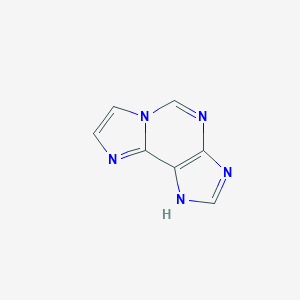
1,N6-Ethenoadenine
Übersicht
Beschreibung
1,N6-Ethenoadenine (εA) is a tricyclic derivative of adenine. An ethylene bridge connects the amine group to the adjacent carbon on the six-member ring, adding another five-membered ring . This modification can occur as a mutation of DNA, and as a DNA modification, it is called an etheno (ε) DNA adduct .
Synthesis Analysis
1,N6-Ethenoadenine can be formed by the reaction of adenine with vinyl chloride or lipid peroxidation products . It can also be produced via the cyclisation of N6-hydroxyethyladenine with DIAD and triphenylphosphine in the Mitsunobu reaction in a THF/MeCN solvent or by reacting with SOCl2 .Molecular Structure Analysis
The molecular structure of 1,N6-Ethenoadenine involves an ethylene bridge that connects the amine group to the adjacent carbon on the six-member ring, forming an additional five-membered ring . This structure is a tricyclic derivative of adenine .Chemical Reactions Analysis
The study of the photoenzymes of 1,N6-Ethenoadenine has been facilitated using flavin analogs. The additional electron density on the etheno group of ε FAD supplies better orbital overlap with the flavin S1 state, accelerating charge transfer in that molecule .Wissenschaftliche Forschungsanwendungen
DNA Repair Mechanisms
1,N6-Ethenoadenine (εA) plays a significant role in the study of DNA repair mechanisms . It is particularly useful in understanding the repair aspect of εA by the 2-oxoglutarate/Fe(II)-dependent AlkB family enzymes . Researchers have investigated εA repair across 16 possible sequence contexts, revealing that repair efficiency is altered according to sequence, enzyme, and strand context .
Study of Mutational Spectra
The mutation patterns of DNA adducts, such as mutational spectra and signatures, are useful tools for diagnostic and prognostic purposes . The study of εA contributes to understanding these mutation patterns, which derive from three sources: adduct formation, replication bypass, and repair .
Photoenzymatic Cofactor
Flavin 1,N6-Ethenoadenine Dinucleotide (εFAD), an analog of FAD, is used to study how different photochemical outcomes depend on the identity of the Ade moiety in stacked FAD and its analog εFAD . This research contributes to understanding the excited state evolution of the flavins .
Study of Photoinduced Redox Chemistry
Flavins, including εFAD, often exploit the absorption of light to energize photoinduced redox chemistry in a variety of contexts . The study of these photoenzymes has been facilitated using flavin analogs, including εFAD .
ADP-Ribosylation Reactions
Nicotinamide 1,N6-Ethenoadenine dinucleotide (ε-NAD) is an NAD fluorescent analog that may be used to study ADP-ribosylation reactions . This research contributes to understanding the structure and kinetics of NAD+ binding enzymes .
Enzyme Turnover
There is recent evidence that adenine (Ade)-modified FAD can affect enzyme turnover . This has been shown for enzymes where the adenine and flavin rings are close to each other in a stacked conformation .
Wirkmechanismus
Target of Action
The primary target of 1,N6-Ethenoadenine (εA) is the Endonuclease III . This enzyme plays a crucial role in DNA repair mechanisms, particularly in the base excision repair (BER) pathway .
Mode of Action
1,N6-Ethenoadenine (εA) is a tricyclic derivative of adenine, where an ethylene bridge connects the amine group to the adjacent carbon on the six-member ring, forming an additional five-membered ring . This modification can occur as a mutation of DNA, where it is referred to as an etheno (ε) DNA adduct . The εA lesion interacts with its target, Endonuclease III, which recognizes and binds to the εA lesion, initiating the repair process .
