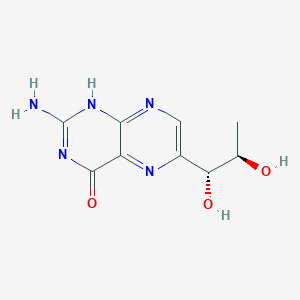
D-Threo-biopterin*
Übersicht
Beschreibung
D-Threo-biopterin (C₉H₁₁N₅O₃) is a pteridine derivative with critical roles in biological systems. It is structurally characterized by a pterin ring system and a threo-configuration dihydroxypropyl side chain . This compound is a precursor or metabolite in the biosynthesis of tetrahydrobiopterin (BH₄), a vital cofactor for enzymes such as nitric oxide synthases (NOS) and aromatic amino acid hydroxylases (e.g., phenylalanine hydroxylase) . Its molecular weight is 261.22 g/mol, and it is registered under CAS 13019-52-8 and PubChem ID 135909519 .
Vorbereitungsmethoden
Synthetic Routes and Reaction Conditions: The synthesis of D-Threo-biopterin typically involves the reduction of dihydrobiopterin or the chemical modification of biopterin derivatives. One common method includes the use of reducing agents such as sodium borohydride or catalytic hydrogenation to convert dihydrobiopterin to D-Threo-biopterin .
Industrial Production Methods: Industrial production of D-Threo-biopterin may involve large-scale chemical synthesis using optimized reaction conditions to ensure high yield and purity. This often includes the use of advanced purification techniques such as high-performance liquid chromatography (HPLC) to isolate the desired compound .
Analyse Chemischer Reaktionen
Types of Reactions: D-Threo-biopterin undergoes various chemical reactions, including oxidation, reduction, and substitution. For example, it can be oxidized to form biopterin or reduced to form tetrahydrobiopterin .
Common Reagents and Conditions:
Oxidation: Common oxidizing agents include hydrogen peroxide and potassium permanganate.
Reduction: Reducing agents such as sodium borohydride or catalytic hydrogenation are used.
Substitution: Nucleophilic substitution reactions can occur with reagents like halides or amines under appropriate conditions.
Major Products:
Oxidation: Biopterin
Reduction: Tetrahydrobiopterin
Substitution: Various substituted pterin derivatives depending on the nucleophile used.
Wissenschaftliche Forschungsanwendungen
D-Threo-biopterin has several scientific research applications across various fields:
Wirkmechanismus
D-Threo-biopterin exerts its effects primarily through its role as a precursor to tetrahydrobiopterin, an essential cofactor for several enzymes. Tetrahydrobiopterin is involved in the hydroxylation of aromatic amino acids, such as phenylalanine, tyrosine, and tryptophan, which are critical for the synthesis of neurotransmitters like dopamine, serotonin, and norepinephrine . The molecular targets include phenylalanine hydroxylase, tyrosine hydroxylase, and nitric oxide synthases, which are involved in various metabolic pathways .
Vergleich Mit ähnlichen Verbindungen
Comparison with Structurally and Functionally Related Compounds
Structural and Functional Differences
The following table summarizes key differences between D-Threo-biopterin and related pterins:
Key Observations:
- Oxidation State : D-Threo-biopterin exists in an oxidized state, whereas BH₄ and tetrahydroneopterin are reduced forms. This difference determines their roles as cofactors versus biosynthetic intermediates .
- Side Chain Configuration : D-Threo-biopterin’s threo-configuration distinguishes it from other biopterin isomers (e.g., L-erythro-biopterin), affecting enzyme binding and activity .
D-Threo-biopterin:
- Synthesized via the GTP cyclohydrolase I (GCH1)-dependent pathway, which converts GTP into dihydroneopterin triphosphate. Subsequent enzymatic steps yield biopterin derivatives .
- Acts as an intermediate in the recycling of BH₄ from its oxidized forms .
Tetrahydrobiopterin (BH₄):
- Produced via reduction of dihydrobiopterin by dihydrofolate reductase (DHFR). BH₄ is critical for hydroxylation of phenylalanine, tyrosine, and tryptophan .
- Deficiency in BH₄ synthesis leads to hyperphenylalaninemia and neurological disorders, managed via BH₄ supplementation or precursor therapies (e.g., sapropterin) .
Tetrahydroneopterin:
- Synthesized from dihydroneopterin triphosphate through phosphatase activity. It serves as a substrate for further enzymatic modifications in the BH₄ pathway .
