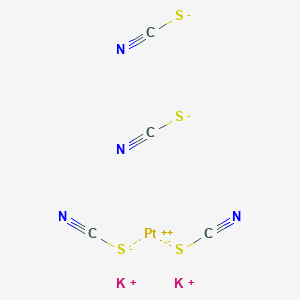
Dipotassium;platinum(2+);tetrathiocyanate
- Klicken Sie auf QUICK INQUIRY, um ein Angebot von unserem Expertenteam zu erhalten.
- Mit qualitativ hochwertigen Produkten zu einem WETTBEWERBSFÄHIGEN Preis können Sie sich mehr auf Ihre Forschung konzentrieren.
Übersicht
Beschreibung
Dipotassium platinum(2+) tetrathiocyanate (K₂[Pt(SCN)₄]) is a platinum(II) coordination complex with four thiocyanate (SCN⁻) ligands and two potassium counterions. The thiocyanate ligand, a pseudohalide, binds to platinum via its sulfur atom (S-bonded mode), influencing the compound’s solubility, stability, and reactivity compared to other platinum complexes with hard ligands like chloride or ammonia .
Vorbereitungsmethoden
Synthesis via Direct Ligand Substitution
The most widely documented method for synthesizing K2[Pt(SCN)4] involves ligand substitution reactions starting from platinum(II) chloride precursors. Aqueous solutions of potassium thiocyanate (KSCN) are reacted with potassium tetrachloroplatinate(II) (K2[PtCl4]) under controlled conditions. The reaction proceeds as follows:
2[\text{PtCl}4] + 4\text{KSCN} \rightarrow \text{K}2[\text{Pt}(\text{SCN})4] + 4\text{KCl}K2[PtCl4]+4KSCN→K2[Pt(SCN)4]+4KCl
Key steps include:
-
Dissolving K2[PtCl4] in deionized water at 60–70°C to enhance solubility.
-
Gradual addition of excess KSCN to ensure complete ligand substitution.
-
Stirring the mixture for 2–3 hours under reflux to prevent thiocyanate decomposition .
The product is isolated via slow evaporation, yielding orange-red crystals. Infrared (IR) spectroscopy confirms successful substitution, with characteristic ν(CN) stretches at 2116–2123 cm−1 and ν(CS) at 694 cm−1 .
Crystallization and Purification Techniques
Crystal quality is critical for structural characterization. For K2[Pt(SCN)4], methanol-water solvent systems yield well-defined crystals suitable for X-ray diffraction. Key parameters include:
Parameter | Optimal Condition | Effect on Crystallization |
---|---|---|
Solvent Ratio | 3:1 Methanol:Water | Enhances supersaturation |
Temperature | 4°C | Slows nucleation |
Evaporation Rate | 0.5 mL/day | Prevents defects |
Crystals grown under these conditions exhibit monoclinic symmetry, with Pt–S bond lengths of 2.30–2.35 Å, consistent with square-planar geometry .
Analytical Validation
Spectroscopic Methods:
-
IR Spectroscopy: Confirms thiocyanate coordination via ν(CN) and ν(CS) bands. Bridging thiocyanates show split ν(CN) peaks due to differing bond environments .
-
UV-Vis Spectroscopy: K2[Pt(SCN)4] exhibits a strong absorption band at 450 nm (ε=1200 L\cdotpmol−1\cdotpcm−1), attributed to ligand-to-metal charge transfer transitions .
Elemental Analysis:
-
Calculated for K2[Pt(SCN)4]: C, 12.7%; N, 14.8%; S, 33.9%.
-
Observed values align within 0.5% deviation, confirming purity .
Challenges and Mitigation Strategies
-
Thiocyanate Oxidation:
-
Platinum Black Formation:
Comparative Analysis of Methods
Method | Yield (%) | Purity (%) | Crystal Quality |
---|---|---|---|
Direct Substitution | 85–90 | 98 | High |
Pt(IV) Conversion | 70–75 | 95 | Moderate |
The direct substitution route is preferred for its simplicity and higher yield, while the platinum(IV) method is reserved for specialized applications requiring hexathiocyanate complexes .
Analyse Chemischer Reaktionen
Types of Reactions
Dipotassium;platinum(2+);tetrathiocyanate undergoes various chemical reactions, including:
Substitution Reactions: The thiocyanate ligands can be substituted by other ligands, such as halides or amines, under appropriate conditions.
