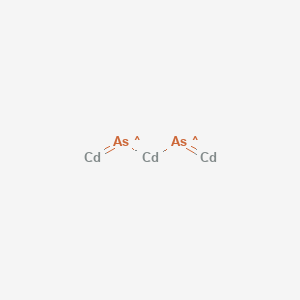
Cadmium arsenide
- Klicken Sie auf QUICK INQUIRY, um ein Angebot von unserem Expertenteam zu erhalten.
- Mit qualitativ hochwertigen Produkten zu einem WETTBEWERBSFÄHIGEN Preis können Sie sich mehr auf Ihre Forschung konzentrieren.
Übersicht
Beschreibung
Cadmium arsenide is an inorganic compound with the chemical formula Cd₃As₂ It is a member of the II-V family of semimetals and is known for its unique electronic propertiesIt is characterized by its dark grey appearance and solid state at room temperature .
Wirkmechanismus
Target of Action
Cadmium arsenide (Cd3As2) is an inorganic semimetal in the II-V family . It has been rediscovered as a 3D topological semimetal, positioned as a bulk analogue of graphene . The primary targets of this compound are the conduction band and valence band, which have a linear dispersion law and touch each other in the 3D Brillouin zone to form Dirac points .
Mode of Action
This compound interacts with its targets by forming Dirac points in the 3D Brillouin zone . Provided the time reversal and inverted symmetry, the Dirac points are doubly degenerate . Symmetry breaking leads to splitting of a Dirac point . A magnetic field transforms the Weyl semimetal, generating negative magnetoresistance (NMR) and giving rise to superconducting properties .
Biochemical Pathways
It is known that cadmium can induce a decrease in mitochondrial membrane potential, primarily resulting from disruptions in the electron transport chain (etc), which reduce the efficiency of proton pumps and consequently fail to maintain the essential proton gradient across the mitochondrial membrane .
Pharmacokinetics
It is known that this compound decomposes in water , which may affect its bioavailability.
Result of Action
The molecular and cellular effects of this compound’s action are complex. It is known to exhibit the Nernst effect . It also shows degenerate n-type semiconductor intrinsic conductivity with a large mobility, low effective mass, and highly non-parabolic conduction band . It displays an inverted band structure, and the optical energy gap is less than 0 .
Action Environment
Environmental factors can influence the action, efficacy, and stability of this compound. For instance, the transition from cubic β-Cd3As2 to tetragonal α-Cd3As2 is accompanied by a significant change in volume, so single crystals are destroyed . Also, soil pH is a critical factor, as acidic conditions can enhance cadmium mobility and increase its leaching potential .
Biochemische Analyse
Biochemical Properties
Cadmium arsenide plays a role in various biochemical reactions, particularly those involving metal ions. It interacts with several enzymes and proteins, often disrupting their normal functions. For instance, cadmium ions from this compound can bind to thiol groups in proteins, leading to the inhibition of enzymes such as glutathione peroxidase and superoxide dismutase. These interactions can result in oxidative stress and cellular damage .
Cellular Effects
This compound has profound effects on various cell types and cellular processes. It can induce oxidative stress by generating reactive oxygen species (ROS), which in turn affects cell signaling pathways, gene expression, and cellular metabolism. For example, exposure to this compound can activate the mitogen-activated protein kinase (MAPK) pathway, leading to altered gene expression and apoptosis . Additionally, this compound can disrupt calcium homeostasis, further impacting cellular functions .
Molecular Mechanism
At the molecular level, this compound exerts its effects through several mechanisms. It can bind to DNA and proteins, causing structural changes and functional disruptions. Cadmium ions from this compound can replace essential metal ions in enzymes, leading to enzyme inhibition or activation. For instance, cadmium can inhibit DNA repair enzymes, resulting in increased mutagenesis and carcinogenesis . Furthermore, this compound can modulate gene expression by interacting with transcription factors and signaling molecules .
Temporal Effects in Laboratory Settings
In laboratory settings, the effects of this compound can change over time. This compound is relatively stable, but its degradation products can also be toxic. Long-term exposure to this compound in vitro or in vivo can lead to chronic oxidative stress, DNA damage, and impaired cellular functions . Studies have shown that this compound can accumulate in tissues over time, leading to prolonged toxic effects .
