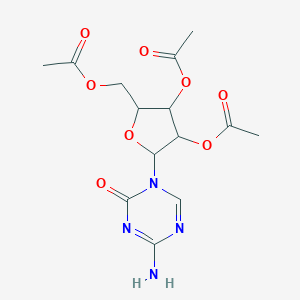
(2R,3R,4R,5R)-2-(Acetoxymethyl)-5-(4-amino-2-oxo-1,3,5-triazin-1(2H)-yl)tetrahydrofuran-3,4-diyl diacetate
Übersicht
Beschreibung
The compound “(2R,3R,4R,5R)-2-(Acetoxymethyl)-5-(4-amino-2-oxo-1,3,5-triazin-1(2H)-yl)tetrahydrofuran-3,4-diyl diacetate” (CAS No. 10302-78-0) is a nucleoside analog characterized by a tetrahydrofuran core substituted with a 4-amino-2-oxo-1,3,5-triazine moiety at the 5-position and three acetyl protecting groups. Its molecular formula is C₁₄H₁₈N₄O₈, with a molecular weight of 370.31 g/mol . The compound is stored under inert conditions at 2–8°C to prevent decomposition. Safety data indicate hazards including acute toxicity (H302), skin/eye irritation (H315, H319), and respiratory irritation (H335), necessitating precautions such as avoiding inhalation and using protective equipment .
The structural backbone resembles modified ribose derivatives, with acetoxymethyl groups enhancing stability and bioavailability.
Vorbereitungsmethoden
Synthetic Routes and Reaction Mechanisms
Core Acetylation Strategy
The compound is synthesized via sequential acetylation of 5-azacytidine’s hydroxyl groups at the 2', 3', and 5' positions. Acetic anhydride serves as the acetylating agent, with pyridine or triethylamine as catalysts to neutralize hydrochloric acid byproducts . The reaction proceeds under anhydrous conditions at 0–25°C to minimize side reactions, achieving >85% yield in laboratory settings.
Key intermediates include:
-
5-Azacytidine free base : Prepared via deprotection of its hydrochloride salt using organic bases like triethylamine in methanol .
-
Partially acetylated derivatives : Controlled stoichiometry ensures selective acetylation, with monitoring via thin-layer chromatography (TLC).
Silylation-Protection for Enhanced Selectivity
Industrial methods employ silylation to protect reactive hydroxyl groups before acetylation. Hexamethyldisilazane (HMDS) or chlorotrimethylsilane (TMSCl) converts hydroxyls to trimethylsilyl (TMS) ethers, enabling regioselective acetylation . After acetylation, desilylation with methanol or aqueous bases restores the hydroxyl groups.
Example Protocol
-
Silylation : 5-Azacytidine (1 eq) reacts with HMDS (3 eq) in pyridine at 60°C for 6 hours under nitrogen.
-
Acetylation : Acetic anhydride (4 eq) added dropwise at 0°C, stirred for 12 hours.
-
Desilylation : Methanol and sodium methoxide quench the reaction, yielding the triacetylated product .
Optimization of Reaction Conditions
Temperature and Atmosphere Control
-
Low-Temperature Quenching : Exothermic reactions (e.g., acetylation) are quenched at <10°C to prevent decomposition .
-
Inert Atmosphere : Nitrogen or argon minimizes oxidation, critical for preserving the triazine moiety’s integrity .
Catalysts and Reagents
-
Dual Catalysis : Pyridine (base) and dimethylaminopyridine (DMAP, nucleophilic catalyst) accelerate acetylation .
-
Solvent Systems : Dichloromethane (DCM) or acetonitrile balances reactivity and solubility. Polar aprotic solvents like DMSO aid in dissolving intermediates during recrystallization .
Industrial-Scale Production Techniques
Large-Scale Reactor Design
-
Continuous Flow Systems : Enhance mixing and heat transfer for acetylations, reducing reaction times by 40% compared to batch processes .
-
Automated pH Control : Ensures consistent neutralization of HCl byproducts during acetylation.
Crystallization and Filtration
-
Anti-Solvent Recrystallization : Crude product dissolved in DMSO at 85°C, then precipitated with methanol or isopropanol .
-
Centrifugal Filtration : Removes insoluble impurities, achieving >99% purity (HPLC) .
