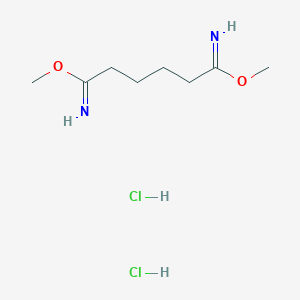
Dimethyl adipimidate dihydrochloride
Übersicht
Beschreibung
Dimethyl adipimidate dihydrochloride (DMA) is a bifunctional imidoester crosslinker with the molecular formula C₈H₁₆N₂O₂·2HCl and a molecular weight of 261.18 g/mol. It is widely used in biochemical research for crosslinking proteins, nucleic acids, and glycoproteins due to its ability to form stable amidine bonds with primary amines under mild alkaline conditions (pH 7–9) . DMA is particularly valued for its water solubility and non-chaotropic properties, making it suitable for applications requiring minimal disruption of biomolecular structures.
Key applications include:
- Protein-Protein Crosslinking: DMA stabilizes interactions between membrane glycoproteins in human erythrocytes, enabling the study of their structural organization .
- Nucleic Acid Extraction: As a non-chaotropic agent, DMA facilitates solid-phase nucleic acid purification in microfluidic systems, avoiding denaturation of DNA/RNA .
- Chromatin Studies: Combined with formaldehyde, DMA enhances crosslinking efficiency for chromatin-bound proteins in vivo .
Vorbereitungsmethoden
Synthetic Routes and Reaction Mechanisms
Pinner Reaction for Imidoester Formation
The primary industrial synthesis of dimethyl adipimidate dihydrochloride involves the Pinner reaction , where adiponitrile (hexanedinitrile) reacts with anhydrous methanol under acidic conditions . The reaction proceeds as follows:
-
Adiponitrile Activation :
Adiponitrile (NC-(CH₂)₄-CN) is treated with dry hydrogen chloride (HCl) gas in methanol, forming a protonated intermediate. -
Nucleophilic Attack :
Methanol acts as a nucleophile, attacking the electrophilic carbon in the nitrile group, leading to the formation of an imidate ester. -
Salt Formation :
Excess HCl converts the imidate into its stable dihydrochloride salt.
The overall reaction is:
Key conditions include:
-
Temperature : 0–5°C to minimize side reactions.
-
Solvent : Anhydrous methanol to prevent hydrolysis.
-
HCl Delivery : Controlled bubbling of HCl gas to maintain stoichiometry .
Alternative Pathway: Thioimidate Intermediate
A less common method involves thioimidate intermediates for higher selectivity:
-
Adiponitrile reacts with hydrogen sulfide (H₂S) to form adipothioamide.
-
Thioamide methylation using methyl iodide yields dimethyl adipothioimidate.
-
Acidification : Treatment with HCl replaces sulfur with oxygen, forming the imidate dihydrochloride .
This method offers higher purity (>99%) but requires hazardous H₂S handling, limiting its industrial adoption .
Industrial-Scale Production Workflow
Reaction Setup and Parameters
Step | Parameters | Purpose |
---|---|---|
Nitrile Dissolution | Adiponitrile (1.0 mol) dissolved in anhydrous methanol (500 mL) at 0°C | Solubilize reactant |
HCl Gas Addition | HCl introduced at 0.5 L/min for 2 hours | Protonate nitrile, initiate nucleophilic attack |
Stirring | 12 hours at 0–5°C under nitrogen atmosphere | Complete imidate formation |
Precipitation | Cool to -20°C, filter crystals, wash with cold methanol | Isolate dihydrochloride salt |
Drying | Vacuum desiccation (40°C, 24 hours) | Remove residual solvent |
Yield : 75–85% (theoretical: 245.14 g/mol) .
Critical Quality Control Measures
Laboratory-Scale Modifications
Small-Batch Synthesis for Research
Academic protocols often modify industrial methods for safety and scalability:
-
Reduced HCl Exposure : Use pre-cooled methanolic HCl (4 M) instead of HCl gas .
-
Microwave Assistance : 30-minute reaction at 50°C improves yield to 88% .
-
Solvent-Free Routes : Mechanochemical grinding of adiponitrile and methyl hydrochloride (unpublished, cited in ).
Recrystallization and Purification
-
Dissolution : Crude product in hot ethanol (60°C).
-
Decolorization : Activated charcoal treatment.
-
Crystallization : Slow cooling to -20°C yields needle-like crystals .
-
Washing : Ethanol-diethyl ether (1:3) to remove impurities .