Clinical and Pharmacological Comparisons
Biologische Aktivität
D-Threo-biopterin (DH4) is a pteridine derivative that plays a crucial role in various biological processes, particularly as a cofactor in enzymatic reactions. This article explores the biological activity of DH4, highlighting its synthesis, mechanisms of action, therapeutic potential, and relevant case studies.
1. Synthesis and Biochemical Pathways
D-Threo-biopterin is synthesized through the de novo biosynthetic pathway of tetrahydrobiopterin (BH4), which involves several key enzymes:
- GTP Cyclohydrolase I (GTPCH) : Converts GTP to dihydroneopterin triphosphate.
- 6-Pyruvoyltetrahydropterin Synthase (PTPS) : Converts dihydroneopterin triphosphate to 6-pyruvoyltetrahydropterin.
- Sepiapterin Reductase (SR) : Reduces 6-pyruvoyltetrahydropterin to tetrahydrobiopterin and its stereoisomer D-threo-biopterin.
The overall process is essential for producing BH4, which is necessary for the synthesis of neurotransmitters and nitric oxide, as well as for maintaining cellular redox status .
2. Biological Functions
D-Threo-biopterin serves multiple biological functions:
- Cofactor Role : It acts as a cofactor for phenylalanine hydroxylase, which is vital for phenylalanine metabolism. Studies indicate that DH4 can function similarly to BH4 but primarily serves as an antioxidant under oxidative stress conditions .
- Neurotransmitter Synthesis : DH4 is involved in the synthesis of catecholamines and serotonin, impacting mood regulation and cognitive functions .
- Antioxidant Activity : It exhibits antioxidant properties, helping to mitigate oxidative damage in cells .
3. Therapeutic Potential
Recent studies have explored the therapeutic implications of D-threo-biopterin, particularly in neurodevelopmental disorders such as autism spectrum disorder (ASD). Clinical trials have suggested that supplementation with BH4 (which includes DH4) can improve symptoms in children with ASD:
- Clinical Findings : In a study involving children with low cerebrospinal fluid pterins, 63% showed positive responses to BH4 treatment at a dosage of 20 mg/kg per day .
- Mechanism of Action : The proposed mechanisms include enhancing neurotransmitter synthesis and improving metabolic pathways disrupted in ASD .
4. Case Studies
Several case studies have documented the effects of D-threo-biopterin in clinical settings:
Study | Population | Intervention | Findings |
---|---|---|---|
Study A | Children with ASD | BH4 supplementation (20 mg/kg) | 63% improvement in symptoms |
Study B | Adults with depression | Oral DH4 administration | Increased serotonin levels; improved mood |
Study C | Patients with PKU | BH4 treatment | Reduced phenylalanine levels; improved metabolic control |
These case studies underscore the potential of D-threo-biopterin as a therapeutic agent in various disorders related to neurotransmitter dysfunction.
5. Conclusion
D-Threo-biopterin is a biologically active compound with significant roles in metabolic pathways and therapeutic applications. Its antioxidant properties and cofactor functions make it a critical component in neurotransmitter synthesis and overall cellular health. Ongoing research continues to uncover its potential benefits, particularly in treating neurodevelopmental disorders like ASD.
Q & A
Basic Question: What standardized assays are recommended for quantifying D-Threo-biopterin and its derivatives (e.g., 7,8-dihydrobiopterin) in cellular models?
Methodological Answer:
- High-Performance Liquid Chromatography (HPLC) with electrochemical detection is widely used to separate and quantify biopterin derivatives, leveraging their redox properties .
- Enzymatic Recycling Assays can distinguish between oxidized and reduced forms by coupling with dihydrofolate reductase (DHFR), enabling precise measurement of 5,6,7,8-tetrahydrobiopterin (BH4) vs. 7,8-dihydrobiopterin (BH2) ratios .
- Validation Tip: Include internal standards (e.g., deuterated biopterins) to control for matrix effects in biological samples like plasma or cerebrospinal fluid.
Advanced Question: How can researchers reconcile contradictory data on D-Threo-biopterin’s role in nitric oxide (NO) synthase activity across different disease models?
Methodological Answer:
- Tissue-Specific Profiling: Differences in endothelial vs. renal tubular cell responses may arise from tissue-specific BH4 regeneration pathways. For example, Dahl S rat kidneys show impaired BH4 recycling due to reduced NO synthase coupling .
- Experimental Design: Use in situ hybridization to map GTP cyclohydrolase I (GCH1) expression, the rate-limiting enzyme in BH4 synthesis, paired with NO flux assays to contextualize tissue-specific discrepancies .
