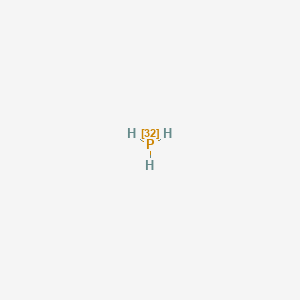
Phosphorus-32
- Klicken Sie auf QUICK INQUIRY, um ein Angebot von unserem Expertenteam zu erhalten.
- Mit qualitativ hochwertigen Produkten zu einem WETTBEWERBSFÄHIGEN Preis können Sie sich mehr auf Ihre Forschung konzentrieren.
Übersicht
Beschreibung
Phosphor-32 ist ein radioaktives Isotop von Phosphor. Es enthält 15 Protonen und 17 Neutronen, was es um ein Neutron schwerer macht als das häufigste Isotop, Phosphor-31 . Phosphor-32 ist bekannt für seine radiozytotoxische Aktivität, bei der Beta-Teilchen emittiert werden, was es in verschiedenen wissenschaftlichen und medizinischen Anwendungen nützlich macht .
Vorbereitungsmethoden
Phosphor-32 kann durch verschiedene Verfahren hergestellt werden:
- Synthetische Wege: Eine übliche Methode beinhaltet die Bestrahlung von Schwefel-32 mit mittelschnellen Neutronen, was zur Bildung von Phosphor-32 und einem Proton führt . Die Kernreaktion für diese Reaktion lautet:
1632S+n→1532P+p
Industrielle Produktion: Eine weitere Methode beinhaltet die Bestrahlung von rotem Phosphor mit schnellen Neutronen.
Analyse Chemischer Reaktionen
Phosphor-32 unterliegt verschiedenen Arten von chemischen Reaktionen:
- Beta-Zerfall: Phosphor-32 zerfällt durch Beta-Zerfall zu Schwefel-32 und setzt dabei 1,709 MeV Energie frei . Die Kernreaktion für diesen Zerfall lautet:
1532P→1632S+e−+νe
Oxidation und Reduktion: Phosphor-32 kann, ähnlich wie andere Phosphorisotope, an Oxidations- und Reduktionsreaktionen teilnehmen. Häufige Reagenzien umfassen Oxidationsmittel wie Kaliumpermanganat und Reduktionsmittel wie Natriumborhydrid.
Substitutionsreaktionen: Phosphor-32 kann Substitutionsreaktionen eingehen, bei denen es ein Phosphoratom in einem Molekül ersetzt.
Wissenschaftliche Forschungsanwendungen
Phosphor-32 hat eine breite Palette von Anwendungen in der wissenschaftlichen Forschung:
5. Wirkmechanismus
Phosphor-32 übt seine Wirkungen durch die Emission von Beta-Teilchen aus, die die zelluläre DNA direkt schädigen. Dieser Schaden wird durch die Ionisierung von intrazellulärem Wasser verursacht, wodurch zytotoxische freie Radikale und Superoxide entstehen, die zum Tod von Tumorzellen führen . Der einzigartige Mechanismus der direkten Einarbeitung in neu entstehende DNA-Ketten führt zu Doppelstrangbrüchen, die von anderen Elektronen emittierenden Radioisotopen nicht repliziert werden können .
Wirkmechanismus
Phosphorus P-32 exerts its effects through the emission of beta particles, which directly damage cellular DNA. This damage is caused by ionizing intracellular water to produce cytotoxic free radicals and superoxides, resulting in tumor cell death . The unique mechanism of direct incorporation into nascent DNA chains leads to double-stranded breakage, which is not replicable by other electron-emitting radioisotopes .
Vergleich Mit ähnlichen Verbindungen
Phosphor-32 wird oft mit anderen Beta-emittierenden Radioisotopen wie Jod-131 und Yttrium-90 verglichen:
Yttrium-90: Yttrium-90 ist ein stärkerer Beta-Emitter als Phosphor-32 und wird in der Radioembolisation zur Behandlung von Leberkrebs eingesetzt.
Phosphor-32 zeichnet sich durch seinen einzigartigen Wirkmechanismus und seine Fähigkeit aus, in einer Vielzahl von Anwendungen eingesetzt zu werden, von medizinischen Behandlungen bis hin zur wissenschaftlichen Forschung.
Eigenschaften
CAS-Nummer |
14596-37-3 |
---|---|
Molekularformel |
H3P |
Molekulargewicht |
34.998 g/mol |
IUPAC-Name |
phosphane |
InChI |
InChI=1S/H3P/h1H3/i1+1 |
InChI-Schlüssel |
XYFCBTPGUUZFHI-OUBTZVSYSA-N |
SMILES |
P |
Isomerische SMILES |
[32PH3] |
Kanonische SMILES |
P |
14596-37-3 | |
Synonyme |
32P radioisotope P-32 radioisotope Phosphorus-32 |
Herkunft des Produkts |
United States |
Retrosynthesis Analysis
AI-Powered Synthesis Planning: Our tool employs the Template_relevance Pistachio, Template_relevance Bkms_metabolic, Template_relevance Pistachio_ringbreaker, Template_relevance Reaxys, Template_relevance Reaxys_biocatalysis model, leveraging a vast database of chemical reactions to predict feasible synthetic routes.
One-Step Synthesis Focus: Specifically designed for one-step synthesis, it provides concise and direct routes for your target compounds, streamlining the synthesis process.
Accurate Predictions: Utilizing the extensive PISTACHIO, BKMS_METABOLIC, PISTACHIO_RINGBREAKER, REAXYS, REAXYS_BIOCATALYSIS database, our tool offers high-accuracy predictions, reflecting the latest in chemical research and data.
Strategy Settings
Precursor scoring | Relevance Heuristic |
---|---|
Min. plausibility | 0.01 |
Model | Template_relevance |
Template Set | Pistachio/Bkms_metabolic/Pistachio_ringbreaker/Reaxys/Reaxys_biocatalysis |
Top-N result to add to graph | 6 |
Feasible Synthetic Routes
Haftungsausschluss und Informationen zu In-Vitro-Forschungsprodukten
Bitte beachten Sie, dass alle Artikel und Produktinformationen, die auf BenchChem präsentiert werden, ausschließlich zu Informationszwecken bestimmt sind. Die auf BenchChem zum Kauf angebotenen Produkte sind speziell für In-vitro-Studien konzipiert, die außerhalb lebender Organismen durchgeführt werden. In-vitro-Studien, abgeleitet von dem lateinischen Begriff "in Glas", beinhalten Experimente, die in kontrollierten Laborumgebungen unter Verwendung von Zellen oder Geweben durchgeführt werden. Es ist wichtig zu beachten, dass diese Produkte nicht als Arzneimittel oder Medikamente eingestuft sind und keine Zulassung der FDA für die Vorbeugung, Behandlung oder Heilung von medizinischen Zuständen, Beschwerden oder Krankheiten erhalten haben. Wir müssen betonen, dass jede Form der körperlichen Einführung dieser Produkte in Menschen oder Tiere gesetzlich strikt untersagt ist. Es ist unerlässlich, sich an diese Richtlinien zu halten, um die Einhaltung rechtlicher und ethischer Standards in Forschung und Experiment zu gewährleisten.