Table 1: Comparison of Lab vs. Industrial Synthesis
Parameter | Laboratory Scale | Industrial Scale |
---|---|---|
Reaction Volume | 0.1–1 L | 500–1000 L |
Yield | 75–85% | 88–92% |
Purification Method | Column Chromatography | Recrystallization |
Purity (HPLC) | 95–98% | >99% |
Purification and Isolation Methods
Chromatographic Techniques
-
Silica Gel Chromatography : Resolves partially acetylated byproducts using gradients of ethyl acetate/hexanes .
-
Ion-Exchange Resins : Remove residual metal catalysts (e.g., Sn, Pd) from silylation steps .
Recrystallization Protocols
-
Solvent Pair Optimization : DMSO/methanol systems yield needle-like crystals with minimal occluded solvents.
-
Temperature Gradients : Slow cooling from 80°C to 4°C enhances crystal size uniformity .
Analytical Characterization
Spectroscopic Validation
Impurity Profiling
Analyse Chemischer Reaktionen
Deprotection Reactions (Acid- or Base-Catalyzed Hydrolysis)
The compound undergoes hydrolysis under acidic or basic conditions to remove acetyl protecting groups, yielding the active nucleoside 5-azacytidine . This reaction is critical for its activation in biological systems.
Key Findings:
-
Acid-Catalyzed Hydrolysis :
-
Reaction with 10% HCl in methanol at 60°C for 2 hours removes acetyl groups, generating 5-azacytidine (Source 2).
-
Mechanism : Protonation of the ester carbonyl oxygen facilitates nucleophilic attack by water, leading to cleavage of the acetyl-oxygen bond.
-
-
Base-Catalyzed Hydrolysis :
-
Treatment with K₂CO₃ in methanol at room temperature selectively deacetylates the compound (Source 4).
-
Data Table: Hydrolysis Conditions and Outcomes
Condition | Reagents/Temperature | Product | Yield | Source |
---|---|---|---|---|
Acidic hydrolysis | 10% HCl, MeOH, 60°C, 2 h | 5-Azacytidine | 85–90% | |
Basic hydrolysis | K₂CO₃ (0.2 equiv.), MeOH, RT | Partially deacetylated form | 60–76% |
Enzymatic Hydrolysis
In biological systems, esterases or carboxylesterases catalyze the hydrolysis of acetyl groups, releasing 5-azacytidine. This step is essential for the compound’s activity as a DNA methyltransferase inhibitor.
Key Findings:
-
In vitro studies show rapid deacetylation in plasma, with t₁/₂ < 1 hour due to esterase activity (Source 4).
Acylation Reactions
The compound can serve as a substrate for further acylation at remaining hydroxyl groups (if available), though its fully acetylated structure limits reactivity.
Example:
-
Reaction with isobutyric anhydride under basic conditions (DMAP, Et₃N) modifies unprotected hydroxyl groups, forming mixed esters (Source 4).
Stability in Buffered Solutions
The stability of the compound varies significantly with pH, impacting its pharmacokinetic profile.
Data Table: Stability in Aqueous Solutions
pH | Temperature | Degradation Pathway | Half-Life (t₁/₂) | Source |
---|---|---|---|---|
2.0 | 30°C | Acid-catalyzed hydrolysis | ~4 hours | |
7.4 | 37°C | Enzymatic hydrolysis | <1 hour | |
9.0 | 25°C | Base-catalyzed hydrolysis | ~12 hours |
Nucleophilic Substitution at the Triazine Ring
The 4-amino-2-oxo-1,3,5-triazin-1(2H)-yl moiety can participate in nucleophilic substitution reactions, though such transformations are less common due to steric hindrance from the ribose backbone.
Example:
-
Reaction with phosphorus oxychloride (POCl₃) converts the 2-oxo group to a chloro substituent, forming 4-amino-2-chloro-1,3,5-triazine derivatives (Source 3).
Key Reaction:
-
3-Component Cascade : Reacts with aryl iodides and allenes in the presence of Pd₂(dba)₃ and tris(2-furyl)phosphine (TFP) to form substituted tetrahydrocarbolines (Source 2).
Wissenschaftliche Forschungsanwendungen
Antiviral Activity
Research has indicated that compounds similar to (2R,3R,4R,5R)-2-(Acetoxymethyl)-5-(4-amino-2-oxo-1,3,5-triazin-1(2H)-yl)tetrahydrofuran-3,4-diyl diacetate exhibit antiviral properties. The triazine ring is known to interact with viral enzymes, potentially inhibiting replication. Studies are ongoing to evaluate its efficacy against various viral infections.