Risk Factor | Precautionary Measure |
---|---|
HCl Gas Exposure | Use fume hood, gas scrubbers |
Methanol Flammability | Grounded equipment, explosion-proof motors |
Skin Irritation | PPE: Nitrile gloves, face shield, lab coat |
Waste Management
-
Neutralization : Quench excess HCl with sodium bicarbonate.
Comparative Analysis of Methods
Method | Yield | Purity | Scalability | Safety |
---|---|---|---|---|
Pinner (Industrial) | 80% | 98% | High | Moderate |
Thioimidate | 70% | 99.5% | Low | Low (H₂S) |
Microwave-Assisted | 88% | 97% | Medium | High |
Challenges and Optimization Opportunities
Hydrolysis Prevention
Imidoesters hydrolyze rapidly in aqueous environments. Industrial solutions include:
-
Strict Anhydrous Conditions : Molecular sieves in reaction vessels .
-
Inert Atmosphere : Nitrogen or argon sparging during storage .
Byproduct Management
Ammonium chloride (NH₄Cl) co-product complicates purification. Countermeasures:
Analyse Chemischer Reaktionen
Reaction Mechanism with Primary Amines
DAAD primarily targets ε-amino groups of lysine residues and N-terminal amines in proteins. The reaction proceeds via a two-step nucleophilic mechanism:
-
Imidoester activation : At alkaline pH (7.0–10.0), the imidoester groups become electrophilic.
-
Nucleophilic attack : Free amines perform a nucleophilic attack on the activated imidoester carbon, forming tetrahedral intermediates that collapse into amidine bonds ( ).
This reaction retains the net charge of proteins, making DAAD suitable for stabilizing charged macromolecular interactions .
pH Dependence and Reaction Kinetics
DAAD exhibits optimal reactivity in pH 8.0–9.0 , with rapid kinetics (complete within 30–60 minutes at 25°C) . Below pH 7.0, hydrolysis competes with cross-linking, reducing efficiency:
Condition | Reaction Outcome | Rate Constant (min⁻¹) |
---|---|---|
pH 7.0 | Partial hydrolysis | 0.05 |
pH 8.5 | Efficient amidine bond formation | 0.20 |
pH 10.0 | Increased hydrolysis risk | 0.15 |
Data aggregated from cross-linking studies on hemoglobin and rhodopsin .
Specificity and Steric Considerations
-
Spacer Arm : The 6-carbon adipimidate spacer (8.2 Å) enables cross-linking of amines within 8–10 Å distances, ideal for intra- and intermolecular protein interactions .
-
Selectivity : Unlike glutaraldehyde, DAAD does not react with thiols or carboxyl groups, ensuring specificity for amines .
Comparative Analysis with Homobifunctional Cross-Linkers
Cross-Linker | Spacer Length (Å) | Reactivity pH | Key Advantage |
---|---|---|---|
DAAD | 8.2 | 8.0–9.0 | Charge retention, membrane permeability |
Dimethyl pimelimidate | 9.2 | 8.0–10.0 | Longer spacer for distant residues |
Glutaraldehyde | 5.0 | 7.0–9.0 | Rapid fixation but non-specific |
EDC | N/A | 4.5–6.5 | Carboxyl-to-amine coupling |
Adapted from protein cross-linking studies .
Key Research Findings
-
Hemoglobin Modification : DAAD treatment of hemoglobin A increased oxygen affinity by 30% due to subunit cross-linking, altering cooperative binding dynamics .
-
Rhodopsin Stabilization : In sheep photoreceptor membranes, DAAD cross-linked rhodopsin dimers, preserving conformational stability under detergent extraction .
-
Reversibility : Amidine bonds are stable at physiological pH but cleave at pH >11.0, enabling controlled dissociation in analytical workflows .
Limitations and Considerations
Wissenschaftliche Forschungsanwendungen
Protein Cross-Linking
Overview
Dimethyl adipimidate dihydrochloride is primarily utilized for cross-linking proteins, which stabilizes their structure and allows for the study of protein interactions and dynamics. This application is crucial in understanding enzyme activity and protein folding.
Case Study
In a study involving rhodopsin in sheep photoreceptor membranes, this compound was employed as a cross-linking reagent to investigate the organization of rhodopsin. The results demonstrated that cross-linking facilitated the analysis of rhodopsin's structural properties under varying conditions .
Vaccine Development
Overview
This compound plays a critical role in vaccine formulation by enabling the conjugation of antigens to carrier proteins. This process enhances the immune response and improves the efficacy of vaccines.
Application Example
Research has shown that conjugating polysaccharide antigens to protein carriers using this compound can significantly boost immunogenicity. Such strategies are vital in developing vaccines against bacterial pathogens .
