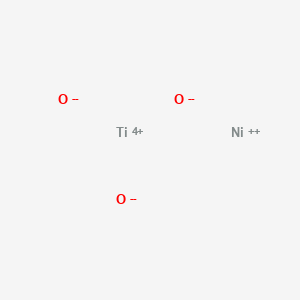
Nickel titanium oxide
Übersicht
Beschreibung
Nickel titanium oxide, also known as nickel(II) titanate, is an inorganic compound with the chemical formula NiTiO₃. It is a coordination compound between nickel(II), titanium(IV), and oxide ions. This compound typically appears as a yellow powder and is known for its unique structural and chemical properties .
Vorbereitungsmethoden
Synthetic Routes and Reaction Conditions: Nickel titanium oxide can be synthesized through several methods:
Solid-State Reaction: This involves heating a mixture of nickel oxide (NiO) and titanium dioxide (TiO₂) at high temperatures (around 1350°C) for several hours. The reaction can be represented as: [ \text{NiO} + \text{TiO}_2 \rightarrow \text{NiTiO}_3 ]
Polymeric Precursor Method: This method involves the spontaneous combustion of titanium isopropoxide, nickel nitrate hexahydrate, and glycine in isopropyl alcohol solution.
Industrial Production Methods: In industrial settings, this compound is often produced using high-temperature solid-state reactions due to the simplicity and scalability of the process. The raw materials, nickel oxide and titanium dioxide, are readily available and cost-effective, making this method suitable for large-scale production .
Analyse Chemischer Reaktionen
Nickel titanium oxide undergoes various chemical reactions, including:
Oxidation: It can act as a catalyst in oxidation reactions, such as the oxidation of toluene.
Reduction: this compound can be reduced under specific conditions, although this is less common.
Substitution: It can participate in substitution reactions where one of its components is replaced by another element or compound.
Common Reagents and Conditions:
Oxidation Reactions: Typically involve oxygen or other oxidizing agents at elevated temperatures.
Reduction Reactions: May involve hydrogen or other reducing agents under controlled conditions.
Major Products:
Oxidation: Produces oxidized organic compounds, such as benzaldehyde from toluene oxidation.
Reduction: Produces reduced forms of nickel and titanium oxides.
Wissenschaftliche Forschungsanwendungen
Nickel titanium oxide has a wide range of applications in scientific research:
Chemistry: Used as a catalyst in various chemical reactions, including oxidation and reduction processes.
Biology and Medicine: Investigated for its potential use in biomedical applications due to its biocompatibility and unique properties.
Industry: Utilized in the production of pigments, electronic materials, and as an electrode material in solid fuel cells and gas sensors
Wirkmechanismus
The mechanism by which nickel titanium oxide exerts its effects is primarily through its catalytic properties. The compound’s structure allows for efficient electron transfer, making it an effective catalyst. In applications such as sodium-ion batteries, its layered structure facilitates the intercalation of sodium ions, enhancing the battery’s performance .
Vergleich Mit ähnlichen Verbindungen
Nickel titanium oxide can be compared with other similar compounds such as:
Nickel Oxide (NiO): While both compounds contain nickel, nickel oxide lacks the titanium component, which imparts unique catalytic and structural properties to this compound.
Titanium Dioxide (TiO₂): Titanium dioxide is widely used as a photocatalyst and in pigments, but it does not possess the same catalytic properties as this compound when it comes to oxidation reactions.
Nickel-Titanium Alloys: These alloys are known for their shape memory properties, which are not present in this compound
This compound stands out due to its combination of nickel and titanium, which provides unique catalytic and electronic properties not found in its individual components.
Biologische Aktivität
Nickel titanium oxide (NiTiO₃), also known as nickel titanate, is an inorganic compound with unique properties that have garnered attention in various scientific fields, particularly in biomedicine and photocatalysis. This article explores the biological activity of NiTiO₃, focusing on its potential toxicity, biocompatibility, and applications in biomedical contexts.
NiTiO₃ crystallizes in an ilmenite structure characterized by rhombohedral symmetry. It consists of alternating layers of nickel and titanium ions interspersed with oxide ions. This structural arrangement contributes to its stability and reactivity under various conditions, making it a candidate for numerous applications, including photocatalysis and energy storage.
Toxicity and Biocompatibility
Research indicates that nickel compounds can pose significant health risks, including allergic reactions and potential carcinogenic effects upon inhalation. Specifically, NiTiO₃ has been associated with skin sensitization and other health issues related to nickel exposure. The following table summarizes key findings regarding the biological activity of NiTiO₃:
Study | Findings | Implications |
---|---|---|
Study 1 | Nickel exposure linked to allergic reactions | Potential risk for sensitization in humans |
Study 2 | Inhalation of nickel compounds raises carcinogenic concerns | Occupational safety measures needed |
Study 3 | NiTiO₃ exhibits low cytotoxicity in vitro | Suggests potential for safe biomedical applications |
Case Studies
- Skin Sensitization : A study highlighted the potential for NiTiO₃ to cause skin sensitization in laboratory animals. This finding necessitates careful handling and assessment of exposure risks in environments where NiTiO₃ is utilized.
- Biocompatibility Assessment : In vitro studies have demonstrated that NiTiO₃ exhibits low cytotoxicity toward various cell lines, suggesting its suitability for biomedical applications such as drug delivery systems or tissue engineering scaffolds .
