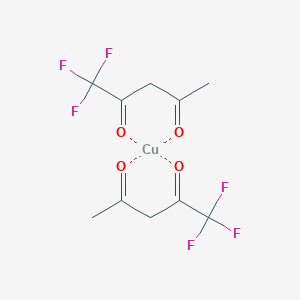
Copper;1,1,1-trifluoropentane-2,4-dione
- Klicken Sie auf QUICK INQUIRY, um ein Angebot von unserem Expertenteam zu erhalten.
- Mit qualitativ hochwertigen Produkten zu einem WETTBEWERBSFÄHIGEN Preis können Sie sich mehr auf Ihre Forschung konzentrieren.
Übersicht
Beschreibung
Copper(II) trifluoroacetylacetonate (Cu(tfacac)₂, CAS 14324-82-4) is a coordination complex where copper(II) is chelated by two 1,1,1-trifluoropentane-2,4-dione (TFAA) ligands. TFAA, a fluorinated β-diketone (IUPAC: 1,1,1-trifluoropentane-2,4-dione, C₅H₅F₃O₂), exhibits unique electronic and steric properties due to the electron-withdrawing trifluoromethyl group. This ligand enhances the Lewis acidity of the metal center and stabilizes the complex through strong intramolecular hydrogen bonds in its enol tautomer . The compound is widely used in catalysis, materials science (e.g., single-molecule magnets ), and dye-sensitized solar cells (DSCs) .
Vorbereitungsmethoden
Conventional Solution-Phase Synthesis
The most widely documented method for preparing Copper;1,1,1-trifluoropentane-2,4-dione involves a ligand-exchange reaction between copper(II) acetate and TFAA in a polar aprotic solvent. This approach aligns with general practices for synthesizing metal β-diketonates .
Reaction Mechanism and Stoichiometry
The synthesis proceeds via deprotonation of TFAA by a base, followed by coordination of the resulting enolate to the copper center. A 1:2 molar ratio of copper(II) acetate to TFAA is typically employed to ensure complete complexation . The overall reaction can be represented as:
2 + 2 \, \text{TFAA} \rightarrow \text{Cu(TFAA)}2 + 2 \, \text{HOAc}Cu(OAc)2+2TFAA→Cu(TFAA)2+2HOAc
where acetic acid (HOAc) is liberated as a byproduct .
Procedure and Conditions
-
Dissolution of Reactants : Copper(II) acetate (1 equiv) and TFAA (2 equiv) are dissolved in anhydrous ethanol under inert atmosphere conditions .
-
Reflux and Stirring : The mixture is refluxed at 78°C for 6–8 hours with vigorous stirring to facilitate ligand exchange .
-
Isolation : The product precipitates upon cooling and is collected via vacuum filtration. Alternatively, solvent removal under reduced pressure yields the complex as a crystalline solid .
-
Purification : Recrystallization from dichloromethane/hexane mixtures enhances purity .
Table 1: Optimization Parameters for Conventional Synthesis
Alternative Synthetic Routes
While the conventional method remains predominant, exploratory studies have investigated variations in copper precursors and solvents:
Use of Copper(II) Nitrate
Substituting copper(II) nitrate for acetate in tetrahydrofuran (THF) has been attempted, but yields are marginally lower (~75% vs. 85–90% for acetate) . This is attributed to nitrate’s weaker basicity, which slows deprotonation of TFAA .
Solvent Effects
Non-polar solvents like toluene require extended reaction times (12–14 hours) and higher temperatures (110°C), yet achieve comparable yields . Polar solvents such as acetonitrile reduce reaction times but may co-coordinate with copper, necessitating post-synthesis ligand displacement steps .
Characterization and Validation
Successful synthesis is confirmed through spectroscopic and analytical techniques:
-
IR Spectroscopy : Strong absorption bands at 1600–1650 cm⁻¹ (C=O stretch) and 1250–1300 cm⁻¹ (C-F stretch) validate ligand coordination .
-
Elemental Analysis : Matches theoretical values for C (32.35%), H (2.71%), and F (30.68%) .
