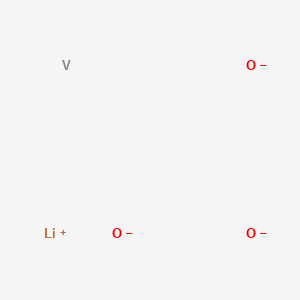
Lithium vanadium oxide (LiVO3)
Übersicht
Beschreibung
Lithium vanadium oxide (LiVO₃) is a vanadium-based compound with the molecular formula LiVO₃ and a molecular weight of 211.76 g/mol . It crystallizes in a monoclinic structure (space group C2/c) under ambient conditions and exhibits unique electrochemical properties, making it a candidate for lithium-ion battery (LIB) cathodes . LiVO₃ is synthesized via dehydration of lithium decavanadate (Li₆[V₁₀O₂₈]·16H₂O) at ~120°C or through precipitation and hydrothermal methods using alkaline LiOH and LiVO₃ solutions .
LiVO₃ undergoes a conversion-type lithiation mechanism, where initial Li⁺ insertion forms a rock-salt Li₂VO₃ phase, followed by structural disintegration into metallic vanadium and Li₂O . This mechanism contributes to high theoretical capacities (~776 mAh/g in LiVO₃/C core-shell structures) but poses challenges for long-term cycling stability . Nanostructuring strategies, such as nanoplate-stacked morphologies or carbon coatings, mitigate vanadium dissolution and enhance rate performance (e.g., 205 mAh/g after 2,000 cycles at 10 A/g) .
Wissenschaftliche Forschungsanwendungen
Lithium-Ion Batteries (LIBs)
Anode Material Development
LiVO3 has been extensively studied as an anode material in lithium-ion batteries due to its high theoretical capacity and stability. Research indicates that lithium vanadate oxides, particularly Li3VO4, have shown promising results when combined with conductive matrices like MXenes to enhance their electrochemical performance. For instance, a composite of Li3VO4 and Ti3C2Tx MXene demonstrated a capacity of 151.6 mA h g−1 at a high rate of 5 C, significantly outperforming traditional graphite anodes .
Fast-Charging Capabilities
The fast-charging capabilities of LiVO3 derivatives have been highlighted in recent studies. The Li3VO4/Ti3C2Tx composite not only exhibited high capacity but also maintained structural integrity without dendrite formation during rapid charging cycles, which is a common issue with conventional anodes . This characteristic enhances the safety and longevity of the batteries.
Zinc-Ion Batteries (ZIBs)
Intercalation Cathode
Layered lithium vanadium oxide (LiV3O8) has emerged as a promising intercalation cathode for zinc-ion batteries. This material shows high storage capacities and favorable electrochemical behaviors, making it suitable for next-generation energy storage systems . The ability to intercalate zinc ions allows for efficient charge/discharge cycles, which is critical for practical applications in energy storage.
Polymer Lithium-Ion Batteries (PLIBs)
Material Composition
LiVO3 is also utilized in the development of polymer lithium-ion batteries. Its incorporation into battery formulations enhances the overall electrochemical performance and stability of the polymer matrix . The compound's properties contribute to improved conductivity and energy density, which are essential for the advancement of flexible and lightweight battery technologies.
Coatings and Nanostructures
Advanced Coatings
Beyond battery applications, LiVO3 is used in coatings for various substrates due to its unique chemical properties. It can be applied in nanowires and nanofibers, enhancing the mechanical strength and thermal stability of materials used in electronics and other industries .
Textiles and Plastics
The integration of LiVO3 into textiles and plastics has been explored for creating smart materials that can respond to environmental changes or enhance energy storage capabilities. This application opens avenues for innovative product designs that incorporate energy-harvesting technologies.
