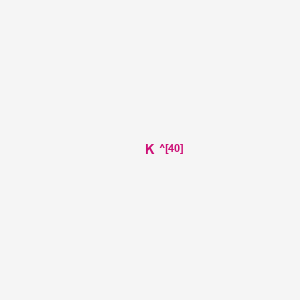
Potassium K-40
Übersicht
Beschreibung
Potassium-40 (K-40) is a naturally occurring radioactive isotope of potassium, constituting approximately 0.0117% of natural potassium . It has a half-life of 1.248 billion years and decays via beta decay (89.3%) to calcium-40 and electron capture (10.7%) to argon-40 . K-40 emits gamma rays at 1461 keV, making it detectable via gamma spectroscopy .
Key Properties of K-40:
K-40 is ubiquitous in nature due to potassium’s presence in minerals, fertilizers, and biological systems.
Vorbereitungsmethoden
Natural Occurrence and Challenges in ⁴⁰K Extraction
Abundance and Decay Characteristics
Potassium-40 exists in trace amounts within natural potassium, with an isotopic abundance of 0.012% (120 parts per million) . Its decay pathways include beta emission to calcium-40 (89.6% probability), electron capture to argon-40 (10.3%), and positron emission (0.001%) . The low natural abundance and complex decay profile necessitate specialized enrichment strategies to obtain usable quantities of ⁴⁰K.
Limitations of Conventional Extraction
Traditional chemical separation methods, such as fractional crystallization or ion exchange, struggle to isolate ⁴⁰K due to its nearly identical chemical behavior to stable isotopes (³⁹K and ⁴¹K) . Furthermore, the minute isotopic differences in atomic mass (Δm = 1–2 atomic mass units) render centrifugal and electromagnetic separation inefficient without prior enrichment .
Magneto-Optical Isotope Enrichment
Principles of Laser Cooling and Trapping
Magneto-optical trapping (MOT) exploits isotope-specific shifts in atomic energy levels to selectively cool and trap ⁴⁰K atoms. By tuning lasers to the D1 and D2 transitions of potassium (767 nm and 770 nm, respectively), researchers achieve resonance conditions unique to ⁴⁰K, enabling spatial separation from other isotopes . A recent breakthrough demonstrated 2D transverse cooling, reducing the divergence angle of the atomic beam and enhancing collimation .
Enrichment Performance
In 2024, a magneto-optical enrichment system achieved a 1,000-fold increase in ⁴⁰K concentration, elevating its abundance from 0.012% to 12–20% . This method employs a Halbach-type magnet array to deflect laser-cooled ⁴⁰K atoms into a collection chamber, while uncooled isotopes (³⁹K, ⁴¹K) continue unimpeded. The process yields milligram quantities of ⁴⁰K with minimal contamination, making it suitable for quantum gas experiments .
Table 1: Magneto-Optical Enrichment Parameters
Parameter | Value | Source |
---|---|---|
Initial Abundance | 0.012% | |
Final Abundance | 12–20% | |
Enrichment Factor | 1,000× | |
Throughput | 10¹² atoms/hour |
Chemical Purification and the Saiger Process
Oxide Removal via Surface Adhesion
The Saiger process purifies metallic potassium by exploiting the preferential adhesion of potassium oxides (K₂O, KO₂) to glass surfaces. Molten potassium is poured over a heated glass column, where oxides form a slag layer, while purified metal flows into an argon-filled ampoule4. This method, though non-selective for isotopes, is critical for preparing high-purity potassium feedstock prior to isotopic enrichment.
Operational Challenges
Early attempts at Saiger purification faced contamination from residual moisture and oxygen, resulting in suboptimal yields (~50% recovery)4. Modern implementations employ vacuum baking (10⁻⁶ mbar) and argon flushing (99.996% purity) to mitigate oxidation. Despite these improvements, the process remains limited to bulk purification and requires subsequent isotopic separation4.