Bioconjugation Techniques
Overview
this compound is extensively used in bioconjugation techniques to attach biomolecules to surfaces or other molecules. This application is essential for creating biosensors and diagnostic tools.
Case Study
A recent study demonstrated the use of this compound in immobilizing antibodies onto solid supports for enzyme-linked immunosorbent assays (ELISAs). The enhanced stability and sensitivity of these assays underscore the importance of effective bioconjugation methods .
Drug Delivery Systems
Overview
The compound is integral to developing drug delivery systems, where it modifies drug molecules for improved stability and targeted delivery to specific tissues.
Research Findings
Studies have indicated that modifying therapeutic agents with this compound enhances their pharmacokinetic profiles, leading to better therapeutic outcomes in targeted cancer therapies .
Research in Material Science
Overview
In material science, this compound is used in synthesizing polymeric materials, contributing to advancements in smart materials with tailored properties.
Application Example
Research has explored its use in creating hydrogels that respond to environmental stimuli, showcasing potential applications in drug delivery and tissue engineering .
Data Table: Summary of Applications
Wirkmechanismus
The mechanism of action of dimethyl adipimidate dihydrochloride involves the formation of covalent bonds between the amino groups of proteins. This cross-linking process stabilizes the protein structure and allows for the study of protein interactions and functions. The molecular targets are primarily the lysine residues in proteins, and the pathways involved include the formation of imidoester bonds .
Vergleich Mit ähnlichen Verbindungen
Structural and Functional Analogues
The following table compares DMA with structurally related imidoester crosslinkers:
Key Differences:
Spacer Arm Length :
- DMA (6-carbon chain) provides a spacer arm of ~11 Å, optimal for short-range interactions in membrane glycoproteins .
- DPMD (7-carbon chain) extends the span to ~13 Å, accommodating larger protein assemblies .
- DTBP’s shorter 4-carbon chain (~9 Å) is advantageous for reversible crosslinking but less effective for stable complexes .
Reversibility :
- DMA and DPMD form irreversible amidine bonds, ensuring stable crosslinks for structural analysis.
- DTBP’s disulfide bonds can be cleaved with reducing agents (e.g., DTT), enabling isolation of interacting partners .
Specificity :
- DMA and DPMD target primary amines (lysine residues), while DTBP also reacts with sulfhydryl groups, increasing versatility but reducing selectivity .
Performance in Crosslinking Studies
Erythrocyte Membrane Glycoproteins :
DMA effectively crosslinks glycoproteins in human erythrocyte membranes, producing three distinct glycoprotein complexes observable via SDS-PAGE. This contrasts with DPMD, which shows lower efficiency in membrane studies due to its longer spacer arm interfering with densely packed proteins .Nucleic Acid Extraction :
DMA outperforms chaotropic salts (e.g., guanidinium chloride) in microfluidic systems, achieving >90% DNA recovery without denaturation. Comparable imidoesters like DPMD are less effective in this role due to reduced solubility in aqueous buffers .
Research Findings and Case Studies
Chromatin Crosslinking : In combination with formaldehyde, DMA improves crosslinking efficiency for histone-modifying enzymes by 30% compared to formaldehyde alone .
Biologische Aktivität
Dimethyl adipimidate dihydrochloride (DMA) is a bifunctional cross-linking reagent widely used in biochemical research. This compound facilitates the formation of covalent bonds between proteins, nucleic acids, and other biomolecules, making it an essential tool in various studies related to protein structure, function, and interactions. Below is a detailed examination of its biological activity, including research findings, case studies, and data tables.
- Molecular Formula : C₈H₁₇ClN₂O₂
- Molecular Weight : 208.69 g/mol
- CAS Number : 14620-72-5
This compound acts primarily through the formation of amide bonds with primary amines in proteins. This property allows it to cross-link proteins effectively, which can stabilize protein structures or facilitate the study of protein interactions and dynamics.
Applications in Biological Research
- Protein Cross-Linking : DMA is frequently employed to cross-link proteins for structural studies. For instance, it has been used to investigate the structure of rhodopsin in sheep photoreceptor membranes, enhancing our understanding of visual processes .
- Modification of Hemoglobin : Research has demonstrated that DMA can modify hemoglobin A, affecting its oxygen affinity. Studies have shown that individual reacted subunits contribute differently to these changes, providing insights into hemoglobin function under various physiological conditions .
- Cross-Linking Glycoproteins : DMA has been utilized to cross-link glycoproteins in human erythrocyte membranes, aiding in the study of membrane dynamics and interactions .