-
X-ray Crystallography : Confirms octahedral geometry around the copper center, with two bidentate TFAA ligands and two solvent molecules occupying axial positions .
Challenges and Mitigation Strategies
Hygroscopicity
The compound’s hygroscopic nature complicates handling. Storage under desiccating conditions (e.g., P₂O₅) is critical to prevent hydrolysis .
Ligand Purity
Impurities in TFAA (e.g., unreacted 1,1,1-trifluoro-2,4-pentanedione) reduce complex yields. Pre-synthesis distillation of TFAA at 150–160°C under reduced pressure is recommended .
Analyse Chemischer Reaktionen
Types of Reactions
Copper;1,1,1-trifluoropentane-2,4-dione undergoes various chemical reactions, including:
Coordination Reactions: Forms complexes with other metal ions such as nickel(II), cobalt(II), and iron(III).
Oxidation and Reduction: Can participate in redox reactions, although specific conditions and reagents vary.
Substitution Reactions: The trifluoromethyl group can be substituted under specific conditions.
Common Reagents and Conditions
Coordination Reactions: Typically involve aqueous solutions at 25°C and 0.5 mol dm⁻³ ionic strength.
Oxidation and Reduction: Common reagents include oxidizing agents like hydrogen peroxide and reducing agents like sodium borohydride.
Substitution Reactions: Often require catalysts and specific solvents to facilitate the reaction.
Major Products
The major products of these reactions depend on the specific conditions and reagents used. For example, coordination reactions with nickel(II) yield nickel complexes, while oxidation reactions may produce copper(II) derivatives.
Wissenschaftliche Forschungsanwendungen
Copper;1,1,1-trifluoropentane-2,4-dione has a wide range of applications in scientific research:
Biology: Investigated for its potential use in biological assays and as a probe for studying enzyme activities.
Medicine: Explored for its potential therapeutic properties, particularly in the development of metal-based drugs.
Wirkmechanismus
The mechanism of action of Copper;1,1,1-trifluoropentane-2,4-dione involves its ability to form stable complexes with metal ions. The compound reacts primarily with the enol tautomer of the β-diketone, while the keto-tautomer remains inert . This selective reactivity allows it to act as a chelating agent, facilitating various chemical transformations.
Vergleich Mit ähnlichen Verbindungen
Comparative Analysis with Similar Compounds
Structural and Electronic Properties
Hydrogen Bonding and Tautomerism
- TFAA vs. Non-Fluorinated β-Diketones: Unlike pentane-2,4-dione (acetylacetone, Hacac), TFAA exhibits significant hydrogen bond acidity due to the electron-withdrawing CF₃ group, which polarizes the enolic O–H bond. This results in stronger H-bonding interactions and a higher propensity to exist in the enol form (>90% enol tautomer in pure liquid) . In contrast, acetylacetone exists in a keto-enol equilibrium with ~80% enol form in nonpolar solvents.
- TFAA vs. Chlorinated Analogues : 1-Chloro-1,1-difluoropentane-2,4-dione (CDFAA) shows reduced symmetry and altered dipole moments compared to TFAA, as revealed by DFT calculations. The Cl substituent in CDFAA increases molecular polarity but weakens H-bond acidity relative to TFAA .
Coordination Geometry
- Cu(tfacac)₂ vs. Cu(acac)₂: The trifluoromethyl group in TFAA induces greater distortion in the copper coordination sphere compared to acetylacetonate (acac) ligands.
Physicochemical Properties
Partition Coefficients (log P)
TFAA derivatives exhibit distinct partitioning behavior due to fluorination:
Compound | log P (Water → Octanol) | Hydrogen Bond Acidity (A) |
---|---|---|
1,1,1-Trifluoropentane-2,4-dione | 1.36 | 0.69 (significant) |
Pentane-2,4-dione | 0.91 | 0.00 (no H-bond acidity) |
1,1,1-Trifluorobenzoylacetone | 2.73 (in CHCl₃) | 0.71 |
TFAA’s higher log P and H-bond acidity enhance its lipophilicity and solvent compatibility relative to non-fluorinated β-diketones .