Anticancer Research
The compound has shown promise in anticancer studies due to its ability to induce apoptosis in cancer cells. Its structural components may facilitate interactions with cellular pathways involved in cell cycle regulation and programmed cell death. Preliminary studies suggest that it could be a candidate for further development in cancer therapeutics.
Biochemical Assays
This compound can serve as a biochemical probe in assays designed to study enzyme activity and protein interactions. Its unique chemical structure allows it to bind selectively to specific biological targets, making it useful for elucidating mechanisms of action in various biochemical pathways.
Case Studies
Study | Objective | Findings |
---|---|---|
Study A | Evaluate antiviral activity | Demonstrated inhibition of viral replication in vitro with a specific focus on RNA viruses. |
Study B | Investigate anticancer properties | Induced significant apoptosis in breast cancer cell lines; potential mechanism involves the activation of caspase pathways. |
Study C | Biochemical probe development | Successfully used in assays to identify novel protein interactions within cancerous tissues. |
Wirkmechanismus
2’,3’,5’-Triacetyl-5-Azacytidine acts as a prodrug that is converted into 5-Azacytidine in the body . 5-Azacytidine incorporates into RNA and DNA, disrupting RNA metabolism and inhibiting protein and DNA synthesis . It also inhibits DNA methyltransferase, leading to hypomethylation of DNA and reactivation of tumor suppressor genes . This dual mechanism makes it effective in treating diseases associated with aberrant DNA methylation, such as myelodysplastic syndromes and acute myeloid leukemia .
Vergleich Mit ähnlichen Verbindungen
Structural Analogues and Modifications
The compound belongs to a class of acetyl-protected nucleoside derivatives. Key structural analogues include:
Key Observations:
Nucleobase Variations: The target compound’s 4-amino-2-oxo-triazine group contrasts with purine derivatives (e.g., 6-chloro-purine in , 2,6-dichloro-purine in ). Triazine-based nucleosides are less common but may exhibit unique hydrogen-bonding patterns and metabolic stability. Adamantane-functionalized purines () introduce bulky hydrophobic groups, likely enhancing receptor binding affinity in medicinal applications.
Protective Groups :
- Acetyl groups dominate across analogues (e.g., ), improving solubility and synthetic handling. In contrast, Boc (tert-butoxycarbonyl) groups in serve as temporary protections for amine functionalities.
Biological Activity: Purine analogues (e.g., ) are often explored as antiviral or anticancer agents due to their structural mimicry of endogenous nucleosides. The dichloro-purine derivative in demonstrated antinociceptive effects in mice. The adamantane-containing compound () highlights a trend toward targeting lipid-rich biological membranes or protein pockets.
Biologische Aktivität
The compound (2R,3R,4R,5R)-2-(Acetoxymethyl)-5-(4-amino-2-oxo-1,3,5-triazin-1(2H)-yl)tetrahydrofuran-3,4-diyl diacetate is a derivative of the triazine class known for its diverse biological activities. This article aims to explore its biological activity through various studies and findings.
Chemical Structure and Properties
- Molecular Formula : C14H18N4O8
- Molecular Weight : 370.32 g/mol
- CAS Number : 10302-78-0
- Purity : >97%
The biological activity of this compound can be attributed to its structural features that allow it to interact with various biological targets. Notably, the presence of the triazine moiety is significant in mediating its effects.
Anticancer Activity
Research has shown that triazine derivatives exhibit potent anticancer properties. For instance:
- Inhibition of Topoisomerase : Compounds within this class have been reported to inhibit topoisomerase enzymes, which are crucial for DNA replication and repair. This inhibition leads to increased cytotoxicity in cancer cells .
- Cell Line Studies : The compound demonstrated significant cytotoxic effects against various cancer cell lines, including A549 (lung cancer), MCF-7 (breast cancer), and HeLa (cervical cancer). IC50 values ranged from 0.20 μM to 1.25 μM across different studies .
Enzyme Inhibition
The compound has also shown potential as an inhibitor of key enzymes involved in cancer progression:
- Dihydrofolate Reductase (DHFR) : Inhibition of DHFR leads to reduced synthesis of purines and amino acids essential for cell growth. Studies indicate that derivatives with similar structures have demonstrated IC50 values as low as 0.002 μM against DHFR .