Data Summary
Application Area | Material Used | Key Properties | Performance Metrics |
---|---|---|---|
Lithium-Ion Batteries | Li3VO4/Ti3C2Tx | High capacity, fast charging | 151.6 mA h g−1 at 5 C |
Zinc-Ion Batteries | LiV3O8 | High storage capacity | Efficient charge/discharge cycles |
Polymer Lithium-Ion Batteries | LiVO3 | Enhanced conductivity | Improved energy density |
Coatings | LiVO3 | Mechanical strength | Enhanced thermal stability |
Textiles and Plastics | LiVO3 | Smart material properties | Energy-harvesting capabilities |
Case Studies
-
Case Study 1: Fast-Charging Anode Development
A study synthesized a composite anode using Li3VO4 embedded in Ti3C2Tx MXene framework. The results showed significant improvements in both safety and performance compared to traditional graphite anodes, paving the way for safer fast-charging solutions in LIBs . -
Case Study 2: Zinc-Ion Battery Implementation
Research on LiV3O8 as a cathode material demonstrated its potential in ZIBs, showcasing high efficiency during cycling tests. The findings suggest that this material could be pivotal in developing sustainable energy storage systems that utilize abundant zinc resources .
Wirkmechanismus
The mechanism by which lithium vanadium oxide exerts its effects involves the intercalation and deintercalation of lithium ions. This process enhances the lithium-ion storage ability and electron transfer capabilities of the electrode . The unique core-shell structure of lithium vanadium oxide, where carbon encapsulation provides many interfaces, enhances electron conductivity and structural consistency .
Vergleich Mit ähnlichen Verbindungen
Lithium Vanadates
Key Differences :
- Mechanism : LiVO₃ follows a conversion reaction, whereas LiV₃O₈ and Li₃VO₄ rely on insertion, leading to differences in capacity and stability .
- Rate Performance : LiVO₃/C core-shell structures achieve superior rate capability (205 mAh/g at 10 A/g) compared to LiV₃O₈ (~100 mAh/g at 2 A/g) .
- Synthesis : LiV₃O₈ is often synthesized via hydrothermal methods, while LiVO₃ requires controlled dehydration or precipitation .
Other Vanadium Oxides
Key Differences :
- Redox Activity : LiVO₃ and V₂O₅ utilize vanadium redox (V³⁺/V⁴⁺/V⁵⁺), while Li₃V₂(PO₄)₃ involves V³⁺/V⁴⁺ transitions .
- Energy Density : LiVO₃’s conversion mechanism provides higher capacity than Li₃V₂(PO₄)₃ but lower than V₂O₅ .
Polyoxovanadates (POVs)
POVs like K₇[MnV₁₃O₃₈] and Li₇[V₁₅O₃₆(CO₃)] are molecular clusters used as LIB cathodes. They exhibit capacities of ~200–250 mAh/g but suffer from thermal instability and structural rearrangements during cycling . In contrast, LiVO₃ offers better thermal stability and higher practical capacities due to its solid-state oxide structure .
Research Findings and Challenges
- LiVO₃ Advantages: High capacity via conversion reaction . Enhanced kinetics through nanostructuring (e.g., pseudo-capacitive contributions in LiVO₃/C) .
- Challenges :
- Comparative Outlook : LiVO₃ outperforms LiV₃O₈ and Li₃VO₄ in capacity but requires further optimization to match the cycling stability of Li₃V₂(PO₄)₃ or the energy density of V₂O₅ .
Biologische Aktivität
Lithium vanadium oxide (LiVO3) is a compound of increasing interest in various fields, particularly in electrochemistry and materials science. This article explores its biological activity, focusing on its applications as an electrode material in lithium-ion batteries (LIBs), and its potential implications in biomedical fields.
Overview of Lithium Vanadium Oxide
Lithium vanadium oxide exists in several forms, with LiV3O8 and Li3VO4 being notable for their electrochemical properties. These compounds exhibit significant redox activity, making them suitable for use in energy storage devices.
Theoretical Capacity : Lithium vanadium oxide has a high theoretical capacity, with Li3VO4 exhibiting up to 394 mAh/g. However, its practical application is often limited by low electrical conductivity.
Conductivity Improvement : Research has shown that combining LiVO3 with conductive materials like graphene can enhance its electrochemical performance. For instance, a composite of lithium vanadium oxide and graphene (LVO/GN) demonstrated an initial capacity of 350.2 mAh/g at a rate of 0.5C, maintaining 86.8% capacity after 200 cycles .
