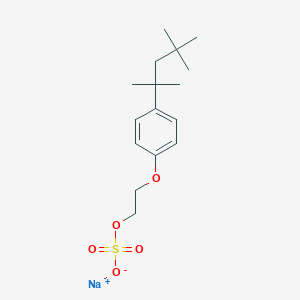
Triton X-301
Übersicht
Beschreibung
Triton X-301 is a non-ionic surfactant widely used in various scientific and industrial applications. It is known for its ability to reduce surface tension and enhance the solubility of hydrophobic compounds in aqueous solutions. This compound is a member of the Triton family of surfactants, which are characterized by their polyethylene glycol (PEG) head groups and hydrophobic alkyl chains .
Wissenschaftliche Forschungsanwendungen
Triton X-301 has a wide range of applications in scientific research, including:
Chemistry: Used as a solubilizing agent for hydrophobic compounds and as a stabilizer in various chemical reactions.
Biology: Employed in cell lysis protocols to extract cellular components and in membrane protein studies to solubilize membrane proteins.
Medicine: Utilized in drug formulation to enhance the solubility and bioavailability of poorly soluble drugs.
Wirkmechanismus
Safety and Hazards
Vorbereitungsmethoden
Synthetic Routes and Reaction Conditions: Triton X-301 is synthesized through the ethoxylation of octylphenol. The process involves the reaction of octylphenol with ethylene oxide under controlled conditions to produce a series of ethoxylated products. The degree of ethoxylation determines the specific properties of the resulting surfactant .
Industrial Production Methods: In industrial settings, the production of this compound involves the use of specialized reactors where octylphenol and ethylene oxide are combined in the presence of a catalyst. The reaction is carried out at elevated temperatures and pressures to ensure efficient ethoxylation. The resulting product is then purified and formulated into a 20% aqueous dispersion for commercial use .
Analyse Chemischer Reaktionen
Types of Reactions: Triton X-301 primarily undergoes reactions typical of non-ionic surfactants, including:
Oxidation: this compound can be oxidized under specific conditions, leading to the formation of various oxidation products.
Reduction: The compound can also undergo reduction reactions, although these are less common.
Substitution: this compound can participate in substitution reactions, particularly in the presence of strong nucleophiles.
Common Reagents and Conditions:
Oxidation: Common oxidizing agents include hydrogen peroxide and potassium permanganate.
Reduction: Reducing agents such as sodium borohydride can be used.
Substitution: Strong nucleophiles like sodium hydroxide are often employed.
Major Products Formed: The major products formed from these reactions depend on the specific conditions and reagents used. For example, oxidation may yield various hydroxylated derivatives, while substitution reactions can produce a range of substituted ethoxylates .
Vergleich Mit ähnlichen Verbindungen
Triton X-100: Another non-ionic surfactant with similar properties but different ethoxylation degree.
Triton X-114: Known for its phase separation properties at different temperatures.
Nonidet P-40 (NP-40): A non-ionic surfactant used in similar applications but with a different hydrophobic chain structure.
Igepal CA-630: Similar to Triton X-100 but with a different brand name
Uniqueness: Triton X-301 is unique due to its specific ethoxylation degree, which provides distinct solubilizing and stabilizing properties. It is particularly effective in applications requiring a balance between hydrophilicity and hydrophobicity .