Chromatographic Separation for High-Purity ⁴⁰K
Single-Column Ion Exchange
An optimized chromatographic method using Bio-Rad AG50W-X8 resin achieves 99.5% potassium recovery with <10 ng procedural blank . The resin selectively retains divalent cations (e.g., Ca²⁺, Mg²⁺) while allowing monovalent K⁺ to elute in nitric acid (0.5M HNO₃). This step is essential for removing matrix elements prior to mass spectrometric analysis or further enrichment .
Coupling with MC-ICP-MS
High-purity potassium isolates are analyzed via multi-collector inductively coupled plasma mass spectrometry (MC-ICP-MS) under "cold" plasma conditions to minimize argon-based interferences (e.g., ³⁸ArH⁺, ⁴⁰Ar⁺) . A dummy bucket collector deflects high-intensity ⁴⁰Ar beams, improving precision to ±0.06‰ for δ⁴¹K measurements .
Table 2: Chromatographic Separation Efficiency
Metric | Performance | Source |
---|---|---|
Column Yield | 99.5 ± 0.6% | |
Procedural Blank | <10 ng K | |
Analysis Precision (δ⁴¹K) | ±0.06‰ (2SD) |
Laser Isotope Separation (LIS)
Resonance Ionization Mass Spectrometry
LIS utilizes the isotope shift in potassium’s electronic transitions (≈1 GHz between ⁴⁰K and ³⁹K) to selectively ionize ⁴⁰K atoms. A two-laser system—a 766.7 nm pump laser and a 693.9 nm ionization laser—excites ⁴⁰K to Rydberg states, which are then ionized by an electric field . This method achieves 95% ionization efficiency but requires ultrahigh vacuum (<10⁻⁹ mbar) to avoid collisional quenching .
Scalability and Cost Considerations
While LIS produces ⁴⁰K with >90% isotopic purity, its energy consumption (≈10 kW per gram) and infrastructure costs limit industrial adoption . Recent advancements in diode-pumped solid-state lasers (DPSSL) have reduced power requirements by 40%, making the technique viable for laboratory-scale enrichment .
Analyse Chemischer Reaktionen
Radioactive Decay Pathways
⁴⁰K undergoes three primary decay modes, each producing distinct daughter nuclides and emission products:
1.1 Beta-minus decay (β⁻)
1.2 Electron capture (EC)
-
Probability : 10.3%
-
Energy released : 1.46 MeV (emitted as gamma radiation during de-excitation of ⁴⁰Ar*) .
1.3 Positron emission (β⁺)
Experimental Data and Activity Calculations
The radioactivity of ⁴⁰K in potassium chloride (KCl) samples can be quantified using Geiger-Müller counters. Key experimental data from controlled studies includes:
Mass of KCl (g) | Background Radiation (counts/sec) | KCl Radiation (counts/sec) | Time Interval (sec) |
---|---|---|---|
2.00 | 0.172 | 0.352 | 900 |
Activity Calculation
Half-life relationship :
, where is the decay constant. For ⁴⁰K, .
Argon accumulation in Earth’s atmosphere
⁴⁰K’s electron capture decay contributes ~1% of atmospheric argon, primarily as ⁴⁰Ar. This process has been critical in radiometric dating methods like potassium-argon dating .
Role in mitochondrial physiology
Recent hypotheses propose that ⁴⁰K’s beta decays produce Auger and Coster-Kronig electrons (ACKE), which ionize nearby molecules. Key findings include:
-
Hydrogen peroxide (H₂O₂) formation : ACKE generates microquantities of H₂O₂ via superoxide intermediates, acting as signaling molecules in cellular processes .
-
Electron transport chain modulation : ⁴⁰K-derived electrons may interact with ubiquinone (CoQ₁₀) in mitochondria, potentially aiding electron transfer during temporary metabolic blocks .
