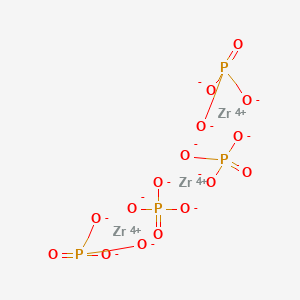
Zirconium phosphate
Übersicht
Beschreibung
Zirconium phosphates (zirconium hydrogen phosphate) are acidic, inorganic cation exchange materials that have a layered structure with formula Zr(HPO 4) 2 ∙nH 2 O . These salts have high thermal and chemical stability, solid state ion conductivity, resistance to ionizing radiation, and the capacity to incorporate different types of molecules with different sizes between their layers .
Synthesis Analysis
Zirconium phosphate can be synthesized by impregnation of Zr(OH)4 and m-ZrO2 with an aqueous solution of (NH4)2HPO4 . Another method involves the addition of zirconyl propionate to phosphoric acid in alcoholic media, which leads to the formation of transparent gels containing solvent intercalated zirconium phosphate (ZrP) nanoparticles with hexagonal shape and a planar size of about 40 nm .
Molecular Structure Analysis
The layered structure of α-Zirconium phosphate consists of Zr(IV) ions situated alternately slightly above and below the ab plane, forming an octahedron with the oxygen atoms of the tetrahedral phosphate groups . The structure of γ-Zirconium phosphate consists of Zr(IV) atoms octahedrally coordinated to four different oxygen atoms of an orthophosphate .
Chemical Reactions Analysis
Zirconium phosphate has been used in several applications such as drug delivery, catalysis, nanocomposite, nuclear waste management, and clinical dialyzer . The presence of PO4 and P-OH groups on porous ZrP is responsible for Brønsted’s acidic properties, and the Zr4+ centers provide Lewis acidic properties, making them a fascinating functional material .
Physical And Chemical Properties Analysis
Zirconium phosphate shows very high affinity for Rb+, allowing its enrichment from low-concentration solutions . The presence of a robust inorganic structure enables its application in relatively high temperature reactions .
Wissenschaftliche Forschungsanwendungen
Synthesis, Properties, and Applications : ZrP and its derivatives, such as zirconium phosphonate, are known for their high ion-exchange capability, excellent thermal stability, and good biocompatibility. They are used in various applications including as catalysts, flame retardants, drug delivery agents, lubrication modifiers, electrolyte membranes in fuel cells, and anti-corrosive agents (Xiao & Liu, 2018).
Lubricant Additives : ZrP has been shown to effectively reduce friction in both non-aqueous and aqueous media, making it a promising additive in lubricants for various sectors including manufacturing and automobiles (He et al., 2014).
Wastewater Treatment : ZrP is used as a sorbent for heavy metal cations or dye molecules in wastewater treatments. Its combination with polymers or other inorganic materials has been explored for large-scale applications (Pica, 2021).
Electrolyte Membranes in Fuel Cells : ZrP has been investigated as a proton conductor in direct hydrocarbon polymer electrolyte membrane fuel cells, particularly at temperatures higher than those of conventional fuel cells (Al-Othman et al., 2010).
Oxygen Evolution Reaction : ZrP has been studied for its use in electrocatalysis of the oxygen evolution reaction (OER), particularly focusing on how the preparation of ZrP affects its activity (Ramos-Garcés & Colón, 2020).
Fluoride Scavenging : ZrP has been developed as an efficient adsorbent for fluoride scavenging, showing good chemical stability in acidic or basic environments, and outperforming many conventional metal oxides (Zhang et al., 2017).
Mesoporous Sol–Gel Zirconium Phosphates for Fuel Cell Applications : Mesoporous zirconium phosphates have been synthesized for use as a proton conductor in proton exchange membrane (PEM) fuel cells, showing potential as a surface functionalized solid acid proton conductor (Hogarth et al., 2005).
Wirkmechanismus
Safety and Hazards
Zirconium phosphate should be handled with care. Avoid dust formation, breathing mist, gas or vapours, and contact with skin and eye. Use personal protective equipment and wear chemical impermeable gloves. Ensure adequate ventilation, remove all sources of ignition, and evacuate personnel to safe areas .
Zukünftige Richtungen
Zirconium phosphate has drawn interdisciplinary attention as an ion exchange material and a competent host for surface functionalization and intercalation . The properties of these materials, such as structure, porosity, and degree of crystallinity, can be controlled by varying the synthetic conditions . Existing problems and challenges related to the synthesis and applications, and future perspectives will be discussed .
