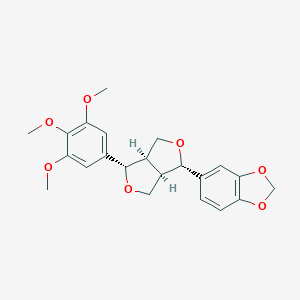
Aschantin
Übersicht
Beschreibung
Aschantin is a bioactive neolignan found in the flower buds of Magnolia flos and Hernandia nymphaeifolia . It exhibits various biological activities, including antioxidant, anti-inflammatory, cytotoxic, and antimicrobial properties . This compound is known for its peroxynitrite scavenging capacity, inhibition of inducible nitric oxide synthetase, antiplasmodial activity, calcium ion antagonistic activity, platelet activating factor-antagonistic activity, and chemopreventive or therapeutic activity mediated via inhibition of mammalian target of rapamycin kinase .
Vorbereitungsmethoden
Synthetic Routes and Reaction Conditions: The preparation of aschantin involves the extraction from natural sources such as the flower buds of Magnolia flos. The methanolic extract is suspended in water and extracted successively with hexane and chloroform . The synthetic route includes the use of various solvents and reagents to isolate and purify this compound.
Industrial Production Methods: Industrial production of this compound typically involves large-scale extraction and purification processes. The dried flower buds of Magnolia fargesii Cheng are used as the primary source, and the ethanol extract is developed as an effective alternative or complement to standard therapies based on its biological activities . The production process includes the use of liquid chromatography and high-resolution mass spectrometry to ensure the purity and quality of this compound .
Analyse Chemischer Reaktionen
Types of Reactions: Aschantin undergoes various chemical reactions, including oxidation, reduction, and substitution reactions. It is extensively metabolized in human and animal hepatocytes, producing multiple phase 1 and phase 2 metabolites .
Common Reagents and Conditions: The common reagents used in the reactions involving this compound include potassium phosphate buffer, magnesium chloride, and nicotinamide adenine dinucleotide phosphate . The reaction conditions typically involve incubation at 37°C in a shaking water bath .
Major Products Formed: The major products formed from the reactions of this compound include this compound catechol, O-desmethylthis compound, and hydroxythis compound . These metabolites are produced via O-demethylenation, catalyzed by cytochrome P450 enzymes .
Wissenschaftliche Forschungsanwendungen
Aschantin has a wide range of scientific research applications in chemistry, biology, medicine, and industry. In chemistry, it is used to study the inhibitory effects on cytochrome P450 and uridine 5′-diphospho-glucuronosyltransferase enzyme activities in human liver microsomes . In biology, this compound is investigated for its antioxidant and anti-inflammatory properties . In medicine, it is explored for its potential therapeutic applications in treating various diseases, including cancer and neuroinflammatory disorders . In industry, this compound is used in the development of natural medicine products and as a potential drug candidate .
Wirkmechanismus
Aschantin exerts its effects through various molecular targets and pathways. It inhibits the activities of cytochrome P450 enzymes, including CYP2C8, CYP2C9, CYP2C19, and CYP3A4 . It also inhibits the mammalian target of rapamycin kinase, leading to the inhibition of mTORC2/Akt and Akt/mTORC1/p70S6K signaling pathways . Additionally, this compound exhibits mechanism-based inhibition of cytochrome P450 enzymes, indicating its potential interactions with pharmacokinetic drugs in vivo .
Vergleich Mit ähnlichen Verbindungen
Aschantin is similar to other neolignans such as magnolin, eudesmin, fargesin, epimagnolin A, and yangambin . this compound is unique in its potent mechanism-based inhibition of cytochrome P450 enzymes and its wide range of biological activities . The structural differences between this compound and its epimer epi-aschantin do not significantly affect their anti-neuroinflammatory effects .