Decay Energy Distribution
Decay Mode | Energy (MeV) | Frequency (%) |
---|---|---|
β⁻ to ⁴⁰Ca | 1.31 | 89.6 |
EC to ⁴⁰Ar* | 1.46 | 10.3 |
β⁺ to ⁴⁰Ar | 0.48 | 0.001 |
Wissenschaftliche Forschungsanwendungen
Geochronology: Potassium-Argon Dating
K-40 is crucial in geochronology, particularly in potassium-argon (K-Ar) dating. This method measures the ratio of K-40 to argon-40 (Ar-40) in mineral samples to determine their age. The process is based on the principle that when a mineral forms, it initially contains no Ar-40. As K-40 decays over time, Ar-40 accumulates, allowing scientists to calculate the time elapsed since the mineral's formation.
Sample Type | Age Range | Typical Use |
---|---|---|
Volcanic Rocks | 100,000 years to billions of years | Dating geological events |
Metamorphic Rocks | 1 million years to billions of years | Understanding tectonic processes |
Biological and Medical Research
Recent studies have suggested that K-40 may play a role in cellular physiology and mitochondrial function. The beta decay of K-40 can produce low-energy electrons that may influence cellular processes:
- Cellular Homeostasis : K-40's beta radiation could contribute to maintaining cellular homeostasis by influencing electron transfer mechanisms within mitochondria .
Case Study:
A study proposed using potassium enriched with K-40 in conjunction with Coenzyme Q10 for therapeutic purposes in mitochondrial dysfunction diseases . This approach aims to explore whether K-40 can aid in energy production during mitochondrial stress.
Environmental Monitoring
K-40 is a significant contributor to natural background radiation and is used in environmental monitoring:
- Soil and Food Safety : The presence of K-40 is measured in soils and food products to assess natural radioactivity levels. High-resolution gamma spectrometry is commonly employed for this purpose .
Measurement Type | Detection Method | Application |
---|---|---|
Soil Samples | Gamma Spectrometry | Assessing radioactivity levels |
Food Products | Portable Detectors | Ensuring safety standards |
Case Study 1: Geological Applications
In a study conducted in volcanic regions, researchers applied K-Ar dating to determine the ages of lava flows, providing insights into volcanic activity timelines and tectonic movements over millions of years.
Case Study 2: Biological Impact
Research at the Waste Isolation Pilot Plant explored how varying levels of background radiation, including K-40, affected seed germination and growth rates, suggesting potential influences on biological systems due to low-level radiation exposure .
Wirkmechanismus
Potassium-40 exerts its effects through radioactive decay. The decay processes involve the transformation of potassium-40 into other elements (calcium-40 and argon-40) with the emission of particles and radiation. These decay events contribute to natural radioactivity and can be detected and measured using radiation detection equipment .
Vergleich Mit ähnlichen Verbindungen
Comparison with Other Potassium Isotopes
Stable Isotopes: K-39 and K-41
Natural potassium comprises 93.2581% K-39 and 6.7302% K-41, both stable .
Isotopic Composition of Natural Potassium:
Isotope | Abundance (%) | Stability |
---|---|---|
K-39 | 93.2581 | Stable |
K-40 | 0.0117 | Radioactive |
K-41 | 6.7302 | Stable |
Comparison with Other Natural Radionuclides
Uranium and Thorium Series (U-238, Th-232)
- Correlation in Environmental Samples:
Activity Concentrations in Legumes (Iraqi Market Samples) :
Radionuclide | Average Activity (Bq/kg) |
---|---|
U-238 | 7.475 |
Th-232 | 3.799 |
K-40 | 385.248 |
Cesium-137 (Cs-137) and Strontium-90 (Sr-90)
- Uptake Mechanisms:
- Environmental Persistence:
K-40 in Agricultural and Environmental Systems
Fertilizers and Soil Enrichment
- Potassium fertilizers derived from potash deposits contain K-40 at natural abundance levels (0.0117%) .