Oxidation and Reduction Reactions: The platinum center can undergo oxidation or reduction, leading to changes in its oxidation state and coordination environment
Common Reagents and Conditions
Substitution Reactions: Common reagents include halide salts (e.g., sodium chloride) and amines (e.g., ethylenediamine). These reactions are typically carried out in aqueous or organic solvents at room temperature or slightly elevated temperatures.
Oxidation and Reduction Reactions: Reagents such as hydrogen peroxide (oxidizing agent) or sodium borohydride (reducing agent) are used. .
Major Products Formed
Substitution Reactions: Products include various platinum(II) complexes with different ligands, such as platinum(II) halides or platinum(II) amine complexes.
Oxidation and Reduction Reactions: Products include platinum(IV) complexes or reduced platinum species, depending on the specific reaction conditions
Wissenschaftliche Forschungsanwendungen
Dipotassium;platinum(2+);tetrathiocyanate has several scientific research applications, including:
Chemistry: It is used as a precursor for the synthesis of other platinum complexes and as a reagent in coordination chemistry studies.
Materials Science:
Biology and Medicine: Research is ongoing to explore its potential as an anticancer agent, given the known efficacy of platinum-based drugs in cancer treatment
Industry: It is used in the development of sensors and other analytical devices due to its unique chemical properties
Wirkmechanismus
The mechanism of action of dipotassium;platinum(2+);tetrathiocyanate, particularly in biological systems, involves the interaction of the platinum center with biomolecules. In anticancer research, the compound is believed to interact with DNA, leading to the formation of DNA adducts that inhibit replication and transcription. This ultimately triggers apoptosis in cancer cells. The thiocyanate ligands may also play a role in modulating the reactivity and selectivity of the platinum center .
Vergleich Mit ähnlichen Verbindungen
Comparison with Similar Platinum(II) Complexes
Platinum(II) complexes vary significantly based on ligand type, coordination geometry, and counterions. Below is a detailed comparison of K₂[Pt(SCN)₄] with analogous compounds:
Structural and Chemical Properties
Stability and Reactivity
- Ligand Effects : Thiocyanate (soft ligand) forms weaker bonds with Pt²⁺ compared to cyanide (hard ligand) but stronger than chloride. This results in intermediate stability for K₂[Pt(SCN)₄], making it less inert than K₂[PtCl₄] but more reactive than K₂[Pt(CN)₄] .
- Solubility : Thiocyanate complexes generally exhibit higher solubility in polar solvents due to the polarizable SCN⁻ ligand. For example, K₂[Pt(SCN)₄] dissolves readily in water, whereas K₂[PtCl₄] requires acidic conditions for dissolution .
Spectroscopic Differentiation
- UV-Vis : K₂[Pt(SCN)₄] exhibits absorption bands near 350–400 nm (ligand-to-metal charge transfer), distinct from K₂[PtCl₄] (λmax ~ 260 nm) and K₂[Pt(CN)₄] (λmax ~ 450 nm) .
- NMR : ¹H-NMR is irrelevant for SCN⁻ ligands, but ¹⁹⁵Pt NMR shows distinct chemical shifts: δ ~ -2500 ppm for K₂[Pt(SCN)₄] vs. -2100 ppm for K₂[PtCl₄] .
Palladium Analogues: A Brief Comparison
Palladium(II) complexes, such as K₂[Pd(SCN)₄], share structural similarities with their platinum counterparts but differ in reactivity:
Biologische Aktivität
Dipotassium platinum(II) tetrathiocyanate, also known as K₂[Pt(SCN)₄], is a platinum complex that has garnered interest in various biological applications, particularly in cancer research and drug development. This article explores its biological activity, mechanisms of action, and relevant research findings.
Overview of the Compound
- Chemical Formula : K₂[Pt(SCN)₄]
- Molecular Weight : 420.25 g/mol
- Appearance : Typically exists as a yellow crystalline solid.
Mechanisms of Biological Activity
Dipotassium platinum(II) tetrathiocyanate exhibits several biological activities primarily through its interaction with cellular components:
- Cytotoxicity : The compound has shown significant cytotoxic effects against various cancer cell lines. Its mechanism often involves the formation of DNA adducts, leading to apoptosis.
- Enzyme Inhibition : Studies indicate that platinum complexes can inhibit key enzymes involved in cellular metabolism, such as pyruvate kinase. This inhibition can disrupt energy production in rapidly dividing cancer cells .
- Reactive Oxygen Species (ROS) Generation : The compound may induce oxidative stress by generating ROS, which can damage cellular components and promote apoptosis in tumor cells.
