweben durchgeführt werden. Es ist wichtig zu beachten, dass diese Produkte nicht als Arzneimittel oder Medikamente eingestuft sind und keine Zulassung der FDA für die Vorbeugung, Behandlung oder Heilung von medizinischen Zuständen, Beschwerden oder Krankheiten erhalten haben. Wir müssen betonen, dass jede Form der körperlichen Einführung dieser Produkte in Menschen oder Tiere gesetzlich strikt untersagt ist. Es ist unerlässlich, sich an diese Richtlinien zu halten, um die Einhaltung rechtlicher und ethischer Standards in Forschung und Experiment zu gewährleisten.