Case Study 1: Electrochemical Performance Enhancement
A study investigated the synthesis of LiVO3 using the sol-gel method, resulting in uniform nanoparticle morphology. The addition of graphene significantly improved the distribution and conductivity of the composite, leading to enhanced cycling performance .
Material | Initial Capacity (mAh/g) | Capacity Retention After 200 Cycles (%) |
---|---|---|
Li3VO4 | 394 | - |
LVO/GN Composite | 350.2 | 86.8 |
Pure LiVO3 | - | 71.8 |
Case Study 2: Structural Stability and Phase Transformation
Another research highlighted the structural changes in LiV3O8 upon cycling in LIBs. The study revealed that initial lithium intercalation occurs without significant structural alteration; however, prolonged cycling leads to phase transformations that can affect stability and capacity retention .
Mechanisms of Biological Activity
The biological activity of lithium vanadium oxide can be attributed to several factors:
- Redox Reactions : The ability of vanadium to switch between different oxidation states (V(IV) and V(V)) facilitates electron transfer processes essential for battery operation.
- Ionic Conductivity : The presence of lithium ions enhances ionic conductivity, which is crucial for battery performance.
- Structural Integrity : Maintaining structural integrity during charge-discharge cycles is vital for the longevity and efficiency of electrode materials.
Future Directions and Applications
The potential applications of lithium vanadium oxide extend beyond energy storage:
- Biomedical Applications : Research is ongoing into the use of LiVO3 in drug delivery systems and as a biocompatible material due to its electrochemical properties.
- Environmental Impact : The compound's stability under various conditions makes it a candidate for environmental sensors and remediation technologies.
Q & A
Q. Basic: What are the primary synthesis methods for LiVO₃, and how do parameters like pH influence the resulting phases?
Answer:
LiVO₃ is synthesized via precipitation and hydrothermal methods. In precipitation, adjusting the pH of lithium hydroxide and vanadium pentoxide solutions controls phase formation. For example:
- At pH = 2–6, precipitation yields LiVO₃/Li₄V₁₀O₂₇ mixtures .
- Oxygen treatment of these precipitates produces LiVO₃/Li₃VO₄ composites .
Hydrothermal synthesis at similar pH values generates LiV₃O₈ nanobelts .
Key Insight: pH governs the solubility and condensation of vanadium species, directly affecting crystallinity and phase purity. XRD and electrochemical profiling are critical for validating synthesis outcomes .
Q. Basic: How does the lithiation mechanism of LiVO₃ differ from other vanadium oxides?
Answer:
LiVO₃ undergoes a unique two-step lithiation:
Insertion Phase: Initial Li⁺ intercalation forms rock-salt Li₂VO₃, retaining the host structure .
Conversion Phase: Further lithiation destabilizes the lattice, decomposing Li₂.₅VO₃ into metallic V and Li₂O .
In contrast, layered oxides like LiV₃O₈ exhibit single-phase intercalation without structural collapse .
Methodological Note: Galvanostatic Intermittent Titration Technique (GITT) and impedance spectroscopy (EIS) are used to distinguish insertion/conversion kinetics .
Q. Basic: What structural characterization techniques are essential for analyzing LiVO₃, and what insights do they provide?
Answer:
- XRD: Identifies phases (e.g., monoclinic LiVO₃, JCPDS 32-0606) and lattice parameters .
- EXAFS: Probes local V-O/V-V coordination changes during phase transitions (e.g., V³⁺ trimer formation below 450 K) .
- Neutron Scattering: Reveals disordered rock-salt structures and suppresses dendrite-forming instabilities .
Critical Step: Pairing XRD with spectroscopic methods resolves ambiguities in polymorphic transformations .
Q. Advanced: How do phase transitions in LiVO₃ during cycling affect its electrochemical performance?
Answer:
LiVO₃ exhibits a first-order phase transition at 450–550 K due to V³⁺-V³⁺ interactions forming 2D trimer clusters . During cycling:
- Structural distortion from tetrahedral Li⁺ site occupancy in Li₂.₅VO₃ triggers lattice breakdown, reducing cyclability .