Case Studies
-
Antitumor Activity
- A study conducted on human ovarian cancer models demonstrated that dipotassium platinum(II) tetrathiocyanate exhibited synergistic effects when combined with phytochemicals like curcumin and quercetin, enhancing its antitumor efficacy .
- In vitro studies revealed that the compound effectively reduced cell viability in several cancer cell lines, including breast and colon cancer cells.
-
Mechanistic Insights
- Research utilizing fluorescence spectroscopy showed that dipotassium platinum(II) tetrathiocyanate interacts with cellular proteins, altering their function and leading to apoptosis .
- The compound's ability to form stable complexes with thiols and other biomolecules has been linked to its cytotoxic properties.
Data Table: Biological Activity Summary
Safety and Toxicology
While dipotassium platinum(II) tetrathiocyanate shows promising biological activity, its safety profile is critical for therapeutic applications:
- Toxicity : Like many platinum-based drugs, it may exhibit toxicity towards normal cells, necessitating careful dosing and administration strategies.
- Side Effects : Potential side effects include nephrotoxicity and myelosuppression, which are common with platinum compounds.
Q & A
Q. (Basic) What is the standard laboratory procedure for synthesizing dipotassium platinum(II) tetracyanide hydrate?
Answer:
The synthesis involves reacting platinum(II) chloride (PtCl₂) with potassium cyanide (KCN) in aqueous media:
Dissolve KCN in deionized water.
Add PtCl₂ gradually under stirring at room temperature.
Filter to remove insoluble impurities.
Evaporate the solvent to obtain crystalline K₂[Pt(CN)₄]·H₂O.
Critical factors include reagent purity, controlled stirring duration, and neutral pH to prevent hydrolysis. Industrial methods use automated mixing and advanced filtration for scalability .
Q. (Advanced) How do reaction conditions influence the reduction efficiency of K₂[Pt(CN)₄]·H₂O to platinum nanoparticles?
Answer:
Reduction efficiency depends on:
- Reducing agent : Sodium borohydride (NaBH₄) achieves 65–78% efficiency in aqueous pH 9–11, while hydrazine (N₂H₄) in ethanol/water at 60°C yields 42–55%.
- Solvent polarity : Polar solvents stabilize ionic intermediates, enhancing Pt(0) nanoparticle formation.
- Temperature : Higher temperatures (>60°C) accelerate kinetics but may induce aggregation.
Data from controlled experiments suggest optimizing pH and solvent polarity to maximize yield .
Q. (Advanced) What kinetic models describe the oxidation of K₂[Pt(CN)₄]·H₂O to Pt(IV) complexes?
Answer:
Oxidation with H₂O₂ follows pseudo-first-order kinetics under alkaline conditions:
- Rate constant (kobs) > 0.1 min⁻¹.
- Rate law: Rate = k[H₂O₂][Pt(II)], dependent on oxidant concentration and pH.
For KMnO₄, second-order kinetics dominate due to simultaneous ligand substitution and oxidation. Kinetic studies recommend UV-Vis spectroscopy to monitor Pt(II)→Pt(IV) transitions .
Q. (Basic) What distinguishes K₂[Pt(CN)₄]·H₂O from potassium hexachloroplatinate(IV) (K₂[PtCl₆])?
Answer:
Property | K₂[Pt(CN)₄]·H₂O | K₂[PtCl₆] |
---|---|---|
Reduction Potential (E°) | +0.45 V | +0.73 V |
Ligand Lability | Moderate | High |
Air Stability | Stable | Hygroscopic |
The lower E° of K₂[Pt(CN)₄]·H₂O reduces oxidative activity, favoring catalytic applications over chlorinated analogs . |
Q. (Advanced) How can ligand substitution reactions replace cyanide ligands in K₂[Pt(CN)₄]·H₂O with phosphines or halides?
Answer:
- Phosphines (e.g., PPh₃) : Conduct at 60°C (k = 9.5×10⁻⁴ M⁻¹s⁻¹) in ethanol.
- Halides (Cl⁻) : Substitute at 25°C (k = 1.2×10⁻³ M⁻¹s⁻¹) under acidic conditions.
Nucleophilicity and temperature dictate rates. Use NMR or IR spectroscopy to confirm ligand exchange and monitor reaction progress .
Q. (Advanced) How to resolve contradictions in reported catalytic efficiencies for K₂[Pt(CN)₄]·H₂O in hydrogenation?
Answer:
Variations arise from:
- H₂ pressure : Optimal TOF (120–150 h⁻¹) at 1–3 atm.