Eigenschaften
IUPAC Name |
zirconium(4+);tetraphosphate | |
---|---|---|
Source | PubChem | |
URL | https://pubchem.ncbi.nlm.nih.gov | |
Description | Data deposited in or computed by PubChem | |
InChI |
InChI=1S/4H3O4P.3Zr/c4*1-5(2,3)4;;;/h4*(H3,1,2,3,4);;;/q;;;;3*+4/p-12 | |
Source | PubChem | |
URL | https://pubchem.ncbi.nlm.nih.gov | |
Description | Data deposited in or computed by PubChem | |
InChI Key |
LEHFSLREWWMLPU-UHFFFAOYSA-B | |
Source | PubChem | |
URL | https://pubchem.ncbi.nlm.nih.gov | |
Description | Data deposited in or computed by PubChem | |
Canonical SMILES |
[O-]P(=O)([O-])[O-].[O-]P(=O)([O-])[O-].[O-]P(=O)([O-])[O-].[O-]P(=O)([O-])[O-].[Zr+4].[Zr+4].[Zr+4] | |
Source | PubChem | |
URL | https://pubchem.ncbi.nlm.nih.gov | |
Description | Data deposited in or computed by PubChem | |
Molecular Formula |
O16P4Zr3 | |
Source | PubChem | |
URL | https://pubchem.ncbi.nlm.nih.gov | |
Description | Data deposited in or computed by PubChem | |
Related CAS |
13772-29-7 (zirconium(+4)salt[2:1]), 28132-50-5 (hydrochloride salt[2:2:1]), 34370-53-1 (hydrochloride salt[2:1:1]) | |
Record name | Zirconium phosphate | |
Source | ChemIDplus | |
URL | https://pubchem.ncbi.nlm.nih.gov/substance/?source=chemidplus&sourceid=0013765952 | |
Description | ChemIDplus is a free, web search system that provides access to the structure and nomenclature authority files used for the identification of chemical substances cited in National Library of Medicine (NLM) databases, including the TOXNET system. | |
DSSTOX Substance ID |
DTXSID40160258 | |
Record name | Zirconium phosphate | |
Source | EPA DSSTox | |
URL | https://comptox.epa.gov/dashboard/DTXSID40160258 | |
Description | DSSTox provides a high quality public chemistry resource for supporting improved predictive toxicology. | |
Molecular Weight |
653.56 g/mol | |
Source | PubChem | |
URL | https://pubchem.ncbi.nlm.nih.gov | |
Description | Data deposited in or computed by PubChem | |
Product Name |
Zirconium phosphate | |
CAS RN |
13765-95-2 | |
Record name | Zirconium phosphate | |
Source | ChemIDplus | |
URL | https://pubchem.ncbi.nlm.nih.gov/substance/?source=chemidplus&sourceid=0013765952 | |
Description | ChemIDplus is a free, web search system that provides access to the structure and nomenclature authority files used for the identification of chemical substances cited in National Library of Medicine (NLM) databases, including the TOXNET system. | |
Record name | Zirconium phosphate | |
Source | EPA DSSTox | |
URL | https://comptox.epa.gov/dashboard/DTXSID40160258 | |
Description | DSSTox provides a high quality public chemistry resource for supporting improved predictive toxicology. | |
Record name | Phosphoric acid, zirconium salt | |
Source | European Chemicals Agency (ECHA) | |
URL | https://echa.europa.eu/substance-information/-/substanceinfo/100.033.963 | |
Description | The European Chemicals Agency (ECHA) is an agency of the European Union which is the driving force among regulatory authorities in implementing the EU's groundbreaking chemicals legislation for the benefit of human health and the environment as well as for innovation and competitiveness. | |
Explanation | Use of the information, documents and data from the ECHA website is subject to the terms and conditions of this Legal Notice, and subject to other binding limitations provided for under applicable law, the information, documents and data made available on the ECHA website may be reproduced, distributed and/or used, totally or in part, for non-commercial purposes provided that ECHA is acknowledged as the source: "Source: European Chemicals Agency, http://echa.europa.eu/". Such acknowledgement must be included in each copy of the material. ECHA permits and encourages organisations and individuals to create links to the ECHA website under the following cumulative conditions: Links can only be made to webpages that provide a link to the Legal Notice page. | |
Synthesis routes and methods I
Procedure details
Synthesis routes and methods II
Procedure details
Synthesis routes and methods III
Procedure details
Synthesis routes and methods IV
Procedure details
Synthesis routes and methods V
Procedure details
Retrosynthesis Analysis
AI-Powered Synthesis Planning: Our tool employs the Template_relevance Pistachio, Template_relevance Bkms_metabolic, Template_relevance Pistachio_ringbreaker, Template_relevance Reaxys, Template_relevance Reaxys_biocatalysis model, leveraging a vast database of chemical reactions to predict feasible synthetic routes.
One-Step Synthesis Focus: Specifically designed for one-step synthesis, it provides concise and direct routes for your target compounds, streamlining the synthesis process.
Accurate Predictions: Utilizing the extensive PISTACHIO, BKMS_METABOLIC, PISTACHIO_RINGBREAKER, REAXYS, REAXYS_BIOCATALYSIS database, our tool offers high-accuracy predictions, reflecting the latest in chemical research and data.
Strategy Settings
Precursor scoring | Relevance Heuristic |
---|---|
Min. plausibility | 0.01 |
Model | Template_relevance |
Template Set | Pistachio/Bkms_metabolic/Pistachio_ringbreaker/Reaxys/Reaxys_biocatalysis |
Top-N result to add to graph | 6 |
Feasible Synthetic Routes
Haftungsausschluss und Informationen zu In-Vitro-Forschungsprodukten
Bitte beachten Sie, dass alle Artikel und Produktinformationen, die auf BenchChem präsentiert werden, ausschließlich zu Informationszwecken bestimmt sind. Die auf BenchChem zum Kauf angebotenen Produkte sind speziell für In-vitro-Studien konzipiert, die außerhalb lebender Organismen durchgeführt werden. In-vitro-Studien, abgeleitet von dem lateinischen Begriff "in Glas", beinhalten Experimente, die in kontrollierten Laborumgebungen unter Verwendung von Zellen oder Geweben durchgeführt werden. Es ist wichtig zu beachten, dass diese Produkte nicht als Arzneimittel oder Medikamente eingestuft sind und keine Zulassung der FDA für die Vorbeugung, Behandlung oder Heilung von medizinischen Zuständen, Beschwerden oder Krankheiten erhalten haben. Wir müssen betonen, dass jede Form der körperlichen Einführung dieser Produkte in Menschen oder Tiere gesetzlich strikt untersagt ist. Es ist unerlässlich, sich an diese Richtlinien zu halten, um die Einhaltung rechtlicher und ethischer Standards in Forschung und Experiment zu gewährleisten.