Eigenschaften
IUPAC Name |
5-[(3S,3aR,6S,6aR)-6-(3,4,5-trimethoxyphenyl)-1,3,3a,4,6,6a-hexahydrofuro[3,4-c]furan-3-yl]-1,3-benzodioxole | |
---|---|---|
Source | PubChem | |
URL | https://pubchem.ncbi.nlm.nih.gov | |
Description | Data deposited in or computed by PubChem | |
InChI |
InChI=1S/C22H24O7/c1-23-18-7-13(8-19(24-2)22(18)25-3)21-15-10-26-20(14(15)9-27-21)12-4-5-16-17(6-12)29-11-28-16/h4-8,14-15,20-21H,9-11H2,1-3H3/t14-,15-,20+,21+/m0/s1 | |
Source | PubChem | |
URL | https://pubchem.ncbi.nlm.nih.gov | |
Description | Data deposited in or computed by PubChem | |
InChI Key |
ONDWGDNAFRAXCN-VUEDXXQZSA-N | |
Source | PubChem | |
URL | https://pubchem.ncbi.nlm.nih.gov | |
Description | Data deposited in or computed by PubChem | |
Canonical SMILES |
COC1=CC(=CC(=C1OC)OC)C2C3COC(C3CO2)C4=CC5=C(C=C4)OCO5 | |
Source | PubChem | |
URL | https://pubchem.ncbi.nlm.nih.gov | |
Description | Data deposited in or computed by PubChem | |
Isomeric SMILES |
COC1=CC(=CC(=C1OC)OC)[C@@H]2[C@H]3CO[C@@H]([C@H]3CO2)C4=CC5=C(C=C4)OCO5 | |
Source | PubChem | |
URL | https://pubchem.ncbi.nlm.nih.gov | |
Description | Data deposited in or computed by PubChem | |
Molecular Formula |
C22H24O7 | |
Source | PubChem | |
URL | https://pubchem.ncbi.nlm.nih.gov | |
Description | Data deposited in or computed by PubChem | |
DSSTOX Substance ID |
DTXSID00156632 | |
Record name | Aschantin | |
Source | EPA DSSTox | |
URL | https://comptox.epa.gov/dashboard/DTXSID00156632 | |
Description | DSSTox provides a high quality public chemistry resource for supporting improved predictive toxicology. | |
Molecular Weight |
400.4 g/mol | |
Source | PubChem | |
URL | https://pubchem.ncbi.nlm.nih.gov | |
Description | Data deposited in or computed by PubChem | |
CAS No. |
13060-15-6 | |
Record name | (+)-Aschantin | |
Source | CAS Common Chemistry | |
URL | https://commonchemistry.cas.org/detail?cas_rn=13060-15-6 | |
Description | CAS Common Chemistry is an open community resource for accessing chemical information. Nearly 500,000 chemical substances from CAS REGISTRY cover areas of community interest, including common and frequently regulated chemicals, and those relevant to high school and undergraduate chemistry classes. This chemical information, curated by our expert scientists, is provided in alignment with our mission as a division of the American Chemical Society. | |
Explanation | The data from CAS Common Chemistry is provided under a CC-BY-NC 4.0 license, unless otherwise stated. | |
Record name | Aschantin | |
Source | ChemIDplus | |
URL | https://pubchem.ncbi.nlm.nih.gov/substance/?source=chemidplus&sourceid=0013060156 | |
Description | ChemIDplus is a free, web search system that provides access to the structure and nomenclature authority files used for the identification of chemical substances cited in National Library of Medicine (NLM) databases, including the TOXNET system. | |
Record name | Aschantin | |
Source | EPA DSSTox | |
URL | https://comptox.epa.gov/dashboard/DTXSID00156632 | |
Description | DSSTox provides a high quality public chemistry resource for supporting improved predictive toxicology. | |
Retrosynthesis Analysis
AI-Powered Synthesis Planning: Our tool employs the Template_relevance Pistachio, Template_relevance Bkms_metabolic, Template_relevance Pistachio_ringbreaker, Template_relevance Reaxys, Template_relevance Reaxys_biocatalysis model, leveraging a vast database of chemical reactions to predict feasible synthetic routes.
One-Step Synthesis Focus: Specifically designed for one-step synthesis, it provides concise and direct routes for your target compounds, streamlining the synthesis process.
Accurate Predictions: Utilizing the extensive PISTACHIO, BKMS_METABOLIC, PISTACHIO_RINGBREAKER, REAXYS, REAXYS_BIOCATALYSIS database, our tool offers high-accuracy predictions, reflecting the latest in chemical research and data.
Strategy Settings
Precursor scoring | Relevance Heuristic |
---|---|
Min. plausibility | 0.01 |
Model | Template_relevance |
Template Set | Pistachio/Bkms_metabolic/Pistachio_ringbreaker/Reaxys/Reaxys_biocatalysis |
Top-N result to add to graph | 6 |
Feasible Synthetic Routes
Q1: What is the primary molecular target of aschantin?
A1: this compound directly targets the kinase domain of the mammalian target of rapamycin (mTOR). []
Q2: How does this compound interact with mTOR?
A2: this compound acts as an mTOR kinase inhibitor by competitively binding to the active pocket of the kinase domain, thereby blocking adenosine triphosphate (ATP) binding. []
Q3: What are the downstream effects of this compound's inhibition of mTOR?