- Reversible disordering in the rock-salt phase at high Li content enables rapid charge/discharge but risks capacity fade .
Mitigation Strategy: Operando PDF analysis tracks real-time structural reversibility to optimize voltage windows .
Q. Advanced: What advanced operando techniques are used to study LiVO₃'s structural dynamics during lithiation?
Answer:
- Operando PDF (Pair Distribution Function): Captures transient Li⁺ positions and bond-length evolution (e.g., 1.7 Å V-O distances in charged states) .
- XANES: Monitors vanadium oxidation states during conversion (V⁵⁺ → V³⁺/V⁰) .
- EIS with GITT: Quantifies Li⁺ diffusion coefficients (D~10⁻¹²–10⁻¹⁴ cm²/s) and interfacial resistance .
Application: These techniques correlate structural disintegration with capacity loss, guiding electrode design .
Q. Advanced: How do computational methods like DFT contribute to understanding LiVO₃'s properties?
Answer:
- DFT Calculations: Predict Li⁺ migration barriers in rock-salt frameworks and identify metastable intermediates (e.g., Li₂.₅VO₃) .
- Machine Learning: Accelerates discovery of open-tunnel oxides (e.g., MoVO analogs) by screening Li⁺/Mg²⁺ adsorption potentials (~4–5 eV) .
Outcome: Models prioritize synthesis targets with optimal ion-transport pathways and structural stability .
Q. Advanced: What strategies mitigate capacity fading in LiVO₃ electrodes?
Answer:
- Nanostructuring: Hydrothermally synthesized LiV₃O₈ nanobelts improve Li⁺ accessibility and reduce pulverization .
- Composite Design: Blending LiVO₃ with conductive matrices (e.g., carbon) buffers volume changes during conversion .
- Voltage Control: Restricting lithiation to the insertion phase (≤1.5 Li⁺ per formula unit) avoids structural collapse .
Validation: Long-term cycling tests (e.g., 100+ cycles) with capacity retention >80% validate these approaches .
Vorbereitungsmethoden
Solid-State Synthesis: Conventional and Advanced Approaches
Solid-state synthesis remains the most widely used method for LiVO₃ production due to its scalability and cost-effectiveness. The process involves mixing lithium and vanadium precursors in stoichiometric ratios, followed by high-temperature calcination.
Precursor Selection and Stoichiometric Control
The molar ratio of lithium to vanadium precursors critically influences phase purity. Patent US6136476A recommends a Li:V ratio of 1.0:3.0 to 1.5:3.0 to avoid secondary phases like LiV₂O₅ or unreacted V₂O₅ . Common precursors include:
-
Lithium sources : Li₂CO₃, LiOH·H₂O
-
Vanadium sources : V₂O₅, NH₄VO₃
A typical procedure involves:
-
Dry grinding precursors in a mortar or ball mill for homogeneity.
-
Calcining in an oxidizing atmosphere (air or O₂) at 550–630°C for ≤24 hours .
-
Rapid cooling to room temperature to preserve phase integrity.
Table 1: Solid-State Synthesis Parameters and Outcomes
Precursors | Li:V Ratio | Temperature (°C) | Time (h) | Phase Purity | Source |
---|---|---|---|---|---|
Li₂CO₃ + V₂O₅ | 1.2:3.0 | 550 | 12 | 98% LiVO₃ | |
LiOH + NH₄VO₃ | 1.5:3.0 | 630 | 24 | 95% LiVO₃ |
Flash Calcining and Jet Milling Innovations
To enhance reaction kinetics, advanced solid-state methods employ flash calcining , where precursors are heated rapidly (≥100°C/min) to sub-melting temperatures (≤550°C) . This reduces energy consumption and prevents vanadium sublimation. Additionally, jet milling improves particle mixing and reduces agglomeration, yielding LiVO₃ with uniform morphology .