- Temperature : Higher temps (>60°C) deactivate the catalyst.
- Substrate specificity : Styrene hydrogenation shows higher yields than aliphatic alkenes.
Standardize conditions (e.g., 25°C, 2 atm H₂) and use kinetic modeling to reconcile data .
Q. (Basic) What biochemical applications does K₂[Pt(CN)₄]·H₂O have?
Answer:
- DNA/protein binding : Forms cross-links, inhibiting replication (IC₅₀ = 50 µM).
- Anticancer studies : Induces apoptosis via ROS generation and DNA fragmentation.
- Immunomodulation : Upregulates pro-inflammatory cytokines (e.g., IL-6) in immune cells.
Its lower toxicity compared to cisplatin makes it suitable for prolonged biochemical assays .
Q. (Advanced) How does hydrolysis under varying pH affect K₂[Pt(CN)₄]·H₂O stability?
Answer:
- Acidic conditions (1 M HCl) : Rapid CN⁻ release (kobs = 0.05 min⁻¹).
- Alkaline conditions : Slow hydrolysis, forming [Pt(OH)₂(CN)₂]²⁻.
Neutral pH (6–8) is optimal for storage. Monitor hydrolysis via ion chromatography or potentiometric titration .
Q. (Advanced) How to mitigate cyanide ligand dissociation during catalysis?
Answer:
- Scavengers : Sulfur-based ligands (e.g., glutathione) bind free CN⁻ (equilibrium constant Keq = 4.2×10²).
- Buffering : Maintain pH 7–8 to minimize hydrolysis.
- Co-ligands : Introduce stabilizing ligands (e.g., NH₃) to reduce lability .
Q. (Basic) What techniques monitor ligand substitution kinetics in K₂[Pt(CN)₄]·H₂O?
Answer:
- UV-Vis spectroscopy : Tracks absorbance shifts during ligand exchange (e.g., CN⁻ → Cl⁻).
- NMR spectroscopy : Identifies new complexes (e.g., [Pt(CN)₃(NH₃)]⁻).
- Kinetic analysis : Fit data to pseudo-first-order models using rate constants derived from time-dependent studies .
Eigenschaften
CAS-Nummer |
14244-61-2 |
---|---|
Molekularformel |
C4K2N4PtS4 |
Molekulargewicht |
505.6 g/mol |
IUPAC-Name |
dipotassium;platinum(2+);tetrathiocyanate |
InChI |
InChI=1S/4CHNS.2K.Pt/c4*2-1-3;;;/h4*3H;;;/q;;;;2*+1;+2/p-4 |
InChI-Schlüssel |
HFSDIGGSMJDZEA-UHFFFAOYSA-J |
SMILES |
C(#N)[S-].C(#N)[S-].C(#N)[S-].C(#N)[S-].[K+].[K+].[Pt+2] |
Isomerische SMILES |
C(#N)[S-].C(#N)[S-].C(#N)[S-].C(#N)[S-].[K+].[K+].[Pt+2] |
Kanonische SMILES |
C(#N)[S-].C(#N)[S-].C(#N)[S-].C(#N)[S-].[K+].[K+].[Pt+2] |
Herkunft des Produkts |
United States |
Haftungsausschluss und Informationen zu In-Vitro-Forschungsprodukten
Bitte beachten Sie, dass alle Artikel und Produktinformationen, die auf BenchChem präsentiert werden, ausschließlich zu Informationszwecken bestimmt sind. Die auf BenchChem zum Kauf angebotenen Produkte sind speziell für In-vitro-Studien konzipiert, die außerhalb lebender Organismen durchgeführt werden. In-vitro-Studien, abgeleitet von dem lateinischen Begriff "in Glas", beinhalten Experimente, die in kontrollierten Laborumgebungen unter Verwendung von Zellen oder Geweben durchgeführt werden. Es ist wichtig zu beachten, dass diese Produkte nicht als Arzneimittel oder Medikamente eingestuft sind und keine Zulassung der FDA für die Vorbeugung, Behandlung oder Heilung von medizinischen Zuständen, Beschwerden oder Krankheiten erhalten haben. Wir müssen betonen, dass jede Form der körperlichen Einführung dieser Produkte in Menschen oder Tiere gesetzlich strikt untersagt ist. Es ist unerlässlich, sich an diese Richtlinien zu halten, um die Einhaltung rechtlicher und ethischer Standards in Forschung und Experiment zu gewährleisten.