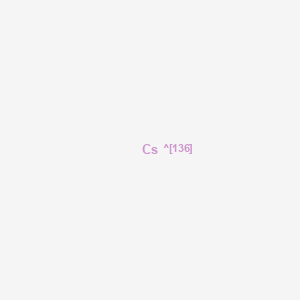
Cesium-136
- Klicken Sie auf QUICK INQUIRY, um ein Angebot von unserem Expertenteam zu erhalten.
- Mit qualitativ hochwertigen Produkten zu einem WETTBEWERBSFÄHIGEN Preis können Sie sich mehr auf Ihre Forschung konzentrieren.
Übersicht
Beschreibung
Cesium-136 is a radioactive isotope of cesium with a mass number of 136. It is a product of nuclear fission and has a half-life of approximately 13.01 days
Vorbereitungsmethoden
Synthetic Routes and Reaction Conditions: Cesium-136 is typically produced through the neutron bombardment of xenon-136 in a nuclear reactor or particle accelerator . The reaction involves the transformation of xenon-136 into this compound through neutron capture and subsequent beta decay.
Industrial Production Methods: Industrial production of this compound is not common due to its short half-life and the specialized equipment required for its synthesis. it can be produced in research reactors where controlled neutron flux is available .
Analyse Chemischer Reaktionen
Types of Reactions: Cesium-136 primarily undergoes beta decay, transforming into barium-136. This decay process is accompanied by the emission of beta particles and gamma rays .
Common Reagents and Conditions: The production of this compound involves the use of xenon-136 as a target material and a neutron source for bombardment. The reaction conditions include a high neutron flux environment, typically found in nuclear reactors or particle accelerators .
Major Products Formed: The primary product formed from the decay of this compound is barium-136. The beta decay process results in the emission of beta particles and gamma rays, which are detectable and useful in various scientific applications .
Wissenschaftliche Forschungsanwendungen
Key Applications
-
Particle Astrophysics
- Cesium-136 is utilized in large, low-background detectors aimed at detecting dark matter and studying rare decays of atomic nuclei. The gamma rays emitted during its decay are crucial for distinguishing rare signals from background radioactivity, enhancing the sensitivity of experiments searching for elusive particles like neutrinos .
-
Nuclear Forensics
- In nuclear forensics, this compound is valuable for analyzing cumulative fission yields. This analysis helps researchers understand fission events and the origins of nuclear materials, contributing to national security and non-proliferation efforts.
- Solar Neutrino Detection
Recent Discoveries and Research Findings
Recent studies have revealed low-lying isomeric states in this compound that open new avenues for research:
- Gamma-Ray Emission Studies : Research conducted by a team from Lawrence Berkeley National Laboratory and other institutions has focused on measuring gamma-ray emissions from this compound produced in nuclear reactions. These measurements provide critical data for modeling charged-current neutrino-nucleus interactions, which are essential in large xenon detectors used in particle physics experiments .
- Detection of Isomeric States : The discovery of excited states with lifetimes of approximately 95 and 157 nanoseconds allows researchers to identify these states distinctly from background noise. This capability enhances the precision of experiments like LUX-ZEPLIN, which aim to detect solar neutrinos and potential dark matter candidates .
Comparative Analysis with Similar Isotopes
Isotope | Half-Life | Key Applications |
---|---|---|
This compound | Short-lived | Dark matter detection, solar neutrino studies |
Cesium-137 | ~30 years | Medical applications (radiotherapy), industrial use |
Xenon-136 | Stable | Used as a target medium in dark matter experiments |
This compound's short half-life and specific applications in fundamental physics distinguish it from other isotopes like cesium-137, which has more extensive medical uses but longer half-life.
Wirkmechanismus
The primary mechanism of action for cesium-136 involves its beta decay process. When this compound undergoes beta decay, it transforms into barium-136, emitting beta particles and gamma rays in the process . These emissions are detectable and can be used to study various nuclear interactions and processes.
Molecular Targets and Pathways: In particle astrophysics, this compound interacts with neutrinos and other particles, providing valuable data for understanding fundamental physics phenomena . The gamma-ray emissions from this compound are used to identify and study rare interactions in large detectors.