Hydrothermal Synthesis: Low-Temperature Crystallization
Hydrothermal synthesis offers precise control over particle size and crystallinity at lower temperatures (120–200°C). This method is ideal for producing nanostructured LiVO₃ with high surface area, beneficial for battery applications.
Reaction Mechanism and Optimization
A representative protocol involves:
-
Dissolving LiOH and NH₄VO₃ in deionized water.
-
Transferring the solution to a Teflon-lined autoclave.
-
Heating at 180°C for 12–48 hours under autogenous pressure.
-
Annealing the precipitate in N₂ at 400–500°C to enhance crystallinity.
Synchrotron-based operando energy-dispersive X-ray diffraction (EDXRD) reveals that hydrothermal LiVO₃ forms a layered structure with intercalation-friendly channels. Post-annealing in nitrogen minimizes oxygen vacancies, which can degrade ionic conductivity .
Table 2: Hydrothermal Synthesis Conditions
Precursors | Temperature (°C) | Time (h) | Annealing Atmosphere | Crystallite Size (nm) |
---|---|---|---|---|
LiOH + NH₄VO₃ | 180 | 24 | N₂ | 25 ± 3 |
LiNO₃ + V₂O₅ | 200 | 48 | Ar | 40 ± 5 |
Carbon-Coated LiVO₃ Core-Shell Structures
Carbon coating improves LiVO₃’s electronic conductivity and mitigates volume expansion during lithiation. A 2022 study demonstrated that LiVO₃/C composites achieve 776 mAh g⁻¹ at 0.2 A g⁻¹, outperforming bare LiVO₃ by 40% .
Synthesis via Oxalic Acid Carbonization
The core-shell structure is fabricated through:
-
Hydrothermal synthesis of LiVO₃ nanoparticles.
-
Mixing with oxalic acid (C₂H₂O₄) as a carbon precursor.
-
Carbonization at 600–700°C in inert atmosphere (Ar/N₂).
Transmission electron microscopy (TEM) confirms a 5–10 nm carbon layer uniformly encapsulating LiVO₃ particles . This coating reduces charge-transfer resistance from 85 Ω to 32 Ω, enabling high-rate cycling (205 mAh g⁻¹ at 10 A g⁻¹ over 2000 cycles) .
Computational Insights into Synthesis-Structure Relationships
Density functional theory (DFT) calculations predict that LiVO₃’s lithiation proceeds via a two-phase reaction between LiVO₃ and Li₂VO₃ . The metastable Li₂VO₃ phase forms at Li:V > 1.5:3.0, necessitating precise stoichiometric control during synthesis. Molecular dynamics (MD) simulations further reveal that exceeding Li₂.₅VO₃ induces structural amorphization, degrading cyclability .
Comparative Analysis of Synthesis Methods
Table 3: Method Comparison for LiVO₃ Preparation
Method | Crystallinity | Capacity Retention (0.2 A g⁻¹) | Scalability | Cost |
---|---|---|---|---|
Solid-State | High | 68% (100 cycles) | High | Low |
Hydrothermal | Moderate | 82% (200 cycles) | Moderate | Medium |
Core-Shell | High | 89% (200 cycles) | Low | High |
Eigenschaften
IUPAC Name |
lithium;oxygen(2-);vanadium | |
---|---|---|
Source | PubChem | |
URL | https://pubchem.ncbi.nlm.nih.gov | |
Description | Data deposited in or computed by PubChem | |
InChI |
InChI=1S/Li.3O.V/q+1;3*-2; | |
Source | PubChem | |
URL | https://pubchem.ncbi.nlm.nih.gov | |
Description | Data deposited in or computed by PubChem | |
InChI Key |
IZPPMPGSWJHKSD-UHFFFAOYSA-N | |
Source | PubChem | |
URL | https://pubchem.ncbi.nlm.nih.gov | |
Description | Data deposited in or computed by PubChem | |
Canonical SMILES |
[Li+].[O-2].[O-2].[O-2].[V] | |
Source | PubChem | |
URL | https://pubchem.ncbi.nlm.nih.gov | |
Description | Data deposited in or computed by PubChem | |
Molecular Formula |
LiO3V-5 | |
Source | PubChem | |
URL | https://pubchem.ncbi.nlm.nih.gov | |
Description | Data deposited in or computed by PubChem | |
DSSTOX Substance ID |
DTXSID601014303 | |
Record name | Lithium vanadium oxide (LiVO3) | |
Source | EPA DSSTox | |
URL | https://comptox.epa.gov/dashboard/DTXSID601014303 | |
