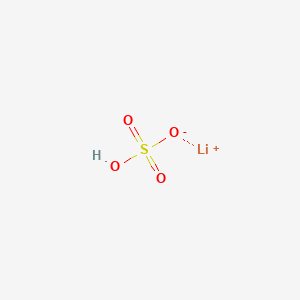
Lithium hydrogen sulfate
Übersicht
Beschreibung
Lithium hydrogen sulfate is a chemical compound with the formula LiHSO₄. It belongs to the family of hydrogen-bonded compounds with a general formula MHXO₄, where M can be ammonium, rubidium, cesium, lithium, or potassium, and X can be sulfur or selenium . This compound is known for its high-temperature phase transition and proton conductivity, making it of significant interest for various scientific and industrial applications .
Vorbereitungsmethoden
Synthetic Routes and Reaction Conditions
Lithium hydrogen sulfate can be synthesized through the reaction of lithium carbonate with sulfuric acid. The reaction involves adding lithium carbonate to a reactor and slowly adding sulfuric acid under stirring conditions. The resulting solution is then evaporated and concentrated, followed by cooling and crystallization to obtain lithium sulfate monohydrate, which is then dried to produce this compound .
Industrial Production Methods
In industrial settings, this compound is typically produced using similar methods but on a larger scale. The process involves careful control of reaction conditions to ensure high purity and yield. The use of advanced techniques such as continuous flow reactors and automated crystallization systems can enhance the efficiency and scalability of the production process .
Analyse Chemischer Reaktionen
Types of Reactions
Lithium hydrogen sulfate undergoes various chemical reactions, including:
Oxidation and Reduction: It can be reduced to lithium sulfide using hydrogen gas, a process that is both green and cost-effective.
Substitution: It can participate in metathesis reactions with other lithium salts and sodium sulfide to produce lithium sulfide.
Common Reagents and Conditions
Oxidation and Reduction: Hydrogen gas is commonly used as a reducing agent under controlled temperature and pressure conditions.
Substitution: Lithium salts (such as lithium halides and nitrates) and sodium sulfide are used in metathesis reactions.
Major Products Formed
Wissenschaftliche Forschungsanwendungen
Lithium hydrogen sulfate has several scientific research applications, including:
Wirkmechanismus
The mechanism of action of lithium hydrogen sulfate involves its ability to participate in proton conduction and phase transitions. The compound exhibits a first-order phase transition accompanied by a significant increase in conductivity, primarily due to protonic diffusion . This proton conduction is crucial for its applications in fuel cells and energy storage systems .
Vergleich Mit ähnlichen Verbindungen
Lithium hydrogen sulfate belongs to a family of hydrogen-bonded compounds with similar properties. Some of the similar compounds include:
- Ammonium hydrogen sulfate (NH₄HSO₄)
- Rubidium hydrogen sulfate (RbHSO₄)
- Cesium hydrogen sulfate (CsHSO₄)
- Potassium hydrogen sulfate (KHSO₄)
Uniqueness
What sets this compound apart from these similar compounds is its high-temperature phase transition and superior proton conductivity. These properties make it particularly suitable for applications in high-temperature environments and advanced energy storage systems .
Biologische Aktivität
Lithium hydrogen sulfate (LiHSO4) is a compound that has garnered attention for its potential biological activities, particularly in the context of lithium's known therapeutic effects in psychiatric disorders. This article explores the biological activity of LiHSO4, its mechanisms of action, and relevant research findings.
This compound is synthesized through various methods, including the slow evaporation technique, which allows for the growth of single crystals. The compound has distinct properties that make it suitable for various applications, including its use in batteries and as a potential therapeutic agent .
Lithium compounds, including LiHSO4, exhibit biological activity primarily through their interaction with cellular signaling pathways. One notable mechanism involves the inhibition of glycogen synthase kinase 3 beta (GSK3B), an enzyme that plays a crucial role in various cellular processes, including apoptosis, inflammation, and neuroprotection. Lithium has been shown to compete with magnesium for binding sites on GSK3B, leading to altered phosphorylation states of numerous substrates involved in critical signaling pathways .
Table 1: Key Biological Effects of Lithium Compounds
Case Studies and Research Findings
Several studies have investigated the biological effects of lithium compounds, including LiHSO4. Notably:
- Neuroprotective Effects : Research indicates that lithium can protect neurons from apoptosis by modulating GSK3B activity. This action is significant in the context of neurodegenerative diseases where apoptosis plays a critical role .
- Gene Expression Modulation : Lithium treatment has been shown to significantly alter gene expression profiles in brain cells, suggesting its role in neurodevelopment and plasticity .
- Therapeutic Applications : Clinical studies have demonstrated that lithium salts can effectively treat bipolar disorder and depression. The mechanisms involve complex interactions with neurotransmitter systems and neurohormonal pathways .