Vergleich Mit ähnlichen Verbindungen
Cesium-136 can be compared with other isotopes of cesium and similar elements in the periodic table. Some of the similar compounds include:
Cesium-137: Another radioactive isotope of cesium with a longer half-life of about 30 years. It is commonly used in medical and industrial applications.
Barium-136: The decay product of this compound, which is stable and non-radioactive.
Xenon-136: The precursor isotope used in the production of this compound through neutron bombardment.
Uniqueness of this compound: this compound is unique due to its short half-life and specific applications in nuclear physics and astrophysics. Its ability to emit gamma rays during decay makes it valuable for detecting rare interactions and studying fundamental physics phenomena .
Eigenschaften
IUPAC Name |
cesium-136 |
Source
|
---|---|---|
Source | PubChem | |
URL | https://pubchem.ncbi.nlm.nih.gov | |
Description | Data deposited in or computed by PubChem | |
InChI |
InChI=1S/Cs/i1+3 |
Source
|
Source | PubChem | |
URL | https://pubchem.ncbi.nlm.nih.gov | |
Description | Data deposited in or computed by PubChem | |
InChI Key |
TVFDJXOCXUVLDH-AKLPVKDBSA-N |
Source
|
Source | PubChem | |
URL | https://pubchem.ncbi.nlm.nih.gov | |
Description | Data deposited in or computed by PubChem | |
Canonical SMILES |
[Cs] |
Source
|
Source | PubChem | |
URL | https://pubchem.ncbi.nlm.nih.gov | |
Description | Data deposited in or computed by PubChem | |
Isomeric SMILES |
[136Cs] |
Source
|
Source | PubChem | |
URL | https://pubchem.ncbi.nlm.nih.gov | |
Description | Data deposited in or computed by PubChem | |
Molecular Formula |
Cs |
Source
|
Source | PubChem | |
URL | https://pubchem.ncbi.nlm.nih.gov | |
Description | Data deposited in or computed by PubChem | |
DSSTOX Substance ID |
DTXSID50931411 |
Source
|
Record name | (~136~Cs)Caesium | |
Source | EPA DSSTox | |
URL | https://comptox.epa.gov/dashboard/DTXSID50931411 | |
Description | DSSTox provides a high quality public chemistry resource for supporting improved predictive toxicology. | |
Molecular Weight |
135.90731 g/mol |
Source
|
Source | PubChem | |
URL | https://pubchem.ncbi.nlm.nih.gov | |
Description | Data deposited in or computed by PubChem | |
CAS No. |
14234-29-8 |
Source
|
Record name | (~136~Cs)Caesium | |
Source | EPA DSSTox | |
URL | https://comptox.epa.gov/dashboard/DTXSID50931411 | |
Description | DSSTox provides a high quality public chemistry resource for supporting improved predictive toxicology. | |
Haftungsausschluss und Informationen zu In-Vitro-Forschungsprodukten
Bitte beachten Sie, dass alle Artikel und Produktinformationen, die auf BenchChem präsentiert werden, ausschließlich zu Informationszwecken bestimmt sind. Die auf BenchChem zum Kauf angebotenen Produkte sind speziell für In-vitro-Studien konzipiert, die außerhalb lebender Organismen durchgeführt werden. In-vitro-Studien, abgeleitet von dem lateinischen Begriff "in Glas", beinhalten Experimente, die in kontrollierten Laborumgebungen unter Verwendung von Zellen oder Geweben durchgeführt werden. Es ist wichtig zu beachten, dass diese Produkte nicht als Arzneimittel oder Medikamente eingestuft sind und keine Zulassung der FDA für die Vorbeugung, Behandlung oder Heilung von medizinischen Zuständen, Beschwerden oder Krankheiten erhalten haben. Wir müssen betonen, dass jede Form der körperlichen Einführung dieser Produkte in Menschen oder Tiere gesetzlich strikt untersagt ist. Es ist unerlässlich, sich an diese Richtlinien zu halten, um die Einhaltung rechtlicher und ethischer Standards in Forschung und Experiment zu gewährleisten.