Optical Properties
- Refractive Index: TFAA’s refractive index (1.388) is lower than acetylacetone (1.449) due to fluorination reducing electron density. However, computational models (e.g., COSMO-RS) show deviations when stereochemical H-bonding in the enol form is neglected .
- Absorption Spectra : In Ru(II)-polypyridyl DSCs, TFAA-based dyes (e.g., [Ru(tctpy)(tfpd)(NCS)]) exhibit broad metal-to-ligand charge transfer (MLCT) bands extending to 950 nm, outperforming analogues with longer alkyl chains (e.g., tfdd, tfid) in near-IR absorption .
Condensation Reactions
- Cyclization with Hydrazines : TFAA reacts with hydrazinyl groups to form 5-hydroxy-3-methyl-5-(trifluoromethyl)-4,5-dihydropyrazoles in 76% yield, whereas hexafluoropentane-2,4-dione yields analogous products in 64% yield. The CF₃ group in TFAA stabilizes intermediates, enhancing reaction efficiency .
- Enaminone Formation: TFAA-derived enaminones show lower cyclization yields compared to non-fluorinated β-diketones due to steric and electronic effects .
Catalytic Activity
Cu(tfacac)₂ demonstrates superior catalytic activity in defluoroborylation reactions compared to Cu(acac)₂, attributed to the enhanced electrophilicity of the Cu center .
Single-Molecule Magnets (SMMs)
Dy(III) complexes with TFAA ligands (e.g., Dy(tfacac)₃(H₂O)₂) exhibit slow magnetic relaxation, a hallmark of SMM behavior. The CF₃ group improves ligand field anisotropy, increasing the energy barrier for spin reversal .
Dye-Sensitized Solar Cells
TFAA-based Ru(II) sensitizers achieve higher power conversion efficiencies (8–10%) than non-fluorinated analogues due to extended MLCT absorption and reduced electron recombination .
Biologische Aktivität
Copper;1,1,1-trifluoropentane-2,4-dione, commonly referred to as copper(II) bis(1,1,1-trifluoropentane-2,4-dionato), is a coordination compound formed by the complexation of copper ions with 1,1,1-trifluoropentane-2,4-dione. This compound has garnered interest for its potential biological activities, particularly in the fields of medicinal chemistry and catalysis. The presence of trifluoromethyl groups enhances its reactivity and solubility in organic solvents.
This compound can be synthesized through various methods, including the reaction of copper(II) acetate with 1,1,1-trifluoropentane-2,4-dione in organic solvents like ethanol. The resulting complex is characterized by its unique coordination chemistry and stability due to the electron-withdrawing properties of the trifluoromethyl groups.
Property | Value |
---|---|
Molecular Formula | C5H5F3O2 |
Molecular Weight | 154.09 g/mol |
CAS Number | 367-57-7 |
Solubility | Slightly soluble in water; soluble in many organic solvents |
The biological activity of this compound is primarily attributed to its ability to form stable complexes with various biomolecules and metal ions. The compound interacts preferentially with the enolic form of β-diketones, which can significantly influence the stability and reactivity of metal complexes formed with this diketone. This interaction is crucial for its potential applications in enzyme studies and as a probe for biological assays.
Biological Applications
Research has indicated that this compound exhibits various biological activities:
- Antibacterial and Antifungal Activity: Some studies suggest that copper(II) complexes may possess antibacterial or antifungal properties. The specific mechanisms are still under investigation but may involve disruption of microbial cell membranes or interference with enzymatic functions.
- Enzyme Studies: The compound has been utilized as a probe for studying enzyme activities due to its ability to interact with different enzymes and affect their catalytic efficiency .
Case Study 1: Enzyme Inhibition
In a study exploring the inhibition of certain enzymes by metal complexes, this compound was shown to significantly inhibit the activity of specific dehydrogenases. The inhibition was attributed to the formation of stable enzyme-metal complexes that altered the active site conformation .