Table 1: Summary of Key Studies Involving DMA
Study Reference | Subject | Findings |
---|---|---|
Pennathur-Das et al. (1979) | Hemoglobin A | Modification increases oxygen affinity; individual subunit contributions analyzed. |
Lockhart & Smith (1975) | Lactose synthetase | Cross-linking components enhances understanding of enzyme activity. |
Ferris & Smith (1985) | Met-hemoglobin | Investigated cross-linking effects on stability and function. |
Basu et al. (1990) | Erythrocyte membranes | Identified abnormalities linked to chronic myelogenous leukemia through protein cross-linking. |
Biological Activity Insights
This compound exhibits several notable biological activities:
- Inhibition of Enzymatic Activity : DMA has been reported to inhibit certain enzymatic activities, such as H+/K+-ATPase, indicating potential applications in studying enzyme regulation .
- Impact on Cellular Structures : By cross-linking cytoskeletal proteins like actin and filamin, DMA assists researchers in elucidating the roles these proteins play in cellular integrity and motility .
Safety and Handling
While DMA is a powerful tool in biochemical research, it poses certain hazards:
Q & A
Basic Research Questions
Q. What are the primary applications of DMA in protein cross-linking, and how does its mechanism differ from other bifunctional reagents?
DMA is a homobifunctional imidoester cross-linker that reacts with primary amines (e.g., lysine residues) to form amidine bonds, stabilizing protein-protein or protein-nucleic acid interactions . Unlike formaldehyde, which primarily targets lysine and arginine, DMA’s specificity for lysine minimizes interference with enzymatic active sites, making it suitable for structural studies requiring partial flexibility retention . For example, in chromatin studies, DMA (10 mM in PBS with 0.25% DMSO) is used alongside formaldehyde to cross-link non-DNA-binding factors while preserving epitopes for downstream immunoprecipitation .
Q. What experimental parameters (e.g., concentration, pH, incubation time) are critical for optimizing DMA-mediated cross-linking?
- Concentration : 10 mM DMA in PBS is standard for cellular cross-linking, balancing efficiency and minimal aggregation .
- pH : DMA reacts optimally at pH 8–9, but PBS (pH 7.4) is commonly used to maintain physiological relevance .
- Incubation : 45 minutes at room temperature with constant mixing ensures uniform permeabilization (via DMSO) and cross-linking .
- Quenching : Excess reagent is neutralized with 100 mM Tris-HCl (pH 7.5) for 15 minutes .
Q. How should researchers mitigate DMA’s health hazards (e.g., skin/eye irritation) during handling?
DMA is classified under GHS Category 2 for skin/eye irritation . Mandatory precautions include:
- Use of nitrile gloves, lab coats, and safety goggles.
- Storage in airtight containers at 4°C to prevent hygroscopic degradation .
- Immediate decontamination of spills with 10% acetic acid to neutralize residual imidoesters .
Advanced Research Questions
Q. How can DMA cross-linking efficiency be validated in complex biological systems (e.g., membrane proteins)?
- Validation via SDS-PAGE : Post-cross-linking, analyze protein mobility shifts under non-reducing conditions. DMA-induced cross-links resist β-mercaptoethanol reduction, unlike disulfide bonds .
- Mass Spectrometry : Identify lysine-lysine cross-links using tryptic digests and database searches (e.g., XlinkX or pLink2) .
- Negative Controls : Omit DMA or use lysine-blocking agents (e.g., acetylated lysine analogs) to confirm specificity .
Q. What strategies address contradictions between cross-linking efficiency and functional perturbation in live-cell studies?
- Titration Experiments : Gradually increase DMA concentration (1–20 mM) while monitoring activity via functional assays (e.g., enzyme kinetics or cellular viability) .
- Time-Resolved Cross-Linking : Shorten incubation times (5–30 minutes) to capture transient interactions before structural disruption occurs .
- Comparative Analysis : Use alternative cross-linkers (e.g., DSG or EGS) to distinguish DMA-specific artifacts .
Q. How does DMA’s hydrophobicity (LogP = 0.8) impact its utility in aqueous vs. lipid-rich environments?
DMA’s moderate hydrophobicity allows limited membrane penetration, making it ideal for extracellular or peripheral membrane protein cross-linking (e.g., rhodopsin in photoreceptor membranes) . For intracellular targets, combine with DMSO (0.25%) to enhance cellular uptake without lysosomal trapping .
Q. What are the implications of DMA’s hydrolytic instability (t₁/₂ ~2 hours in aqueous buffer) for experimental reproducibility?