Description | DSSTox provides a high quality public chemistry resource for supporting improved predictive toxicology. | |
Molecular Weight |
105.9 g/mol | |
Source | PubChem | |
URL | https://pubchem.ncbi.nlm.nih.gov | |
Description | Data deposited in or computed by PubChem | |
CAS No. |
15060-59-0 | |
Record name | Lithium vanadium oxide (LiVO3) | |
Source | ChemIDplus | |
URL | https://pubchem.ncbi.nlm.nih.gov/substance/?source=chemidplus&sourceid=0015060590 | |
Description | ChemIDplus is a free, web search system that provides access to the structure and nomenclature authority files used for the identification of chemical substances cited in National Library of Medicine (NLM) databases, including the TOXNET system. | |
Record name | Lithium vanadium oxide (LiVO3) | |
Source | EPA Chemicals under the TSCA | |
URL | https://www.epa.gov/chemicals-under-tsca | |
Description | EPA Chemicals under the Toxic Substances Control Act (TSCA) collection contains information on chemicals and their regulations under TSCA, including non-confidential content from the TSCA Chemical Substance Inventory and Chemical Data Reporting. | |
Record name | Lithium vanadium oxide (LiVO3) | |
Source | EPA DSSTox | |
URL | https://comptox.epa.gov/dashboard/DTXSID601014303 | |
Description | DSSTox provides a high quality public chemistry resource for supporting improved predictive toxicology. | |
Record name | Lithium vanadium trioxide | |
Source | European Chemicals Agency (ECHA) | |
URL | https://echa.europa.eu/substance-information/-/substanceinfo/100.035.553 | |
Description | The European Chemicals Agency (ECHA) is an agency of the European Union which is the driving force among regulatory authorities in implementing the EU's groundbreaking chemicals legislation for the benefit of human health and the environment as well as for innovation and competitiveness. | |
Explanation | Use of the information, documents and data from the ECHA website is subject to the terms and conditions of this Legal Notice, and subject to other binding limitations provided for under applicable law, the information, documents and data made available on the ECHA website may be reproduced, distributed and/or used, totally or in part, for non-commercial purposes provided that ECHA is acknowledged as the source: "Source: European Chemicals Agency, http://echa.europa.eu/". Such acknowledgement must be included in each copy of the material. ECHA permits and encourages organisations and individuals to create links to the ECHA website under the following cumulative conditions: Links can only be made to webpages that provide a link to the Legal Notice page. | |
Haftungsausschluss und Informationen zu In-Vitro-Forschungsprodukten
Bitte beachten Sie, dass alle Artikel und Produktinformationen, die auf BenchChem präsentiert werden, ausschließlich zu Informationszwecken bestimmt sind. Die auf BenchChem zum Kauf angebotenen Produkte sind speziell für In-vitro-Studien konzipiert, die außerhalb lebender Organismen durchgeführt werden. In-vitro-Studien, abgeleitet von dem lateinischen Begriff "in Glas", beinhalten Experimente, die in kontrollierten Laborumgebungen unter Verwendung von Zellen oder Geweben durchgeführt werden. Es ist wichtig zu beachten, dass diese Produkte nicht als Arzneimittel oder Medikamente eingestuft sind und keine Zulassung der FDA für die Vorbeugung, Behandlung oder Heilung von medizinischen Zuständen, Beschwerden oder Krankheiten erhalten haben. Wir müssen betonen, dass jede Form der körperlichen Einführung dieser Produkte in Menschen oder Tiere gesetzlich strikt untersagt ist. Es ist unerlässlich, sich an diese Richtlinien zu halten, um die Einhaltung rechtlicher und ethischer Standards in Forschung und Experiment zu gewährleisten.