- Photocatalytic Properties : NiTiO₃ has been investigated for its photocatalytic activity, which can be harnessed for environmental remediation processes. Its ability to degrade organic pollutants under UV light makes it a promising candidate for wastewater treatment applications .
The biological activity of NiTiO₃ is influenced by several factors:
- Particle Size : Smaller nanoparticles tend to exhibit enhanced biological activity due to their increased surface area and reactivity.
- Doping with Other Elements : Research shows that doping NiTiO₃ with other metals can improve its photocatalytic efficiency and reduce toxicity, thereby enhancing its applicability in various fields .
Research Findings
Recent studies have explored the interactions between NiTiO₃ and biological systems:
- Antimicrobial Activity : Some research indicates that nickel titanate may possess antimicrobial properties, although further studies are necessary to fully understand its efficacy against specific pathogens .
- Cellular Interactions : Investigations into how NiTiO₃ interacts with immune cells reveal that it may modulate immune responses, which could be beneficial or detrimental depending on the context of exposure .
Eigenschaften
IUPAC Name |
nickel(2+);oxygen(2-);titanium(4+) | |
---|---|---|
Source | PubChem | |
URL | https://pubchem.ncbi.nlm.nih.gov | |
Description | Data deposited in or computed by PubChem | |
InChI |
InChI=1S/Ni.3O.Ti/q+2;3*-2;+4 | |
Source | PubChem | |
URL | https://pubchem.ncbi.nlm.nih.gov | |
Description | Data deposited in or computed by PubChem | |
InChI Key |
KJLFZWJDCDJCFB-UHFFFAOYSA-N | |
Source | PubChem | |
URL | https://pubchem.ncbi.nlm.nih.gov | |
Description | Data deposited in or computed by PubChem | |
Canonical SMILES |
[O-2].[O-2].[O-2].[Ti+4].[Ni+2] | |
Source | PubChem | |
URL | https://pubchem.ncbi.nlm.nih.gov | |
Description | Data deposited in or computed by PubChem | |
Molecular Formula |
NiO3Ti | |
Source | PubChem | |
URL | https://pubchem.ncbi.nlm.nih.gov | |
Description | Data deposited in or computed by PubChem | |
Molecular Weight |
154.559 g/mol | |
Source | PubChem | |
URL | https://pubchem.ncbi.nlm.nih.gov | |
Description | Data deposited in or computed by PubChem | |
CAS No. |
12653-76-8 | |
Record name | Nickel titanium oxide | |
Source | ChemIDplus | |
URL | https://pubchem.ncbi.nlm.nih.gov/substance/?source=chemidplus&sourceid=0012653768 | |
Description | ChemIDplus is a free, web search system that provides access to the structure and nomenclature authority files used for the identification of chemical substances cited in National Library of Medicine (NLM) databases, including the TOXNET system. | |
Record name | Nickel titanium oxide | |
Source | EPA Chemicals under the TSCA | |
URL | https://www.epa.gov/chemicals-under-tsca | |
Description | EPA Chemicals under the Toxic Substances Control Act (TSCA) collection contains information on chemicals and their regulations under TSCA, including non-confidential content from the TSCA Chemical Substance Inventory and Chemical Data Reporting. | |
Record name | Nickel titanium oxide | |
Source | European Chemicals Agency (ECHA) | |
URL | https://echa.europa.eu/substance-information/-/substanceinfo/100.032.489 | |
Description | The European Chemicals Agency (ECHA) is an agency of the European Union which is the driving force among regulatory authorities in implementing the EU's groundbreaking chemicals legislation for the benefit of human health and the environment as well as for innovation and competitiveness. | |
Explanation | Use of the information, documents and data from the ECHA website is subject to the terms and conditions of this Legal Notice, and subject to other binding limitations provided for under applicable law, the information, documents and data made available on the ECHA website may be reproduced, distributed and/or used, totally or in part, for non-commercial purposes provided that ECHA is acknowledged as the source: "Source: European Chemicals Agency, http://echa.europa.eu/". Such acknowledgement must be included in each copy of the material. ECHA permits and encourages organisations and individuals to create links to the ECHA website under the following cumulative conditions: Links can only be made to webpages that provide a link to the Legal Notice page. | |
Haftungsausschluss und Informationen zu In-Vitro-Forschungsprodukten
Bitte beachten Sie, dass alle Artikel und Produktinformationen, die auf BenchChem präsentiert werden, ausschließlich zu Informationszwecken bestimmt sind. Die auf BenchChem zum Kauf angebotenen Produkte sind speziell für In-vitro-Studien konzipiert, die außerhalb lebender Organismen durchgeführt werden. In-vitro-Studien, abgeleitet von dem lateinischen Begriff "in Glas", beinhalten Experimente, die in kontrollierten Laborumgebungen unter Verwendung von Zellen oder Geweben durchgeführt werden. Es ist wichtig zu beachten, dass diese Produkte nicht als Arzneimittel oder Medikamente eingestuft sind und keine Zulassung der FDA für die Vorbeugung, Behandlung oder Heilung von medizinischen Zuständen, Beschwerden oder Krankheiten erhalten haben. Wir müssen betonen, dass jede Form der körperlichen Einführung dieser Produkte in Menschen oder Tiere gesetzlich strikt untersagt ist. Es ist unerlässlich, sich an diese Richtlinien zu halten, um die Einhaltung rechtlicher und ethischer Standards in Forschung und Experiment zu gewährleisten.