Biochemical Pathways
The primary biochemical pathway affected by εA is the base excision repair (BER) pathway . This pathway is responsible for repairing small, non-helix-distorting base lesions from the genome. The process involves the removal and replacement of the modified nucleobase through cleavage of the glycosidic bond by a glycosylase, followed by filling . Another pathway involved in the repair of εA is the direct reversal repair (DRR) pathway .
Result of Action
The εA lesion is mutagenic, leading to primarily A→T transversions as well as A→G and A→C mutations . These εA-induced mutations have been associated with p53 mutation hotspots, cancerous tissues, and inflammatory diseases . The biological consequences of εa lesions can be minimized through the action of dna repair mechanisms, specifically the ber and drr pathways .
Action Environment
The action, efficacy, and stability of 1,N6-Ethenoadenine are influenced by various environmental factors. For instance, eukaryotic DNA is packaged in chromatin, which impacts the BER and DRR pathways, specifically in regards to the repair of εA . Furthermore, the formation of εA can be induced by exogenous sources such as vinyl chloride or endogenous sources like lipid peroxidation products .
Eigenschaften
IUPAC Name |
1H-imidazo[2,1-f]purine | |
---|---|---|
Source | PubChem | |
URL | https://pubchem.ncbi.nlm.nih.gov | |
Description | Data deposited in or computed by PubChem | |
InChI |
InChI=1S/C7H5N5/c1-2-12-4-11-6-5(7(12)8-1)9-3-10-6/h1-4H,(H,9,10) | |
Source | PubChem | |
URL | https://pubchem.ncbi.nlm.nih.gov | |
Description | Data deposited in or computed by PubChem | |
InChI Key |
OGVOXGPIHFKUGM-UHFFFAOYSA-N | |
Source | PubChem | |
URL | https://pubchem.ncbi.nlm.nih.gov | |
Description | Data deposited in or computed by PubChem | |
Canonical SMILES |
C1=CN2C=NC3=C(C2=N1)NC=N3 | |
Source | PubChem | |
URL | https://pubchem.ncbi.nlm.nih.gov | |
Description | Data deposited in or computed by PubChem | |
Molecular Formula |
C7H5N5 | |
Source | PubChem | |
URL | https://pubchem.ncbi.nlm.nih.gov | |
Description | Data deposited in or computed by PubChem | |
DSSTOX Substance ID |
DTXSID90160768 | |
Record name | 1,N(6)-Ethenoadenine | |
Source | EPA DSSTox | |
URL | https://comptox.epa.gov/dashboard/DTXSID90160768 | |
Description | DSSTox provides a high quality public chemistry resource for supporting improved predictive toxicology. | |
Molecular Weight |
159.15 g/mol | |
Source | PubChem | |
URL | https://pubchem.ncbi.nlm.nih.gov | |
Description | Data deposited in or computed by PubChem | |
Product Name |
1,N6-Ethenoadenine | |
CAS RN |
13875-63-3 | |
Record name | Ethenoadenine | |
Source | CAS Common Chemistry | |
URL | https://commonchemistry.cas.org/detail?cas_rn=13875-63-3 | |
Description | CAS Common Chemistry is an open community resource for accessing chemical information. Nearly 500,000 chemical substances from CAS REGISTRY cover areas of community interest, including common and frequently regulated chemicals, and those relevant to high school and undergraduate chemistry classes. This chemical information, curated by our expert scientists, is provided in alignment with our mission as a division of the American Chemical Society. | |
Explanation | The data from CAS Common Chemistry is provided under a CC-BY-NC 4.0 license, unless otherwise stated. | |
Record name | 1,N(6)-Ethenoadenine | |
Source | ChemIDplus | |
URL | https://pubchem.ncbi.nlm.nih.gov/substance/?source=chemidplus&sourceid=0013875633 | |
Description | ChemIDplus is a free, web search system that provides access to the structure and nomenclature authority files used for the identification of chemical substances cited in National Library of Medicine (NLM) databases, including the TOXNET system. | |