Case Study 2: Synthesis of Pyrazoles
Research conducted by Singh et al. demonstrated that copper(II) bis(1,1,1-trifluoropentane-2,4-dionato) acted as an effective catalyst in the regioselective synthesis of pyrazoles under ultrasonic irradiation. The study highlighted the efficiency of this compound in promoting chemical reactions that could lead to biologically active heterocycles .
Comparative Analysis
To understand the uniqueness of this compound compared to similar compounds:
Table 2: Comparison with Similar Compounds
Compound | Key Features | Biological Activity |
---|---|---|
Acetylacetone (AA) | Lacks trifluoromethyl group | Limited antibacterial activity |
Hexafluoroacetylacetone (HFAA) | More electron-withdrawing | Enhanced reactivity but less stability |
Copper(II) Triflate | Used as a catalyst | Exhibits some antibacterial properties |
Q & A
Basic Research Questions
Q. What are the optimal synthetic conditions for Copper;1,1,1-trifluoropentane-2,4-dione complexes, and how do reaction parameters influence yield?
Methodological Answer: Synthesis typically involves ligand exchange reactions under inert atmospheres. Key parameters include solvent choice (e.g., anhydrous THF or acetonitrile), temperature control (60–80°C), and stoichiometric ratios of copper precursors to ligands. For reproducibility, use Schlenk-line techniques to exclude moisture/oxygen. Yield optimization can employ factorial design experiments to test variables like pH, reaction time, and ligand excess . Characterization via elemental analysis and IR spectroscopy validates purity.
Q. Which spectroscopic and crystallographic techniques are most effective for structural characterization of this copper complex?
Methodological Answer: X-ray diffraction (XRD) is critical for determining coordination geometry and bond lengths. Pair this with UV-Vis spectroscopy to confirm d-d transitions (400–800 nm range) and electron paramagnetic resonance (EPR) to identify Cu(II) oxidation states. NMR (¹H, ¹⁹F) in deuterated solvents like CDCl₃ resolves ligand environments, while mass spectrometry (ESI-MS) confirms molecular ion peaks .
Q. How does this complex behave as a catalyst in organic transformations, and what substrates are most reactive?
Methodological Answer: The complex acts as a Lewis acid catalyst in C–F bond activation or cross-coupling reactions. Test catalytic efficiency using styrenes or aryl halides as substrates in trifluoromethylation reactions. Monitor turnover frequency (TOF) via GC-MS or HPLC. Compare with control reactions lacking the catalyst to isolate its effect. Kinetic studies under varying temperatures (25–100°C) and pressures can elucidate mechanistic pathways .
Advanced Research Questions
Q. What electron-transfer mechanisms govern the catalytic activity of this complex in redox reactions?
Methodological Answer: Use cyclic voltammetry (CV) to identify redox potentials and square-wave voltammetry to detect intermediates. Density functional theory (DFT) calculations model ligand-to-metal charge transfer (LMCT) pathways. Pair experimental data with computational results to validate proposed mechanisms, such as single-electron transfer (SET) vs. inner-sphere electron transfer .
Q. How do ligand modifications (e.g., fluorination) impact the stability and reactivity of the copper center?
Methodological Answer: Synthesize analogs with varying fluorination (e.g., CF₃ vs. CH₃ substituents) and compare stability via thermogravimetric analysis (TGA). Reactivity differences can be quantified using kinetic isotope effects (KIE) in catalytic cycles. X-ray absorption spectroscopy (XAS) probes ligand field changes, while Mulliken population analysis in DFT highlights electronic redistribution .
Q. What strategies resolve contradictions in reported catalytic efficiencies across studies?
Methodological Answer: Conduct meta-analyses of published data to identify variables causing discrepancies (e.g., solvent polarity, counterion effects). Reproduce key studies under standardized conditions, using multivariate regression to isolate influential factors. Cross-validate with in-situ FTIR or Raman spectroscopy to detect transient species not accounted for in prior work .
