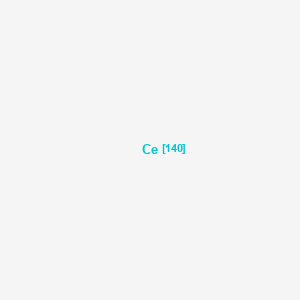
Cerium-140
Übersicht
Beschreibung
Cerium-140 is a stable isotope of the element cerium, which is part of the lanthanide series in the periodic table. Cerium is the most abundant of the rare-earth elements and is found in various minerals such as bastnasite and monazite. This compound constitutes approximately 88.45% of naturally occurring cerium . This isotope is notable for its applications in various scientific fields due to its unique properties.
Vorbereitungsmethoden
Synthetic Routes and Reaction Conditions: Cerium-140 can be isolated from cerium-containing minerals through a series of chemical processes. One common method involves the extraction of cerium from monazite sand using sulfuric acid, followed by the separation of cerium from other rare-earth elements through solvent extraction techniques .
Industrial Production Methods: In industrial settings, cerium is often produced by the reduction of cerium(III) fluoride with calcium at high temperatures. Another method involves the electrolysis of molten cerium chloride. These processes yield cerium metal, which can then be further purified to obtain this compound .
Analyse Chemischer Reaktionen
Types of Reactions: Cerium-140, like other cerium isotopes, undergoes various chemical reactions, including oxidation, reduction, and complexation.
Reduction: Cerium(IV) can be reduced to cerium(III) using reducing agents such as hydrogen or carbon monoxide.
Complexation: Cerium forms complexes with various ligands, which are used in different catalytic and industrial applications.
Common Reagents and Conditions:
Oxidation: Oxygen or air at elevated temperatures.
Reduction: Hydrogen gas or carbon monoxide at high temperatures.
Complexation: Various organic ligands under controlled pH and temperature conditions.
Major Products:
Oxidation: Cerium dioxide (CeO2).
Reduction: Cerium(III) compounds.
Complexation: Cerium-ligand complexes.
Wissenschaftliche Forschungsanwendungen
Cerium-140 has a wide range of applications in scientific research:
Chemistry: Used as a catalyst in organic synthesis and in the production of fine chemicals.
Biology: Employed in studies of cellular processes and as a tracer in biological systems.
Medicine: Investigated for its potential use in cancer treatment and as an antioxidant.
Industry: Utilized in the manufacturing of glass, ceramics, and as a polishing agent.
Wirkmechanismus
The mechanism by which cerium-140 exerts its effects is primarily through its redox properties. Cerium can switch between the +3 and +4 oxidation states, which allows it to participate in various redox reactions. This redox cycling is crucial in its role as a catalyst and in its biological applications, where it can neutralize free radicals and reduce oxidative stress .
Vergleich Mit ähnlichen Verbindungen
Cerium-138: Less abundant, used in specific nuclear applications.
Cerium-142: Also less abundant, with applications in nuclear science.
Cerium-140’s unique combination of abundance and chemical properties makes it particularly valuable for a wide range of applications.
Biologische Aktivität
Cerium-140 () is a radioactive isotope of cerium that has garnered attention for its potential biological activity, particularly in the context of nanomaterials and environmental exposure. This article delves into the biological effects of this compound, focusing on its interactions with biological systems, including antimicrobial properties, toxicity studies, and its behavior in living organisms.
Overview of this compound
Cerium is a lanthanide element that occurs in several isotopes, with being one of the more stable isotopes. It has applications in various fields including catalysis, materials science, and medical research. Its biological activity is primarily studied through cerium oxide nanoparticles (CeO NPs), which exhibit unique properties due to their size and surface characteristics.
Antimicrobial Activity
Recent studies have highlighted the antimicrobial properties of cerium oxide nanoparticles. These nanoparticles have been shown to exhibit significant inhibitory effects against various bacterial strains, particularly Gram-negative bacteria such as Escherichia coli and Pseudomonas aeruginosa.
Table 1: Antimicrobial Activity of Cerium Oxide Nanoparticles
Bacterial Strain | Minimum Inhibitory Concentration (MIC) | Method Used |
---|---|---|
E. coli | 2.58–3.44 mg/mL | Agar diffusion |
S. aureus | >50% inhibition at 2.58–3.44 mg/mL | Time-kill assay |
P. aeruginosa | Significant inhibition observed | Fluorescence assays |
The mechanism of action involves the generation of reactive oxygen species (ROS) that disrupt cellular processes, leading to cell death. The conversion between cerium's oxidation states (Ce to Ce) plays a crucial role in this antimicrobial activity, as it influences the oxidative stress experienced by bacterial cells .
Toxicity Studies
Toxicological assessments have been conducted to evaluate the effects of cerium exposure on various organisms. A notable study utilized Caenorhabditis elegans as a model organism to investigate the uptake and biological impact of cerium compounds.
