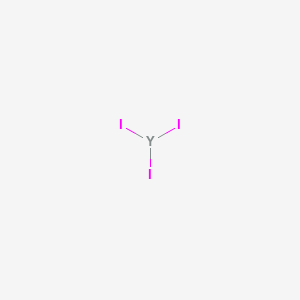
Yttriumiodid
- Klicken Sie auf QUICK INQUIRY, um ein Angebot von unserem Expertenteam zu erhalten.
- Mit qualitativ hochwertigen Produkten zu einem WETTBEWERBSFÄHIGEN Preis können Sie sich mehr auf Ihre Forschung konzentrieren.
Übersicht
Beschreibung
Yttrium iodide is a binary inorganic compound composed of yttrium and iodine, with the chemical formula YI₃. It forms colorless crystals that are soluble in water and ethanol but insoluble in diethyl ether . This compound is known for its unique properties and applications in various fields, including chemistry, biology, medicine, and industry.
Wissenschaftliche Forschungsanwendungen
Yttrium iodide has several scientific research applications:
Wirkmechanismus
Target of Action
Yttrium iodide, also known as Yttrium(III) iodide, is a binary inorganic compound . It primarily targets the reticuloendothelial system , specifically the liver and spleen . It is also used as a precursor for YBCO superconducting materials .
Mode of Action
Yttrium iodide interacts with its targets through ionic interactions . In the case of the reticuloendothelial system, yttrium iodide is taken up by phagocytic cells in the liver and spleen . In the context of superconducting materials, yttrium iodide serves as a precursor in their synthesis .
Biochemical Pathways
It is known that yttrium iodide plays a role in the transport of sodium ions in the yttrium-iodide sodium superionic conductor na3yi6 . This suggests that yttrium iodide may influence ion transport mechanisms within the cell.
Pharmacokinetics
It is known that after administration, most of the yttrium is distributed into the liver, bone, and spleen . Yttrium disappears from the blood within 1 day but is retained in the organs for a long time .
Result of Action
The result of yttrium iodide’s action depends on its application. In the context of the reticuloendothelial system, yttrium iodide can cause acute hepatic injury and a transient increase of plasma calcium . When used as a precursor in the synthesis of superconducting materials, yttrium iodide contributes to the formation of YBCO superconductors .
Action Environment
The action of yttrium iodide can be influenced by environmental factors. For instance, the solubility of yttrium iodide in water and ethanol suggests that its action may be influenced by the presence of these solvents. Furthermore, the crystal structure of yttrium iodide can undergo transformations in a confined, solvent-free environment .
Vorbereitungsmethoden
Yttrium iodide can be synthesized through several methods:
Heating yttrium and iodine in an inert atmosphere: This method involves directly combining yttrium metal with iodine gas under an inert atmosphere to form yttrium iodide.
Heating yttrium oxide with ammonium iodide: Yttrium oxide reacts with ammonium iodide at elevated temperatures to produce yttrium iodide.
Reacting yttrium oxide or yttrium hydroxide with hydroiodic acid: This method involves dissolving yttrium oxide or yttrium hydroxide in hydroiodic acid to form yttrium iodide.
Analyse Chemischer Reaktionen
Yttrium iodide undergoes various chemical reactions, including:
Oxidation: Yttrium iodide can be oxidized to form yttrium oxide (Y₂O₃) when exposed to oxygen or other oxidizing agents.
Common reagents and conditions used in these reactions include oxygen, reducing agents like hydrogen gas, and halide salts. The major products formed from these reactions are yttrium oxide, yttrium metal, and various yttrium halides .
Vergleich Mit ähnlichen Verbindungen
Yttrium iodide can be compared with other yttrium halides, such as yttrium fluoride (YF₃), yttrium chloride (YCl₃), and yttrium bromide (YBr₃). These compounds share similar properties, such as solubility in water and formation of colorless crystals . yttrium iodide is unique due to its specific applications in superconductors and radiopharmaceuticals .
Similar compounds include:
- Yttrium fluoride (YF₃)
- Yttrium chloride (YCl₃)
- Yttrium bromide (YBr₃)
These compounds are also used in various industrial and scientific applications, but yttrium iodide stands out for its role in high-temperature superconductors and cancer treatment .
Eigenschaften
CAS-Nummer |
13470-38-7 |
---|---|
Molekularformel |
I3Y |
Molekulargewicht |
469.6193 g/mol |
IUPAC-Name |
yttrium(3+);triiodide |
InChI |
InChI=1S/3HI.Y/h3*1H;/q;;;+3/p-3 |
InChI-Schlüssel |
LFWQXIMAKJCMJL-UHFFFAOYSA-K |
SMILES |
[Y](I)(I)I |
Kanonische SMILES |
[Y+3].[I-].[I-].[I-] |
Key on ui other cas no. |
13470-38-7 |
Herkunft des Produkts |
United States |
Haftungsausschluss und Informationen zu In-Vitro-Forschungsprodukten
Bitte beachten Sie, dass alle Artikel und Produktinformationen, die auf BenchChem präsentiert werden, ausschließlich zu Informationszwecken bestimmt sind. Die auf BenchChem zum Kauf angebotenen Produkte sind speziell für In-vitro-Studien konzipiert, die außerhalb lebender Organismen durchgeführt werden. In-vitro-Studien, abgeleitet von dem lateinischen Begriff "in Glas", beinhalten Experimente, die in kontrollierten Laborumgebungen unter Verwendung von Zellen oder Geweben durchgeführt werden. Es ist wichtig zu beachten, dass diese Produkte nicht als Arzneimittel oder Medikamente eingestuft sind und keine Zulassung der FDA für die Vorbeugung, Behandlung oder Heilung von medizinischen Zuständen, Beschwerden oder Krankheiten erhalten haben. Wir müssen betonen, dass jede Form der körperlichen Einführung dieser Produkte in Menschen oder Tiere gesetzlich strikt untersagt ist. Es ist unerlässlich, sich an diese Richtlinien zu halten, um die Einhaltung rechtlicher und ethischer Standards in Forschung und Experiment zu gewährleisten.