Transfer to Crops
Transfer Factors (TFs):
Crop/Plant K-40 TF Range Temperate Crops 0.73–1.3 Grassland Species 0.99 ± 0.023
Health and Dosimetry Comparisons
Radiation Dose from K-40
- Annual Effective Dose:
Risk Comparison with Artificial Radionuclides
- K-40 poses lower risk than Cs-137 or Sr-90, which concentrate in specific tissues and have higher radiotoxicity .
Biologische Aktivität
Potassium-40 () is a naturally occurring radioactive isotope of potassium, constituting approximately 0.012% of all potassium found in nature. Its biological activity is significant due to its role in human physiology and potential implications for health and disease. This article explores the biological activity of , including its physiological roles, mechanisms of action, health effects, and relevant research findings.
Physiological Role of Potassium-40
Essential Nutrient
Potassium is a crucial electrolyte in the human body, necessary for various physiological processes including nerve transmission, muscle contraction, and maintaining fluid balance. behaves similarly to stable potassium isotopes in biological systems, being absorbed through dietary intake and distributed throughout the body. An adult male typically contains about 0.1 microcurie (µCi) of , contributing to a radiation dose of approximately 18 millirem (mrem) annually to soft tissues and 14 mrem to bones from its decay products .
Mitochondrial Function
Recent studies suggest that may play a role in mitochondrial physiology. The beta decay of produces low-energy electrons, known as Auger and Coster-Kronig electrons (ACKE), which can influence mitochondrial function by providing energy under specific conditions, such as hypoxia or nutrient deficiency . This mechanism may help sustain cellular energy production during metabolic stress.
Beta Decay and Radiation Effects
The decay of primarily occurs through beta decay and electron capture, leading to the formation of stable argon-40 (). Approximately 10% of the decays result in electron capture, which can impact cellular structures due to the emitted radiation . The radiation emitted can cause ionization in nearby molecules, potentially leading to oxidative stress if not adequately managed by cellular antioxidants.
Homeostatic Control
The body regulates potassium levels tightly through homeostatic mechanisms. The biological half-life of is about 30 days, ensuring that excess potassium is excreted while maintaining necessary physiological levels . This regulation is crucial as both deficiency and excess can lead to significant health issues.
Health Implications
Radiation Exposure Risks
While is essential for health, it also poses risks due to its radioactive nature. External exposure from environmental sources can lead to increased radiation doses, while internal exposure from dietary sources can result in beta particle emissions within tissues . Chronic exposure has been linked to potential health effects including increased cancer risk, although the doses from natural sources are generally considered low.
Therapeutic Potential
Interestingly, some researchers propose that the unique properties of could be harnessed therapeutically. For instance, combining with coenzyme Q10 (CoQ10) may enhance mitochondrial function in patients with mitochondrial disorders by improving energy efficiency during metabolic crises . This hypothesis requires further investigation but highlights the dual role of as both a nutrient and a potential therapeutic agent.
Table 1: Key Research Studies on Potassium-40
Case Studies
- Mitochondrial Dysfunction : A study involving patients with mitochondrial diseases suggested that supplementation with CoQ10 alongside potassium-rich diets may improve energy levels and reduce symptoms associated with mitochondrial dysfunctions like epilepsy and neurodegenerative diseases .
- Population Studies : Research conducted on diverse populations indicated variations in body content based on age and gender, with men generally exhibiting higher concentrations than women across all age groups .
Q & A
Basic Research Questions
Q. What are the primary decay modes and half-life of K-40, and how do they influence its detection in environmental samples?
K-40 decays via beta emission (89.3%) to Ca-40 and electron capture (10.7%) to Ar-40, emitting gamma rays (1460.81 keV) during the latter process . Its half-life of 1.25–1.28 billion years necessitates sensitive detection methods such as gamma spectrometry. The dual decay pathways allow researchers to leverage decay-specific signatures (e.g., gamma peaks for Ar-40) to differentiate K-40 from other isotopes in complex matrices like soils or biological tissues .