Dosage Effects in Animal Models
The effects of this compound vary with different dosages in animal models. Low doses may cause mild oxidative stress and metabolic changes, while high doses can lead to severe toxicity, including liver and kidney damage . Threshold effects have been observed, where certain doses result in significant biochemical and physiological changes. High doses of this compound can also induce apoptosis and necrosis in various tissues .
Metabolic Pathways
This compound is involved in several metabolic pathways, primarily those related to metal ion homeostasis and oxidative stress. It can interact with enzymes such as glutathione reductase and catalase, affecting their activities and leading to altered metabolic flux . This compound can also influence the levels of metabolites such as glutathione and reactive oxygen species, further impacting cellular metabolism .
Transport and Distribution
Within cells and tissues, this compound is transported and distributed through various mechanisms. It can interact with metal transporters and binding proteins, facilitating its uptake and accumulation. For example, cadmium ions from this compound can be transported by divalent metal transporter 1 (DMT1) and metallothioneins, leading to its localization in specific cellular compartments . The distribution of this compound within tissues can also affect its toxicity and biological effects .
Subcellular Localization
This compound exhibits specific subcellular localization, which can influence its activity and function. It is often found in the cytosol, where it can interact with cytoplasmic proteins and enzymes. Additionally, this compound can localize to the nucleus, where it can bind to DNA and affect gene expression . The subcellular distribution of this compound is influenced by factors such as post-translational modifications and targeting signals .
Vorbereitungsmethoden
Synthetic Routes and Reaction Conditions: Cadmium arsenide can be synthesized through several methods. One common approach involves the direct reaction of cadmium and arsenic at high temperatures. The reaction is typically carried out in a sealed tube to prevent the loss of volatile arsenic. The reaction can be represented as:
3Cd+2As→Cd3As2
Industrial Production Methods: In industrial settings, this compound is often produced using thermal evaporation techniques. This involves the evaporation of pre-synthesized this compound batches, followed by condensation to form single crystals or thin films. The process is enhanced by the congruent dissociation of this compound, which ensures the formation of high-purity crystals .
Analyse Chemischer Reaktionen
Types of Reactions: Cadmium arsenide undergoes several types of chemical reactions, including:
Oxidation: this compound can be oxidized to form cadmium oxide and arsenic trioxide.
Reduction: It can be reduced back to its elemental forms under specific conditions.
Substitution: this compound can participate in substitution reactions where arsenic atoms are replaced by other elements.
Common Reagents and Conditions:
Oxidation: Oxygen or air at elevated temperatures.
Reduction: Hydrogen gas or other reducing agents.
Substitution: Various halogens or other reactive elements.
Major Products Formed:
Oxidation: Cadmium oxide (CdO) and arsenic trioxide (As₂O₃).
Reduction: Elemental cadmium and arsenic.
Substitution: Compounds where arsenic is replaced by other elements.
Wissenschaftliche Forschungsanwendungen
Cadmium arsenide has a wide range of scientific research applications:
Electronics: Due to its unique electronic properties, this compound is used in the development of high-speed electronic devices and sensors.
Materials Science: It is studied for its potential use in topological insulators and other advanced materials.
Thermoelectric Devices: this compound’s ability to conduct electricity rapidly makes it a candidate for thermoelectric applications.
Superconductivity: Research has shown that this compound exhibits superconducting properties under certain conditions.
Vergleich Mit ähnlichen Verbindungen
Cadmium arsenide can be compared with other similar compounds in the II-V family, such as:
- Zinc phosphide (Zn₃P₂)
- Zinc arsenide (Zn₃As₂)
- Cadmium phosphide (Cd₃P₂)
Uniqueness: this compound stands out due to its unique electronic properties, including its high electron mobility and the presence of Dirac points. These properties make it a promising material for advanced electronic and thermoelectric applications .
Eigenschaften
CAS-Nummer |
12006-15-4 |
---|---|
Molekularformel |
AsCd |
Molekulargewicht |
187.34 g/mol |
IUPAC-Name |
arsenic;cadmium |
InChI |
InChI=1S/As.Cd |
InChI-Schlüssel |
APAWRDGVSNYWSL-UHFFFAOYSA-N |
SMILES |
[As]=[Cd].[As]=[Cd].[Cd] |
Kanonische SMILES |
[As].[Cd] |
Herkunft des Produkts |
United States |
Q1: What is the chemical formula and molecular weight of cadmium arsenide?
A1: this compound has the chemical formula Cd3As2 and a molecular weight of 483.06 g/mol.
Q2: What are some key spectroscopic characteristics of this compound?