A3: this compound's inhibition of mTOR leads to a cascade of downstream effects: * Inhibition of epidermal growth factor (EGF)-induced full activation of Akt by preventing phosphorylation at Ser473/Thr308. []* Suppression of the mTORC2/Akt and Akt/mTORC1/p70S6K signaling pathways. []* Activation of GSK3β by preventing Akt-mediated phosphorylation of GSK3β at Ser9. []* GSK3β activation leads to c-Jun phosphorylation at Ser243, ultimately promoting c-Jun degradation through the ubiquitination-mediated proteasomal pathway. []
Q4: What is the molecular formula and weight of this compound?
A4: While not explicitly provided in the given research, the molecular formula of this compound can be deduced as C22H26O7 based on its classification as a furofuran lignan and supporting structural data. [, , ] The molecular weight is calculated as 402.44 g/mol.
Q5: What spectroscopic data is available for this compound?
A5: Various studies mention the use of spectroscopic methods to characterize this compound, including:* Nuclear Magnetic Resonance (NMR): Used to determine the structure and stereochemistry of this compound and its isomers. [, , , ]* Mass Spectrometry (MS): Employed for molecular weight determination and structural elucidation. [, ]* Ultraviolet-Visible (UV) Spectroscopy: Used to analyze the characteristic absorption patterns of this compound, indicating the presence of aromatic rings. []* Infrared (IR) Spectroscopy: Reveals functional groups present in this compound, such as hydroxyl groups and aromatic systems. [] * Circular Dichroism (CD) Spectroscopy: Aids in determining the absolute configuration of this compound and its isomers. [, ]
Q6: What are the potential therapeutic applications of this compound?
A6: this compound exhibits several promising pharmacological activities, suggesting potential applications in:* Cancer: this compound demonstrates anti-proliferative and anti-tumor activities by inhibiting the mTOR pathway, a key regulator of cell growth and proliferation. [] It shows potential as a chemopreventive or therapeutic agent against various cancers, including skin, prostate, and pancreatic cancer. []* Allergies: this compound exhibits anti-allergic effects by suppressing mast cell activation. [, ] It could potentially be used to treat allergic rhinitis and sinusitis. [, ] * Inflammation: this compound exhibits anti-inflammatory effects, likely due to its inhibition of iNOS expression and peroxynitrite scavenging activity. [] This suggests potential applications in treating neuro-inflammatory diseases. []
Q7: What are the in vivo effects of this compound?
A8: While the provided research focuses primarily on in vitro studies, some highlight the potential for in vivo efficacy:* this compound demonstrates anti-allergic effects in IgE-induced passive cutaneous anaphylaxis mice models. [, ]* It suppresses tumor growth in animal models, though specific details are not provided. []
Q8: What is known about the metabolism of this compound?
A9: Research indicates that this compound undergoes metabolism in human liver microsomes, primarily mediated by cytochrome P450 (CYP) enzymes, particularly CYP2C8, CYP2C9, CYP2C19, and CYP3A4. [, ] Further studies are needed to fully elucidate the metabolic pathways and identify specific metabolites.
Q9: Are there potential drug interactions with this compound?
A10: Given its potent inhibition of specific CYP enzymes, this compound has the potential to interact with drugs metabolized by these enzymes. [, ] Co-administration with such drugs could lead to altered drug levels and potential adverse effects.
Q10: What is the safety profile of this compound?
A11: While the provided research mentions this compound being "abundantly found in Magnolia flos," a traditional medicine, specific toxicity data are limited. * One study classifies an ethanolic extract of Etlingera alba rhizome containing this compound as "medium toxic" based on its LC50 value. [] * Further research, particularly in vivo studies, is crucial to comprehensively assess the toxicity and long-term safety profile of this compound.
Haftungsausschluss und Informationen zu In-Vitro-Forschungsprodukten
Bitte beachten Sie, dass alle Artikel und Produktinformationen, die auf BenchChem präsentiert werden, ausschließlich zu Informationszwecken bestimmt sind. Die auf BenchChem zum Kauf angebotenen Produkte sind speziell für In-vitro-Studien konzipiert, die außerhalb lebender Organismen durchgeführt werden. In-vitro-Studien, abgeleitet von dem lateinischen Begriff "in Glas", beinhalten Experimente, die in kontrollierten Laborumgebungen unter Verwendung von Zellen oder Geweben durchgeführt werden. Es ist wichtig zu beachten, dass diese Produkte nicht als Arzneimittel oder Medikamente eingestuft sind und keine Zulassung der FDA für die Vorbeugung, Behandlung oder Heilung von medizinischen Zuständen, Beschwerden oder Krankheiten erhalten haben. Wir müssen betonen, dass jede Form der körperlichen Einführung dieser Produkte in Menschen oder Tiere gesetzlich strikt untersagt ist. Es ist unerlässlich, sich an diese Richtlinien zu halten, um die Einhaltung rechtlicher und ethischer Standards in Forschung und Experiment zu gewährleisten.