Record name | 1,N6-Ethenoadenine | |
Source | DrugBank | |
URL | https://www.drugbank.ca/drugs/DB01952 | |
Description | The DrugBank database is a unique bioinformatics and cheminformatics resource that combines detailed drug (i.e. chemical, pharmacological and pharmaceutical) data with comprehensive drug target (i.e. sequence, structure, and pathway) information. | |
Explanation | Creative Common's Attribution-NonCommercial 4.0 International License (http://creativecommons.org/licenses/by-nc/4.0/legalcode) | |
Record name | 1,N(6)-Ethenoadenine | |
Source | EPA DSSTox | |
URL | https://comptox.epa.gov/dashboard/DTXSID90160768 | |
Description | DSSTox provides a high quality public chemistry resource for supporting improved predictive toxicology. | |
Record name | N6-ETHENOADENINE | |
Source | FDA Global Substance Registration System (GSRS) | |
URL | https://gsrs.ncats.nih.gov/ginas/app/beta/substances/SIN7411HG7 | |
Description | The FDA Global Substance Registration System (GSRS) enables the efficient and accurate exchange of information on what substances are in regulated products. Instead of relying on names, which vary across regulatory domains, countries, and regions, the GSRS knowledge base makes it possible for substances to be defined by standardized, scientific descriptions. | |
Explanation | Unless otherwise noted, the contents of the FDA website (www.fda.gov), both text and graphics, are not copyrighted. They are in the public domain and may be republished, reprinted and otherwise used freely by anyone without the need to obtain permission from FDA. Credit to the U.S. Food and Drug Administration as the source is appreciated but not required. | |
Retrosynthesis Analysis
AI-Powered Synthesis Planning: Our tool employs the Template_relevance Pistachio, Template_relevance Bkms_metabolic, Template_relevance Pistachio_ringbreaker, Template_relevance Reaxys, Template_relevance Reaxys_biocatalysis model, leveraging a vast database of chemical reactions to predict feasible synthetic routes.
One-Step Synthesis Focus: Specifically designed for one-step synthesis, it provides concise and direct routes for your target compounds, streamlining the synthesis process.
Accurate Predictions: Utilizing the extensive PISTACHIO, BKMS_METABOLIC, PISTACHIO_RINGBREAKER, REAXYS, REAXYS_BIOCATALYSIS database, our tool offers high-accuracy predictions, reflecting the latest in chemical research and data.
Strategy Settings
Precursor scoring | Relevance Heuristic |
---|---|
Min. plausibility | 0.01 |
Model | Template_relevance |
Template Set | Pistachio/Bkms_metabolic/Pistachio_ringbreaker/Reaxys/Reaxys_biocatalysis |
Top-N result to add to graph | 6 |
Feasible Synthetic Routes
Haftungsausschluss und Informationen zu In-Vitro-Forschungsprodukten
Bitte beachten Sie, dass alle Artikel und Produktinformationen, die auf BenchChem präsentiert werden, ausschließlich zu Informationszwecken bestimmt sind. Die auf BenchChem zum Kauf angebotenen Produkte sind speziell für In-vitro-Studien konzipiert, die außerhalb lebender Organismen durchgeführt werden. In-vitro-Studien, abgeleitet von dem lateinischen Begriff "in Glas", beinhalten Experimente, die in kontrollierten Laborumgebungen unter Verwendung von Zellen oder Geweben durchgeführt werden. Es ist wichtig zu beachten, dass diese Produkte nicht als Arzneimittel oder Medikamente eingestuft sind und keine Zulassung der FDA für die Vorbeugung, Behandlung oder Heilung von medizinischen Zuständen, Beschwerden oder Krankheiten erhalten haben. Wir müssen betonen, dass jede Form der körperlichen Einführung dieser Produkte in Menschen oder Tiere gesetzlich strikt untersagt ist. Es ist unerlässlich, sich an diese Richtlinien zu halten, um die Einhaltung rechtlicher und ethischer Standards in Forschung und Experiment zu gewährleisten.