Eigenschaften
IUPAC Name |
lithium;hydrogen sulfate | |
---|---|---|
Source | PubChem | |
URL | https://pubchem.ncbi.nlm.nih.gov | |
Description | Data deposited in or computed by PubChem | |
InChI |
InChI=1S/Li.H2O4S/c;1-5(2,3)4/h;(H2,1,2,3,4)/q+1;/p-1 | |
Source | PubChem | |
URL | https://pubchem.ncbi.nlm.nih.gov | |
Description | Data deposited in or computed by PubChem | |
InChI Key |
HPCCWDVOHHFCKM-UHFFFAOYSA-M | |
Source | PubChem | |
URL | https://pubchem.ncbi.nlm.nih.gov | |
Description | Data deposited in or computed by PubChem | |
Canonical SMILES |
[Li+].OS(=O)(=O)[O-] | |
Source | PubChem | |
URL | https://pubchem.ncbi.nlm.nih.gov | |
Description | Data deposited in or computed by PubChem | |
Molecular Formula |
LiHSO4, HLiO4S | |
Record name | lithium hydrogen sulfate | |
Source | Wikipedia | |
URL | https://en.wikipedia.org/wiki/Dictionary_of_chemical_formulas | |
Description | Chemical information link to Wikipedia. | |
Source | PubChem | |
URL | https://pubchem.ncbi.nlm.nih.gov | |
Description | Data deposited in or computed by PubChem | |
DSSTOX Substance ID |
DTXSID90928663 | |
Record name | Lithium hydrogen sulfate | |
Source | EPA DSSTox | |
URL | https://comptox.epa.gov/dashboard/DTXSID90928663 | |
Description | DSSTox provides a high quality public chemistry resource for supporting improved predictive toxicology. | |
Molecular Weight |
104.0 g/mol | |
Source | PubChem | |
URL | https://pubchem.ncbi.nlm.nih.gov | |
Description | Data deposited in or computed by PubChem | |
CAS No. |
13453-86-6 | |
Record name | Lithium hydrogen sulphate | |
Source | ChemIDplus | |
URL | https://pubchem.ncbi.nlm.nih.gov/substance/?source=chemidplus&sourceid=0013453866 | |
Description | ChemIDplus is a free, web search system that provides access to the structure and nomenclature authority files used for the identification of chemical substances cited in National Library of Medicine (NLM) databases, including the TOXNET system. | |
Record name | Lithium hydrogen sulfate | |
Source | EPA DSSTox | |
URL | https://comptox.epa.gov/dashboard/DTXSID90928663 | |
Description | DSSTox provides a high quality public chemistry resource for supporting improved predictive toxicology. | |
Record name | Lithium hydrogen sulphate | |
Source | European Chemicals Agency (ECHA) | |
URL | https://echa.europa.eu/substance-information/-/substanceinfo/100.033.291 | |
Description | The European Chemicals Agency (ECHA) is an agency of the European Union which is the driving force among regulatory authorities in implementing the EU's groundbreaking chemicals legislation for the benefit of human health and the environment as well as for innovation and competitiveness. | |
Explanation | Use of the information, documents and data from the ECHA website is subject to the terms and conditions of this Legal Notice, and subject to other binding limitations provided for under applicable law, the information, documents and data made available on the ECHA website may be reproduced, distributed and/or used, totally or in part, for non-commercial purposes provided that ECHA is acknowledged as the source: "Source: European Chemicals Agency, http://echa.europa.eu/". Such acknowledgement must be included in each copy of the material. ECHA permits and encourages organisations and individuals to create links to the ECHA website under the following cumulative conditions: Links can only be made to webpages that provide a link to the Legal Notice page. | |
Haftungsausschluss und Informationen zu In-Vitro-Forschungsprodukten
Bitte beachten Sie, dass alle Artikel und Produktinformationen, die auf BenchChem präsentiert werden, ausschließlich zu Informationszwecken bestimmt sind. Die auf BenchChem zum Kauf angebotenen Produkte sind speziell für In-vitro-Studien konzipiert, die außerhalb lebender Organismen durchgeführt werden. In-vitro-Studien, abgeleitet von dem lateinischen Begriff "in Glas", beinhalten Experimente, die in kontrollierten Laborumgebungen unter Verwendung von Zellen oder Geweben durchgeführt werden. Es ist wichtig zu beachten, dass diese Produkte nicht als Arzneimittel oder Medikamente eingestuft sind und keine Zulassung der FDA für die Vorbeugung, Behandlung oder Heilung von medizinischen Zuständen, Beschwerden oder Krankheiten erhalten haben. Wir müssen betonen, dass jede Form der körperlichen Einführung dieser Produkte in Menschen oder Tiere gesetzlich strikt untersagt ist. Es ist unerlässlich, sich an diese Richtlinien zu halten, um die Einhaltung rechtlicher und ethischer Standards in Forschung und Experiment zu gewährleisten.