Eigenschaften
IUPAC Name |
sodium;2-[4-(2,4,4-trimethylpentan-2-yl)phenoxy]ethyl sulfate | |
---|---|---|
Source | PubChem | |
URL | https://pubchem.ncbi.nlm.nih.gov | |
Description | Data deposited in or computed by PubChem | |
InChI |
InChI=1S/C16H26O5S.Na/c1-15(2,3)12-16(4,5)13-6-8-14(9-7-13)20-10-11-21-22(17,18)19;/h6-9H,10-12H2,1-5H3,(H,17,18,19);/q;+1/p-1 | |
Source | PubChem | |
URL | https://pubchem.ncbi.nlm.nih.gov | |
Description | Data deposited in or computed by PubChem | |
InChI Key |
PQSPZTWMLBELMD-UHFFFAOYSA-M | |
Source | PubChem | |
URL | https://pubchem.ncbi.nlm.nih.gov | |
Description | Data deposited in or computed by PubChem | |
Canonical SMILES |
CC(C)(C)CC(C)(C)C1=CC=C(C=C1)OCCOS(=O)(=O)[O-].[Na+] | |
Source | PubChem | |
URL | https://pubchem.ncbi.nlm.nih.gov | |
Description | Data deposited in or computed by PubChem | |
Molecular Formula |
C16H25NaO5S | |
Source | PubChem | |
URL | https://pubchem.ncbi.nlm.nih.gov | |
Description | Data deposited in or computed by PubChem | |
DSSTOX Substance ID |
DTXSID10155131 | |
Record name | Triton X-301 | |
Source | EPA DSSTox | |
URL | https://comptox.epa.gov/dashboard/DTXSID10155131 | |
Description | DSSTox provides a high quality public chemistry resource for supporting improved predictive toxicology. | |
Molecular Weight |
352.4 g/mol | |
Source | PubChem | |
URL | https://pubchem.ncbi.nlm.nih.gov | |
Description | Data deposited in or computed by PubChem | |
CAS No. |
12627-38-2 | |
Record name | Triton X-301 | |
Source | EPA DSSTox | |
URL | https://comptox.epa.gov/dashboard/DTXSID10155131 | |
Description | DSSTox provides a high quality public chemistry resource for supporting improved predictive toxicology. | |
Retrosynthesis Analysis
AI-Powered Synthesis Planning: Our tool employs the Template_relevance Pistachio, Template_relevance Bkms_metabolic, Template_relevance Pistachio_ringbreaker, Template_relevance Reaxys, Template_relevance Reaxys_biocatalysis model, leveraging a vast database of chemical reactions to predict feasible synthetic routes.
One-Step Synthesis Focus: Specifically designed for one-step synthesis, it provides concise and direct routes for your target compounds, streamlining the synthesis process.
Accurate Predictions: Utilizing the extensive PISTACHIO, BKMS_METABOLIC, PISTACHIO_RINGBREAKER, REAXYS, REAXYS_BIOCATALYSIS database, our tool offers high-accuracy predictions, reflecting the latest in chemical research and data.
Strategy Settings
Precursor scoring | Relevance Heuristic |
---|---|
Min. plausibility | 0.01 |
Model | Template_relevance |
Template Set | Pistachio/Bkms_metabolic/Pistachio_ringbreaker/Reaxys/Reaxys_biocatalysis |
Top-N result to add to graph | 6 |
Feasible Synthetic Routes
Haftungsausschluss und Informationen zu In-Vitro-Forschungsprodukten
Bitte beachten Sie, dass alle Artikel und Produktinformationen, die auf BenchChem präsentiert werden, ausschließlich zu Informationszwecken bestimmt sind. Die auf BenchChem zum Kauf angebotenen Produkte sind speziell für In-vitro-Studien konzipiert, die außerhalb lebender Organismen durchgeführt werden. In-vitro-Studien, abgeleitet von dem lateinischen Begriff "in Glas", beinhalten Experimente, die in kontrollierten Laborumgebungen unter Verwendung von Zellen oder Geweben durchgeführt werden. Es ist wichtig zu beachten, dass diese Produkte nicht als Arzneimittel oder Medikamente eingestuft sind und keine Zulassung der FDA für die Vorbeugung, Behandlung oder Heilung von medizinischen Zuständen, Beschwerden oder Krankheiten erhalten haben. Wir müssen betonen, dass jede Form der körperlichen Einführung dieser Produkte in Menschen oder Tiere gesetzlich strikt untersagt ist. Es ist unerlässlich, sich an diese Richtlinien zu halten, um die Einhaltung rechtlicher und ethischer Standards in Forschung und Experiment zu gewährleisten.