Table 2: Toxicity Effects of Cerium Compounds on C. elegans
Exposure Type | Concentration Range (mg/L) | Observed Effects |
---|---|---|
CeO NPs | 0.5–34.96 | Low retention; minor growth inhibition |
Ce(NO) | 2.3–26 | Significant decrease in growth and fertility |
The study found that exposure to cerium nitrate resulted in severe tissue deformities and reduced reproductive success due to its systemic uptake and accumulation within specific tissues . Histological analyses indicated that cerium primarily accumulated in the pharyngeal valve and foregut, leading to adverse physiological effects .
Case Studies
- Antimicrobial Efficacy Against Opportunistic Pathogens : A series of experiments demonstrated that cerium oxide nanoparticles significantly inhibited the growth of opportunistic pathogens such as Staphylococcus aureus. The studies employed various methods including time-kill assays and agar diffusion tests, confirming that smaller particle sizes enhanced antimicrobial activity due to increased surface area for interaction with bacterial cells .
- Environmental Impact Assessment : Research involving environmental exposure scenarios revealed that cerium nanoparticles could enter aquatic ecosystems, leading to potential bioaccumulation in aquatic organisms. This raises concerns about long-term ecological impacts and necessitates further investigation into the fate of these nanoparticles in natural environments .
Eigenschaften
IUPAC Name |
cerium-140 | |
---|---|---|
Source | PubChem | |
URL | https://pubchem.ncbi.nlm.nih.gov | |
Description | Data deposited in or computed by PubChem | |
InChI |
InChI=1S/Ce/i1+0 | |
Source | PubChem | |
URL | https://pubchem.ncbi.nlm.nih.gov | |
Description | Data deposited in or computed by PubChem | |
InChI Key |
GWXLDORMOJMVQZ-IGMARMGPSA-N | |
Source | PubChem | |
URL | https://pubchem.ncbi.nlm.nih.gov | |
Description | Data deposited in or computed by PubChem | |
Canonical SMILES |
[Ce] | |
Source | PubChem | |
URL | https://pubchem.ncbi.nlm.nih.gov | |
Description | Data deposited in or computed by PubChem | |
Isomeric SMILES |
[140Ce] | |
Source | PubChem | |
URL | https://pubchem.ncbi.nlm.nih.gov | |
Description | Data deposited in or computed by PubChem | |
Molecular Formula |
Ce | |
Source | PubChem | |
URL | https://pubchem.ncbi.nlm.nih.gov | |
Description | Data deposited in or computed by PubChem | |
DSSTOX Substance ID |
DTXSID80931242 | |
Record name | (~140~Ce)Cerium | |
Source | EPA DSSTox | |
URL | https://comptox.epa.gov/dashboard/DTXSID80931242 | |
Description | DSSTox provides a high quality public chemistry resource for supporting improved predictive toxicology. | |
Molecular Weight |
139.90545 g/mol | |
Source | PubChem | |
URL | https://pubchem.ncbi.nlm.nih.gov | |
Description | Data deposited in or computed by PubChem | |
CAS No. |
14191-73-2 | |
Record name | Cerium, isotope of mass 140 | |
Source | ChemIDplus | |
URL | https://pubchem.ncbi.nlm.nih.gov/substance/?source=chemidplus&sourceid=0014191732 | |
Description | ChemIDplus is a free, web search system that provides access to the structure and nomenclature authority files used for the identification of chemical substances cited in National Library of Medicine (NLM) databases, including the TOXNET system. | |
Record name | (~140~Ce)Cerium | |
Source | EPA DSSTox | |
URL | https://comptox.epa.gov/dashboard/DTXSID80931242 | |
Description | DSSTox provides a high quality public chemistry resource for supporting improved predictive toxicology. | |
Haftungsausschluss und Informationen zu In-Vitro-Forschungsprodukten
Bitte beachten Sie, dass alle Artikel und Produktinformationen, die auf BenchChem präsentiert werden, ausschließlich zu Informationszwecken bestimmt sind. Die auf BenchChem zum Kauf angebotenen Produkte sind speziell für In-vitro-Studien konzipiert, die außerhalb lebender Organismen durchgeführt werden. In-vitro-Studien, abgeleitet von dem lateinischen Begriff "in Glas", beinhalten Experimente, die in kontrollierten Laborumgebungen unter Verwendung von Zellen oder Geweben durchgeführt werden. Es ist wichtig zu beachten, dass diese Produkte nicht als Arzneimittel oder Medikamente eingestuft sind und keine Zulassung der FDA für die Vorbeugung, Behandlung oder Heilung von medizinischen Zuständen, Beschwerden oder Krankheiten erhalten haben. Wir müssen betonen, dass jede Form der körperlichen Einführung dieser Produkte in Menschen oder Tiere gesetzlich strikt untersagt ist. Es ist unerlässlich, sich an diese Richtlinien zu halten, um die Einhaltung rechtlicher und ethischer Standards in Forschung und Experiment zu gewährleisten.