Q. Why is K-40 not classified as a hazardous radioactive substance despite its natural radioactivity?
K-40 emits low-energy radiation (beta particles ≤1.31 MeV and weak gamma rays), which is insufficient to pose significant health risks in typical environmental or biological concentrations . Regulatory frameworks exempt K-40 from hazardous categorization because its activity (e.g., ~55 Bq/kg in the human body) falls below thresholds for radiation source classification .
Q. How is gamma spectroscopy utilized to quantify K-40 activity in geological or biological samples?
Gamma spectroscopy targets the 1460.81 keV gamma ray emitted during K-40’s electron capture decay . Researchers calibrate detectors using certified reference materials (e.g., potassium dichromate) to account for geometric efficiency and self-absorption effects in samples . Activity is calculated using the formula:
where = activity (Bq/kg), = net gamma counts, = detector efficiency, = counting time, and = sample mass .
Advanced Research Questions
Q. How can discrepancies between theoretical and experimental K-40 radioactivity concentrations in agricultural products be methodologically addressed?
Theoretical estimates of K-40 activity in fertilizers (e.g., 15,162 Bq/kg) often diverge from experimental gamma spectroscopy results (e.g., 15,242 Bq/kg) due to inhomogeneous isotopic distribution or matrix interference . To resolve this, researchers use neutron activation analysis (NAA) with K-42 as a tracer, which accounts for potassium heterogeneity and improves precision by correlating K-42 gamma emissions (1,524 keV) with K-40 content .
Q. What are the challenges in using neutron activation analysis (NAA) with K-42 to determine K-40 content, and how are they mitigated?
K-42’s short half-life (12.36 hours) limits its utility for long-term studies, while interference from sodium-24 (Na-24) in salt-rich samples can distort gamma spectra . Mitigation strategies include:
- Pre-irradiation chemical separation to isolate potassium.
- Time-delayed counting to allow Na-24 (15-hour half-life) to decay before measurement .
Q. What calibration techniques ensure accuracy in K-40 activity measurements when using gamma spectrometry?
Calibration requires reference standards with known K-40 activity, such as potassium dichromate (K₂Cr₂O₇), to account for detector efficiency and sample geometry . For example, a 297 g K₂Cr₂O₇ sample yielded a calculated activity of 1,284 Bq, but measured activity (1,356 Bq) revealed a 5.6% deviation, highlighting the need for Monte Carlo simulations (e.g., MCC software) to correct for gamma-ray attenuation in complex matrices .
Q. How does whole-body liquid scintillation counting address the measurement of endogenous K-40, and what are its limitations?
This method quantifies total body potassium by detecting K-40’s beta emissions, assuming 0.012% isotopic abundance . However, high equipment costs (~$500,000) and technical challenges (e.g., background radiation interference) limit its accessibility. Alternative approaches, such as stable isotope dilution (K-41/K-39 ratios), are being explored for in vivo body composition studies .