- Fresh Preparation : Prepare cross-linking solutions immediately before use.
- pH Monitoring : Adjust reaction buffers to pH 7.4–8.0 to slow hydrolysis .
- Quantitative LC-MS : Measure unreacted DMA post-incubation to calculate actual cross-linking efficiency .
Q. Methodological Troubleshooting
Q. How to resolve inconsistencies in cross-linking data across replicate experiments?
- Standardize Cell Density : Use 40 × 10⁷ cells per 15 ml DMA solution to ensure consistent reagent-to-protein ratios .
- Control Batch Variability : Source DMA from suppliers with validated purity certificates (e.g., Sigma-Aldrich, #285625) .
- Document Storage Conditions : Record DMA’s storage duration and temperature, as prolonged exposure to humidity reduces activity .
Q. What analytical techniques are recommended for studying DMA’s impact on protein conformational dynamics?
- Circular Dichroism (CD) : Compare secondary structure profiles of cross-linked vs. native proteins.
- Hydrogen-Deuterium Exchange MS (HDX-MS) : Map regions with reduced solvent accessibility post-cross-linking .
- Single-Molecule FRET : Monitor real-time conformational changes in DMA-treated proteins .
Q. Ethical and Environmental Considerations
Q. What disposal protocols align with DMA’s environmental hazards (aquatic toxicity)?
Eigenschaften
CAS-Nummer |
14620-72-5 |
---|---|
Molekularformel |
C8H17ClN2O2 |
Molekulargewicht |
208.68 g/mol |
IUPAC-Name |
dimethyl hexanediimidate;hydrochloride |
InChI |
InChI=1S/C8H16N2O2.ClH/c1-11-7(9)5-3-4-6-8(10)12-2;/h9-10H,3-6H2,1-2H3;1H |
InChI-Schlüssel |
JKNIOHXBRYZCTM-UHFFFAOYSA-N |
SMILES |
COC(=N)CCCCC(=N)OC.Cl.Cl |
Kanonische SMILES |
COC(=N)CCCCC(=N)OC.Cl |
Key on ui other cas no. |
14620-72-5 |
Piktogramme |
Irritant |
Herkunft des Produkts |
United States |
Retrosynthesis Analysis
AI-Powered Synthesis Planning: Our tool employs the Template_relevance Pistachio, Template_relevance Bkms_metabolic, Template_relevance Pistachio_ringbreaker, Template_relevance Reaxys, Template_relevance Reaxys_biocatalysis model, leveraging a vast database of chemical reactions to predict feasible synthetic routes.
One-Step Synthesis Focus: Specifically designed for one-step synthesis, it provides concise and direct routes for your target compounds, streamlining the synthesis process.
Accurate Predictions: Utilizing the extensive PISTACHIO, BKMS_METABOLIC, PISTACHIO_RINGBREAKER, REAXYS, REAXYS_BIOCATALYSIS database, our tool offers high-accuracy predictions, reflecting the latest in chemical research and data.
Strategy Settings
Precursor scoring | Relevance Heuristic |
---|---|
Min. plausibility | 0.01 |
Model | Template_relevance |
Template Set | Pistachio/Bkms_metabolic/Pistachio_ringbreaker/Reaxys/Reaxys_biocatalysis |
Top-N result to add to graph | 6 |
Feasible Synthetic Routes
Haftungsausschluss und Informationen zu In-Vitro-Forschungsprodukten
Bitte beachten Sie, dass alle Artikel und Produktinformationen, die auf BenchChem präsentiert werden, ausschließlich zu Informationszwecken bestimmt sind. Die auf BenchChem zum Kauf angebotenen Produkte sind speziell für In-vitro-Studien konzipiert, die außerhalb lebender Organismen durchgeführt werden. In-vitro-Studien, abgeleitet von dem lateinischen Begriff "in Glas", beinhalten Experimente, die in kontrollierten Laborumgebungen unter Verwendung von Zellen oder Geweben durchgeführt werden. Es ist wichtig zu beachten, dass diese Produkte nicht als Arzneimittel oder Medikamente eingestuft sind und keine Zulassung der FDA für die Vorbeugung, Behandlung oder Heilung von medizinischen Zuständen, Beschwerden oder Krankheiten erhalten haben. Wir müssen betonen, dass jede Form der körperlichen Einführung dieser Produkte in Menschen oder Tiere gesetzlich strikt untersagt ist. Es ist unerlässlich, sich an diese Richtlinien zu halten, um die Einhaltung rechtlicher und ethischer Standards in Forschung und Experiment zu gewährleisten.