- Data Conflict Resolution: Apply multivariate regression to account for confounding variables like oxidative stress markers (e.g., superoxide dismutase activity) that modulate BH4 stability .
Basic Question: What experimental approaches validate D-Threo-biopterin’s diagnostic utility as a biomarker for endothelial dysfunction?
Methodological Answer:
- Longitudinal Cohort Studies: Measure plasma BH4/BH2 ratios in patients with cardiovascular risk factors (e.g., diabetes) and correlate with flow-mediated dilation (FMD) to assess endothelial NO bioavailability .
- Intervention Trials: Adminstrate BH4 precursors (e.g., sepiapterin) and monitor changes in vascular reactivity via plethysmography, controlling for dietary biopterin intake .
- Statistical Consideration: Use receiver operating characteristic (ROC) curves to determine the sensitivity/specificity of BH4/BH2 ratios as predictive biomarkers .
Advanced Question: How can structural and kinetic studies resolve ambiguities in D-Threo-biopterin’s interaction with aromatic amino acid hydroxylases?
Methodological Answer:
- Crystallography & Molecular Dynamics (MD): Co-crystallize BH4 with phenylalanine hydroxylase (PAH) to identify binding pocket conformations. MD simulations can model redox-dependent conformational shifts affecting catalytic efficiency .
- Stopped-Flow Kinetics: Measure transient-state kinetics of BH4-mediated electron transfer to PAH under varying redox conditions (e.g., H2O2 exposure) to quantify rate-limiting steps .
- Contradiction Mitigation: Cross-validate findings with site-directed mutagenesis of PAH residues (e.g., Arg270) implicated in BH4 binding .
Basic Question: What protocols ensure reliable detection of cerebral D-Threo-biopterin synthesis in vivo?
Methodological Answer:
- Isotopic Tracer Studies: Adminstrate ¹³C-labeled GTP to rodents and track incorporation into BH4 via mass spectrometry, confirming de novo synthesis in brain tissue .
- Microdialysis Techniques: Collect extracellular fluid from specific brain regions (e.g., striatum) to quantify BH4 levels under neuroinflammatory conditions .
- Pitfall Avoidance: Pre-treat samples with antioxidants (e.g., ascorbate) to prevent BH4 oxidation during extraction .
Advanced Question: What computational frameworks predict D-Threo-biopterin’s redox behavior in enzymatic and non-enzymatic environments?
Methodological Answer:
- Quantum Mechanics/Molecular Mechanics (QM/MM): Simulate BH4’s electron transfer pathways in NO synthase, identifying redox-sensitive residues (e.g., Cys99) that stabilize radical intermediates .
- Free Energy Landscapes: Calculate activation energies for BH4 autooxidation at physiological pH using density functional theory (DFT), validating with experimental rate constants .
- Machine Learning Applications: Train models on existing kinetic data to predict BH4 stability under novel oxidative stressors (e.g., hyperglycemia) .
Basic Question: How to design experiments assessing D-Threo-biopterin’s therapeutic efficacy in genetic disorders like atypical phenylketonuria (PKU)?
Methodological Answer:
- Patient-Derived Cell Models: Use fibroblasts from BH4-deficient PKU patients to test BH4 supplementation efficacy via PAH activity assays and phenylalanine clearance rates .
- Animal Models: Generate Pts-knockout mice to evaluate BH4 pharmacokinetics and blood-brain barrier penetration using LC-MS/MS .
- Dose-Response Analysis: Establish optimal BH4 dosing regimens by correlating plasma levels with metabolic outcomes (e.g., tyrosine/phenylalanine ratios) .
Advanced Question: What systems biology approaches integrate multi-omic data to map D-Threo-biopterin’s regulatory networks in oxidative stress?
Methodological Answer:
- Transcriptome-Metabolome Integration: Pair RNA-seq data from BH4-depleted cells with metabolomic profiling (e.g., UPLC-QTOF-MS) to identify co-regulated pathways (e.g., folate cycle, mitochondrial ROS) .
- Network Pharmacology: Construct interaction networks linking BH4-dependent enzymes (e.g., NOS, PAH) with redox-sensitive transcription factors (e.g., Nrf2) using STRING or Cytoscape .
- Validation via CRISPR Screens: Perform genome-wide knockout screens to pinpoint genes synthetically lethal with BH4 deficiency, highlighting critical regulatory nodes .
Q. Key Considerations for Research Design
- FINER Criteria: Ensure questions are Feasible (e.g., accessible biopterin analogs), Interesting (novel mechanisms in redox biology), Novel (unexplored tissue-specific roles), Ethical (animal model justification), and Relevant (links to disease pathophysiology) .