Eigenschaften
IUPAC Name |
potassium-40 | |
---|---|---|
Source | PubChem | |
URL | https://pubchem.ncbi.nlm.nih.gov | |
Description | Data deposited in or computed by PubChem | |
InChI |
InChI=1S/K/i1+1 | |
Source | PubChem | |
URL | https://pubchem.ncbi.nlm.nih.gov | |
Description | Data deposited in or computed by PubChem | |
InChI Key |
ZLMJMSJWJFRBEC-OUBTZVSYSA-N | |
Source | PubChem | |
URL | https://pubchem.ncbi.nlm.nih.gov | |
Description | Data deposited in or computed by PubChem | |
Canonical SMILES |
[K] | |
Source | PubChem | |
URL | https://pubchem.ncbi.nlm.nih.gov | |
Description | Data deposited in or computed by PubChem | |
Isomeric SMILES |
[40K] | |
Source | PubChem | |
URL | https://pubchem.ncbi.nlm.nih.gov | |
Description | Data deposited in or computed by PubChem | |
Molecular Formula |
K | |
Source | PubChem | |
URL | https://pubchem.ncbi.nlm.nih.gov | |
Description | Data deposited in or computed by PubChem | |
DSSTOX Substance ID |
DTXSID10904161 | |
Record name | Potassium-40 | |
Source | EPA DSSTox | |
URL | https://comptox.epa.gov/dashboard/DTXSID10904161 | |
Description | DSSTox provides a high quality public chemistry resource for supporting improved predictive toxicology. | |
Molecular Weight |
39.9639982 g/mol | |
Source | PubChem | |
URL | https://pubchem.ncbi.nlm.nih.gov | |
Description | Data deposited in or computed by PubChem | |
Physical Description |
A naturally occurring isotope that comprises about 0.012% of total potassium; K-39 (93% of potassium) and K-39 (7% of potassium) are not radioactive. Elemental potassium is a soft, silver-white metal. [Argonne] | |
Record name | Potassium-40 | |
Source | Haz-Map, Information on Hazardous Chemicals and Occupational Diseases | |
URL | https://haz-map.com/Agents/1832 | |
Description | Haz-Map® is an occupational health database designed for health and safety professionals and for consumers seeking information about the adverse effects of workplace exposures to chemical and biological agents. | |
Explanation | Copyright (c) 2022 Haz-Map(R). All rights reserved. Unless otherwise indicated, all materials from Haz-Map are copyrighted by Haz-Map(R). No part of these materials, either text or image may be used for any purpose other than for personal use. Therefore, reproduction, modification, storage in a retrieval system or retransmission, in any form or by any means, electronic, mechanical or otherwise, for reasons other than personal use, is strictly prohibited without prior written permission. | |
CAS No. |
13966-00-2 | |
Record name | Potassium-40 | |
Source | CAS Common Chemistry | |
URL | https://commonchemistry.cas.org/detail?cas_rn=13966-00-2 | |
Description | CAS Common Chemistry is an open community resource for accessing chemical information. Nearly 500,000 chemical substances from CAS REGISTRY cover areas of community interest, including common and frequently regulated chemicals, and those relevant to high school and undergraduate chemistry classes. This chemical information, curated by our expert scientists, is provided in alignment with our mission as a division of the American Chemical Society. | |
Explanation | The data from CAS Common Chemistry is provided under a CC-BY-NC 4.0 license, unless otherwise stated. | |
Record name | Potassium-40 | |
Source | EPA DSSTox | |
URL | https://comptox.epa.gov/dashboard/DTXSID10904161 | |
Description | DSSTox provides a high quality public chemistry resource for supporting improved predictive toxicology. | |
Synthesis routes and methods I
Procedure details
Synthesis routes and methods II
Procedure details
Synthesis routes and methods III
Procedure details
Haftungsausschluss und Informationen zu In-Vitro-Forschungsprodukten
Bitte beachten Sie, dass alle Artikel und Produktinformationen, die auf BenchChem präsentiert werden, ausschließlich zu Informationszwecken bestimmt sind. Die auf BenchChem zum Kauf angebotenen Produkte sind speziell für In-vitro-Studien konzipiert, die außerhalb lebender Organismen durchgeführt werden. In-vitro-Studien, abgeleitet von dem lateinischen Begriff "in Glas", beinhalten Experimente, die in kontrollierten Laborumgebungen unter Verwendung von Zellen oder Geweben durchgeführt werden. Es ist wichtig zu beachten, dass diese Produkte nicht als Arzneimittel oder Medikamente eingestuft sind und keine Zulassung der FDA für die Vorbeugung, Behandlung oder Heilung von medizinischen Zuständen, Beschwerden oder Krankheiten erhalten haben. Wir müssen betonen, dass jede Form der körperlichen Einführung dieser Produkte in Menschen oder Tiere gesetzlich strikt untersagt ist. Es ist unerlässlich, sich an diese Richtlinien zu halten, um die Einhaltung rechtlicher und ethischer Standards in Forschung und Experiment zu gewährleisten.