A2: Raman spectroscopy reveals characteristic peaks for Cd3As2 films at 194, 249, and 303 cm−1. [] These peaks can be used to identify and characterize the material.
Q3: What makes this compound unique in terms of its electronic properties?
A3: Cd3As2 is classified as a three-dimensional Dirac semimetal, meaning its electrons behave as massless Dirac fermions near the Fermi level. [, ] This leads to exceptional electronic properties, including:
- Ultrahigh electron mobility: Cd3As2 exhibits exceptionally high electron mobilities, exceeding those of many traditional semiconductors. [, , , ] Values as high as 41,000 cm2V−1s−1 have been reported in high-quality (001)-oriented films at low temperatures. [] This is attributed to the unique electronic band structure and the suppression of backscattering for electrons near the Dirac points. []
- Large magnetoresistance: Cd3As2 displays large magnetoresistance, meaning its electrical resistance changes significantly under an applied magnetic field. [, , ] This property makes it suitable for applications in magnetic sensors.
- Topological properties: The surface states of Cd3As2 can exhibit topological insulator properties. [, , , ] This opens up possibilities for exploring exotic quantum phenomena and developing novel spintronic devices.
Q4: What are the potential applications of this compound in electronics?
A4: Due to its unique electronic properties, Cd3As2 holds promise for various electronic applications:
- High-speed transistors: The high electron mobility of Cd3As2 makes it a promising candidate for next-generation, high-speed transistors operating in the terahertz (THz) regime. [] Such transistors could revolutionize communication technologies and enable faster data processing.
- Thermal detectors: The large Nernst effect observed in Cd3As2, coupled with its high electron mobility and low thermal conductivity, makes it attractive for developing efficient thermal detectors. []
- Photodetectors: Cd3As2 has shown potential for use in photodetectors, particularly in the infrared (IR) range. [] Its narrow bandgap and high sensitivity to light make it suitable for detecting low-energy photons.
- Spintronic devices: The topological surface states of Cd3As2 could be harnessed to create spintronic devices, which utilize the spin of electrons for information processing and storage. [, ]
Q5: How does strain engineering influence the properties of this compound thin films?
A5: Applying strain to Cd3As2 thin films can significantly alter their electronic properties by modifying their crystal structure and symmetry. [] For instance, biaxial compressive strain can induce a transition to an orthorhombic phase, while tensile strain leads to a non-centrosymmetric structure. These structural changes can be used to tune the electronic band structure and potentially induce new topological phases. []
Q6: Can you explain the significance of the "Fermi arcs" observed in this compound?
A6: "Fermi arcs" are unusual surface states that connect the Dirac points in the bulk of Cd3As2. [] These arcs are a characteristic signature of Weyl semimetals, a class of materials closely related to Dirac semimetals. The presence of Fermi arcs has important implications for the material's transport properties, contributing to its high conductivity and unique response to magnetic fields.
Q7: How does the surface of this compound interact with its environment, and what are the implications?
A7: The surface of Cd3As2 can undergo reconstruction, where the arrangement of surface atoms deviates from the bulk structure to minimize energy. [] One study revealed that the outermost Cd atoms on a (112) surface descend into the As sublayer, leading to a self-passivated surface with As atoms forming a triangle lattice. [] This reconstruction influences the surface electronic structure and its interaction with adsorbates. For example, oxidation of the reconstructed surface primarily involves the formation of As-O-Cd bonds, yet the overall band structure remains relatively robust against oxidation. []
Q8: What methods are typically used to synthesize this compound thin films?
A8: Several methods are employed for synthesizing Cd3As2 thin films, each offering advantages and challenges:
- Molecular beam epitaxy (MBE): MBE allows for precise control over film thickness, composition, and doping, enabling the growth of high-quality single-crystal films. [, , ] This method is crucial for studying the intrinsic properties of Cd3As2 and exploring its potential in high-performance electronic devices.
- Thermal evaporation: This method involves evaporating Cd3As2 from a source and depositing it onto a substrate. [] While simpler than MBE, it offers less control over film properties and may result in films with higher defect densities.
Q9: How do the properties of this compound films vary with film thickness?