Q. How can computational modeling (e.g., DFT, MD) predict novel applications of this complex in materials science?
Methodological Answer: Perform DFT simulations to screen for potential applications in conductive polymers or magnetic materials. Molecular dynamics (MD) models assess thermal stability in polymer matrices. Validate predictions by synthesizing target materials and testing conductivity (four-point probe) or magnetic susceptibility (SQUID magnetometry) .
Q. What interdisciplinary approaches integrate this complex into hybrid materials (e.g., MOFs, nanocomposites)?
Methodological Answer: Combine with organic linkers (e.g., terephthalic acid) to construct metal-organic frameworks (MOFs). Use solvothermal synthesis and characterize porosity via BET surface area analysis. For nanocomposites, employ atomic layer deposition (ALD) to coat copper complexes onto nanoparticles, then evaluate catalytic performance in flow reactors .
Q. Methodological Framework Design
Q. How to design experiments that align with theoretical frameworks for copper complex reactivity?
Methodological Answer: Ground studies in ligand field theory (LFT) or hard/soft acid-base (HSAB) principles. For example, design experiments testing ligand substitution rates against HSAB predictions. Use control groups with non-fluorinated ligands to isolate electronic effects. Theoretical alignment ensures interpretability and reduces ad-hoc explanations .
Q. What blended data-collection strategies enhance reproducibility in studies of this complex?
Methodological Answer: Combine quantitative data (e.g., kinetic rates, crystallographic data) with qualitative observations (e.g., color changes, precipitate formation). Use open-access databases to share raw spectra and crystallographic files (CIFs). Implement blind testing in collaborative studies to minimize bias .
Eigenschaften
CAS-Nummer |
14324-82-4 |
---|---|
Molekularformel |
C10H10CuF6O4 |
Molekulargewicht |
371.72 g/mol |
IUPAC-Name |
copper;1,1,1-trifluoro-4-hydroxypent-3-en-2-one |
InChI |
InChI=1S/2C5H5F3O2.Cu/c2*1-3(9)2-4(10)5(6,7)8;/h2*2,9H,1H3; |
InChI-Schlüssel |
DAWRQFGRHOWZRV-UHFFFAOYSA-N |
SMILES |
CC(=O)CC(=O)C(F)(F)F.CC(=O)CC(=O)C(F)(F)F.[Cu] |
Isomerische SMILES |
C/C(=C/C(=O)C(F)(F)F)/O.C/C(=C/C(=O)C(F)(F)F)/O.[Cu] |
Kanonische SMILES |
CC(=CC(=O)C(F)(F)F)O.CC(=CC(=O)C(F)(F)F)O.[Cu] |
Key on ui other cas no. |
14324-82-4 |
Piktogramme |
Irritant |
Herkunft des Produkts |
United States |
Haftungsausschluss und Informationen zu In-Vitro-Forschungsprodukten
Bitte beachten Sie, dass alle Artikel und Produktinformationen, die auf BenchChem präsentiert werden, ausschließlich zu Informationszwecken bestimmt sind. Die auf BenchChem zum Kauf angebotenen Produkte sind speziell für In-vitro-Studien konzipiert, die außerhalb lebender Organismen durchgeführt werden. In-vitro-Studien, abgeleitet von dem lateinischen Begriff "in Glas", beinhalten Experimente, die in kontrollierten Laborumgebungen unter Verwendung von Zellen oder Geweben durchgeführt werden. Es ist wichtig zu beachten, dass diese Produkte nicht als Arzneimittel oder Medikamente eingestuft sind und keine Zulassung der FDA für die Vorbeugung, Behandlung oder Heilung von medizinischen Zuständen, Beschwerden oder Krankheiten erhalten haben. Wir müssen betonen, dass jede Form der körperlichen Einführung dieser Produkte in Menschen oder Tiere gesetzlich strikt untersagt ist. Es ist unerlässlich, sich an diese Richtlinien zu halten, um die Einhaltung rechtlicher und ethischer Standards in Forschung und Experiment zu gewährleisten.