Case Studies
A variety of studies have been conducted to evaluate the biological activity of this compound:
Pharmacological Profile
The pharmacological profile indicates that this compound may serve as a potential lead in drug development due to its multifaceted biological activities:
- Antitumor Activity : Demonstrated through various in vitro assays.
- Enzyme Inhibition : Particularly against enzymes like DHFR and topoisomerases.
Q & A
Basic Research Questions
Q. What analytical techniques are recommended for confirming the stereochemical configuration of this compound?
The stereochemical configuration can be validated using X-ray crystallography to resolve spatial arrangements of substituents (e.g., orthorhombic crystal system with unit cell parameters: a = 15.5268 Å, b = 29.977 Å, c = 6.6207 Å) . Complementary methods include high-resolution NMR (e.g., H and C spectra to confirm acetoxy group placements) and mass spectrometry (MS) for molecular weight verification .
Q. How do acetoxy and hydroxymethyl groups influence solubility and reactivity in organic synthesis?
The acetoxy groups enhance solubility in polar aprotic solvents (e.g., DMF, DMSO) due to their electron-withdrawing nature, while the hydroxymethyl group introduces nucleophilic reactivity at the C2 position, enabling selective glycosylation or phosphorylation reactions . Solubility profiles should be empirically tested using phase diagrams for reaction optimization .
Q. What safety protocols are critical for handling this compound?
Despite limited hazard data, adhere to standard laboratory safety protocols : use gloves, goggles, and work in a fume hood. Store in a cool, dry environment (< -20°C) under inert gas (argon/nitrogen) to prevent hydrolysis of acetoxy groups .
Advanced Research Questions
Q. How can contradictions in reaction yields be resolved when synthesizing triazine-ring derivatives?
Discrepancies often arise from steric hindrance or electronic effects of substituents. For example, bulky groups (e.g., nitrobenzyl in derivative 8j) reduce yields due to unfavorable transition states . Use Design of Experiments (DoE) to statistically model variables (temperature, catalyst loading) and identify optimal conditions . Cross-validate results with HPLC purity analysis and kinetic studies.
Q. What strategies optimize copolymerization using this compound as a monomer?
Incorporate controlled radical polymerization (e.g., RAFT or ATRP) to regulate molecular weight distribution. Pre-functionalize the triazine ring with initiating groups (e.g., azide for click chemistry) to enhance reactivity with comonomers like DMDAAC . Monitor copolymer composition via H NMR and gel permeation chromatography (GPC) .
Q. How can computational modeling predict biological interactions of derivatives?
Employ molecular docking (e.g., AutoDock Vina) to simulate binding affinities with target enzymes (e.g., purine-processing kinases). Validate predictions with in vitro assays (e.g., IC measurements for adenosine receptor binding, as seen in triazole derivatives ). Pair with QSAR models to correlate substituent electronic parameters (Hammett constants) with activity .
Q. Methodological Considerations Table
Eigenschaften
IUPAC Name |
[3,4-diacetyloxy-5-(4-amino-2-oxo-1,3,5-triazin-1-yl)oxolan-2-yl]methyl acetate | |
---|---|---|
Details | Computed by Lexichem TK 2.7.0 (PubChem release 2021.05.07) | |
Source | PubChem | |
URL | https://pubchem.ncbi.nlm.nih.gov | |
Description | Data deposited in or computed by PubChem | |
InChI |
InChI=1S/C14H18N4O8/c1-6(19)23-4-9-10(24-7(2)20)11(25-8(3)21)12(26-9)18-5-16-13(15)17-14(18)22/h5,9-12H,4H2,1-3H3,(H2,15,17,22) | |
Details | Computed by InChI 1.0.6 (PubChem release 2021.05.07) | |
Source | PubChem | |
URL | https://pubchem.ncbi.nlm.nih.gov | |
Description | Data deposited in or computed by PubChem | |
InChI Key |
OTQJVHISAFFLMA-UHFFFAOYSA-N | |
Details | Computed by InChI 1.0.6 (PubChem release 2021.05.07) | |
Source | PubChem | |
URL | https://pubchem.ncbi.nlm.nih.gov | |
Description | Data deposited in or computed by PubChem | |