A9: The properties of Cd3As2 films can change significantly with thickness, particularly for very thin films, where quantum confinement effects become important:
- Electronic band structure: As film thickness decreases, the bulk Dirac points can become gapped, leading to a transition from a Dirac semimetal to a topological insulator. [, ] This transition has been observed in (001)-oriented films with thicknesses around 50 nm. []
- Magnetoresistance: The magnitude and anisotropy of magnetoresistance can change with film thickness, providing insights into the interplay between surface and bulk transport mechanisms. []
Q10: What are some challenges in characterizing and manipulating this compound at the nanoscale?
A10: Characterizing and manipulating Cd3As2 at the nanoscale presents challenges due to its complex surface chemistry and the sensitivity of its electronic properties to defects and environmental factors.
- Surface oxidation: Cd3As2 is prone to surface oxidation, which can alter its electronic properties. [] Careful surface passivation or encapsulation techniques are required to protect the material from degradation.
- Defect control: Defects in the crystal structure, such as vacancies and grain boundaries, can significantly impact the electronic transport properties of Cd3As2. [] Controlling defect density during synthesis and processing is crucial for achieving high-performance devices.
Q11: How have computational methods contributed to our understanding of this compound?
A11: Computational chemistry and modeling have played a crucial role in understanding the electronic structure, predicting new phases, and interpreting experimental observations in Cd3As2:
- Density functional theory (DFT): DFT calculations have been extensively used to study the electronic band structure of Cd3As2, confirming its Dirac semimetal nature and predicting the emergence of topological surface states. [, , ] DFT has also been crucial for understanding the impact of strain and doping on the electronic properties.
- Tight-binding models: Tight-binding models, parameterized by DFT calculations, have been developed to describe the low-energy electronic structure of Cd3As2. [] These models provide a simpler framework for studying transport phenomena and exploring the effects of external fields and disorder.
- First-principles calculations: First-principles calculations, based on fundamental physical laws, have been employed to predict the stability of different crystal structures and their response to pressure, providing insights into the phase transitions observed experimentally. [, ]
Q12: What are the safety concerns associated with this compound?
A12: this compound contains cadmium and arsenic, both of which are toxic elements. [] Handling Cd3As2 requires appropriate safety precautions to minimize exposure risks:
Q13: What are the environmental concerns related to this compound?
A13: The presence of cadmium and arsenic in Cd3As2 raises environmental concerns, particularly regarding its disposal and potential leaching into the environment. []
- Water contamination: Cadmium and arsenic are known water pollutants, and their release into water bodies can harm aquatic life and potentially contaminate drinking water sources. [] Proper waste management and disposal are crucial to preventing environmental contamination.
Q14: What are the major challenges and opportunities for future research on this compound?
A14: Despite the significant progress made in understanding and utilizing Cd3As2, several challenges and opportunities remain for future research:
- Understanding the role of electron-phonon interactions: The interaction between electrons and lattice vibrations (phonons) plays a crucial role in determining the transport properties of Cd3As2. Further investigations into electron-phonon coupling are essential for understanding the factors limiting electron mobility and for developing strategies to enhance it. [, ]
- Investigating the potential for topological superconductivity: Theoretical predictions suggest that Cd3As2 could host topological superconductivity, an exotic state of matter that could revolutionize quantum computing. [] Experimental efforts to induce and study topological superconductivity in Cd3As2 are ongoing and hold great promise for future breakthroughs.
Haftungsausschluss und Informationen zu In-Vitro-Forschungsprodukten
Bitte beachten Sie, dass alle Artikel und Produktinformationen, die auf BenchChem präsentiert werden, ausschließlich zu Informationszwecken bestimmt sind. Die auf BenchChem zum Kauf angebotenen Produkte sind speziell für In-vitro-Studien konzipiert, die außerhalb lebender Organismen durchgeführt werden. In-vitro-Studien, abgeleitet von dem lateinischen Begriff "in Glas", beinhalten Experimente, die in kontrollierten Laborumgebungen unter Verwendung von Zellen oder Geweben durchgeführt werden. Es ist wichtig zu beachten, dass diese Produkte nicht als Arzneimittel oder Medikamente eingestuft sind und keine Zulassung der FDA für die Vorbeugung, Behandlung oder Heilung von medizinischen Zuständen, Beschwerden oder Krankheiten erhalten haben. Wir müssen betonen, dass jede Form der körperlichen Einführung dieser Produkte in Menschen oder Tiere gesetzlich strikt untersagt ist. Es ist unerlässlich, sich an diese Richtlinien zu halten, um die Einhaltung rechtlicher und ethischer Standards in Forschung und Experiment zu gewährleisten.