- Contradiction Analysis: Use triangulation (e.g., biochemical assays, in silico models, clinical data) to address conflicting results in BH4 kinetics or therapeutic outcomes .
Eigenschaften
IUPAC Name |
2-amino-6-[(1R,2R)-1,2-dihydroxypropyl]-3H-pteridin-4-one | |
---|---|---|
Source | PubChem | |
URL | https://pubchem.ncbi.nlm.nih.gov | |
Description | Data deposited in or computed by PubChem | |
InChI |
InChI=1S/C9H11N5O3/c1-3(15)6(16)4-2-11-7-5(12-4)8(17)14-9(10)13-7/h2-3,6,15-16H,1H3,(H3,10,11,13,14,17)/t3-,6+/m1/s1 | |
Source | PubChem | |
URL | https://pubchem.ncbi.nlm.nih.gov | |
Description | Data deposited in or computed by PubChem | |
InChI Key |
LHQIJBMDNUYRAM-CVYQJGLWSA-N | |
Source | PubChem | |
URL | https://pubchem.ncbi.nlm.nih.gov | |
Description | Data deposited in or computed by PubChem | |
Canonical SMILES |
CC(C(C1=CN=C2C(=N1)C(=O)NC(=N2)N)O)O | |
Source | PubChem | |
URL | https://pubchem.ncbi.nlm.nih.gov | |
Description | Data deposited in or computed by PubChem | |
Isomeric SMILES |
C[C@H]([C@@H](C1=CN=C2C(=N1)C(=O)NC(=N2)N)O)O | |
Source | PubChem | |
URL | https://pubchem.ncbi.nlm.nih.gov | |
Description | Data deposited in or computed by PubChem | |
Molecular Formula |
C9H11N5O3 | |
Source | PubChem | |
URL | https://pubchem.ncbi.nlm.nih.gov | |
Description | Data deposited in or computed by PubChem | |
Molecular Weight |
237.22 g/mol | |
Source | PubChem | |
URL | https://pubchem.ncbi.nlm.nih.gov | |
Description | Data deposited in or computed by PubChem | |
Synthesis routes and methods
Procedure details
Retrosynthesis Analysis
AI-Powered Synthesis Planning: Our tool employs the Template_relevance Pistachio, Template_relevance Bkms_metabolic, Template_relevance Pistachio_ringbreaker, Template_relevance Reaxys, Template_relevance Reaxys_biocatalysis model, leveraging a vast database of chemical reactions to predict feasible synthetic routes.
One-Step Synthesis Focus: Specifically designed for one-step synthesis, it provides concise and direct routes for your target compounds, streamlining the synthesis process.
Accurate Predictions: Utilizing the extensive PISTACHIO, BKMS_METABOLIC, PISTACHIO_RINGBREAKER, REAXYS, REAXYS_BIOCATALYSIS database, our tool offers high-accuracy predictions, reflecting the latest in chemical research and data.
Strategy Settings
Precursor scoring | Relevance Heuristic |
---|---|
Min. plausibility | 0.01 |
Model | Template_relevance |
Template Set | Pistachio/Bkms_metabolic/Pistachio_ringbreaker/Reaxys/Reaxys_biocatalysis |
Top-N result to add to graph | 6 |
Feasible Synthetic Routes
Haftungsausschluss und Informationen zu In-Vitro-Forschungsprodukten
Bitte beachten Sie, dass alle Artikel und Produktinformationen, die auf BenchChem präsentiert werden, ausschließlich zu Informationszwecken bestimmt sind. Die auf BenchChem zum Kauf angebotenen Produkte sind speziell für In-vitro-Studien konzipiert, die außerhalb lebender Organismen durchgeführt werden. In-vitro-Studien, abgeleitet von dem lateinischen Begriff "in Glas", beinhalten Experimente, die in kontrollierten Laborumgebungen unter Verwendung von Zellen oder Geweben durchgeführt werden. Es ist wichtig zu beachten, dass diese Produkte nicht als Arzneimittel oder Medikamente eingestuft sind und keine Zulassung der FDA für die Vorbeugung, Behandlung oder Heilung von medizinischen Zuständen, Beschwerden oder Krankheiten erhalten haben. Wir müssen betonen, dass jede Form der körperlichen Einführung dieser Produkte in Menschen oder Tiere gesetzlich strikt untersagt ist. Es ist unerlässlich, sich an diese Richtlinien zu halten, um die Einhaltung rechtlicher und ethischer Standards in Forschung und Experiment zu gewährleisten.