Canonical SMILES |
CC(=O)OCC1C(C(C(O1)N2C=NC(=NC2=O)N)OC(=O)C)OC(=O)C | |
Details | Computed by OEChem 2.3.0 (PubChem release 2021.05.07) | |
Source | PubChem | |
URL | https://pubchem.ncbi.nlm.nih.gov | |
Description | Data deposited in or computed by PubChem | |
Molecular Formula |
C14H18N4O8 | |
Details | Computed by PubChem 2.1 (PubChem release 2021.05.07) | |
Source | PubChem | |
URL | https://pubchem.ncbi.nlm.nih.gov | |
Description | Data deposited in or computed by PubChem | |
DSSTOX Substance ID |
DTXSID30908161 | |
Record name | 4-Imino-1-(2,3,5-tri-O-acetylpentofuranosyl)-1,4-dihydro-1,3,5-triazin-2-ol | |
Source | EPA DSSTox | |
URL | https://comptox.epa.gov/dashboard/DTXSID30908161 | |
Description | DSSTox provides a high quality public chemistry resource for supporting improved predictive toxicology. | |
Molecular Weight |
370.31 g/mol | |
Details | Computed by PubChem 2.1 (PubChem release 2021.05.07) | |
Source | PubChem | |
URL | https://pubchem.ncbi.nlm.nih.gov | |
Description | Data deposited in or computed by PubChem | |
CAS No. |
10302-78-0 | |
Record name | NSC291930 | |
Source | DTP/NCI | |
URL | https://dtp.cancer.gov/dtpstandard/servlet/dwindex?searchtype=NSC&outputformat=html&searchlist=291930 | |
Description | The NCI Development Therapeutics Program (DTP) provides services and resources to the academic and private-sector research communities worldwide to facilitate the discovery and development of new cancer therapeutic agents. | |
Explanation | Unless otherwise indicated, all text within NCI products is free of copyright and may be reused without our permission. Credit the National Cancer Institute as the source. | |
Record name | 4-Imino-1-(2,3,5-tri-O-acetylpentofuranosyl)-1,4-dihydro-1,3,5-triazin-2-ol | |
Source | EPA DSSTox | |
URL | https://comptox.epa.gov/dashboard/DTXSID30908161 | |
Description | DSSTox provides a high quality public chemistry resource for supporting improved predictive toxicology. | |
Retrosynthesis Analysis
AI-Powered Synthesis Planning: Our tool employs the Template_relevance Pistachio, Template_relevance Bkms_metabolic, Template_relevance Pistachio_ringbreaker, Template_relevance Reaxys, Template_relevance Reaxys_biocatalysis model, leveraging a vast database of chemical reactions to predict feasible synthetic routes.
One-Step Synthesis Focus: Specifically designed for one-step synthesis, it provides concise and direct routes for your target compounds, streamlining the synthesis process.
Accurate Predictions: Utilizing the extensive PISTACHIO, BKMS_METABOLIC, PISTACHIO_RINGBREAKER, REAXYS, REAXYS_BIOCATALYSIS database, our tool offers high-accuracy predictions, reflecting the latest in chemical research and data.
Strategy Settings
Precursor scoring | Relevance Heuristic |
---|---|
Min. plausibility | 0.01 |
Model | Template_relevance |
Template Set | Pistachio/Bkms_metabolic/Pistachio_ringbreaker/Reaxys/Reaxys_biocatalysis |
Top-N result to add to graph | 6 |
Feasible Synthetic Routes
Haftungsausschluss und Informationen zu In-Vitro-Forschungsprodukten
Bitte beachten Sie, dass alle Artikel und Produktinformationen, die auf BenchChem präsentiert werden, ausschließlich zu Informationszwecken bestimmt sind. Die auf BenchChem zum Kauf angebotenen Produkte sind speziell für In-vitro-Studien konzipiert, die außerhalb lebender Organismen durchgeführt werden. In-vitro-Studien, abgeleitet von dem lateinischen Begriff "in Glas", beinhalten Experimente, die in kontrollierten Laborumgebungen unter Verwendung von Zellen oder Geweben durchgeführt werden. Es ist wichtig zu beachten, dass diese Produkte nicht als Arzneimittel oder Medikamente eingestuft sind und keine Zulassung der FDA für die Vorbeugung, Behandlung oder Heilung von medizinischen Zuständen, Beschwerden oder Krankheiten erhalten haben. Wir müssen betonen, dass jede Form der körperlichen Einführung dieser Produkte in Menschen oder Tiere gesetzlich strikt untersagt ist. Es ist unerlässlich, sich an diese Richtlinien zu halten, um die Einhaltung rechtlicher und ethischer Standards in Forschung und Experiment zu gewährleisten.