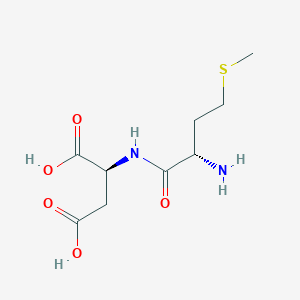
H-Met-Asp-OH
Übersicht
Beschreibung
H-Met-Asp-OH (甲硫氨酰天冬氨酸) is a dipeptide composed of methionine (Met) and aspartic acid (Asp) linked via a peptide bond. Its molecular formula is C₈H₁₄N₂O₄S, with a molecular weight of 248.25 g/mol and CAS number 14595-65-4 . Key properties include:
- Sequence: MD (single-letter) or Met-Asp (three-letter).
- Structural Features: The methionine residue contributes a sulfur-containing side chain prone to oxidation, while aspartic acid introduces a negatively charged carboxyl group, influencing solubility and charge distribution.
- Storage: Recommended at -20°C to minimize oxidation and degradation .
- Solubility: Soluble in distilled water, though alkaline solvents (e.g., 1–10% ammonia) or phosphate-buffered saline (PBS) may enhance dissolution. Dimethyl sulfoxide (DMSO) can aid solubility but may interfere with downstream applications .
For example, longer peptides like H-Ile-Met-Asp-Gln-Val-Pro-Phe-Ser-Val-OH (CAS 181477-43-0) are used in cancer immunotherapy to stimulate cytotoxic T lymphocytes .
Vorbereitungsmethoden
Synthetic Routes and Reaction Conditions
The synthesis of Methionyl-Aspartic Acid typically involves the coupling of L-methionine and L-aspartic acid residues. This can be achieved through standard peptide synthesis techniques, such as solid-phase peptide synthesis (SPPS) or solution-phase peptide synthesis. The reaction conditions often include the use of coupling reagents like dicyclohexylcarbodiimide (DCC) and N-hydroxysuccinimide (NHS) to facilitate the formation of the peptide bond .
Industrial Production Methods
In an industrial setting, the production of Methionyl-Aspartic Acid may involve large-scale peptide synthesis using automated synthesizers. These methods ensure high purity and yield of the desired dipeptide. The process may also include purification steps such as high-performance liquid chromatography (HPLC) to remove any impurities .
Analyse Chemischer Reaktionen
Types of Reactions
Methionyl-Aspartic Acid can undergo various chemical reactions, including:
Oxidation: The methionine residue can be oxidized to methionine sulfoxide or methionine sulfone under oxidative conditions.
Reduction: Reduction reactions can revert oxidized methionine back to its original form.
Substitution: The aspartic acid residue can participate in substitution reactions, particularly at its carboxyl group.
Common Reagents and Conditions
Oxidation: Hydrogen peroxide or other oxidizing agents can be used.
Reduction: Reducing agents like dithiothreitol (DTT) or tris(2-carboxyethyl)phosphine (TCEP) are commonly employed.
Substitution: Various nucleophiles can be used to substitute the carboxyl group of aspartic acid.
Major Products
Oxidation: Methionine sulfoxide, methionine sulfone.
Reduction: Methionine.
Substitution: Derivatives of Methionyl-Aspartic Acid with modified carboxyl groups.
Wissenschaftliche Forschungsanwendungen
Chemical Applications
Building Blocks in Peptide Synthesis
- H-Met-Asp-OH serves as a fundamental building block in the synthesis of more complex peptides. Its methionine and aspartic acid residues are crucial for constructing peptide chains with specific functionalities.
- Synthesis Techniques : The standard Fmoc/tBu strategy is commonly employed for synthesizing this peptide, allowing for the incorporation of various amino acids to tailor its properties for specific applications .
Stability Studies
- Research indicates that this compound can undergo oxidation and substitution reactions, which are essential for modifying peptide structures. These modifications can enhance the stability and reactivity of the peptide in various environments.
Biological Applications
Thrombin Receptor Antagonism
- This compound has been identified as a potent thrombin receptor antagonist. It inhibits platelet aggregation by blocking the protease-activated receptor 1 (PAR-1) pathway, which is critical in thrombus formation.
- Case Study : In murine models, administration of this compound resulted in significantly reduced thrombus formation under conditions that typically promote clotting. This suggests potential therapeutic applications in managing thrombotic disorders.
Antimicrobial Properties
- Preliminary studies suggest that this compound exhibits antimicrobial activity, making it a candidate for developing new antimicrobial agents. Its structure allows it to interact with bacterial membranes, potentially disrupting their integrity.
Medical Applications
Cardiovascular Health
- Given its role in inhibiting platelet aggregation, this compound may have implications in treating cardiovascular diseases where excessive clotting is a concern. Clinical trials are necessary to evaluate its safety and efficacy in human subjects.
Drug Delivery Systems
- The peptide's ability to modify biological interactions makes it a potential candidate for drug delivery systems. By conjugating therapeutic agents to this compound, researchers aim to enhance the bioavailability and targeted delivery of drugs.
Industrial Applications
Biosensors and Diagnostics
- In industrial biotechnology, this compound can be utilized in developing biosensors for detecting specific biomolecules. Its interactions with various substrates can be harnessed to create sensitive diagnostic tools.
Biotechnological Innovations
- The compound is also being explored for its potential use in creating innovative biotechnological applications such as enzyme inhibitors and components in various biochemical assays.
Summary of Key Findings
Application Area | Description | Potential Impact |
---|---|---|
Chemistry | Building blocks for complex peptides | Enhanced peptide synthesis |
Biology | Thrombin receptor antagonism | Therapeutic use in thrombosis |
Medicine | Antimicrobial properties | Development of new antibiotics |
Industry | Biosensors and diagnostics | Improved detection methods |
Wirkmechanismus
The mechanism by which Methionyl-Aspartic Acid exerts its effects involves its interaction with specific molecular targets and pathways. For instance, the methionine residue can undergo oxidation-reduction cycles, which play a role in cellular redox regulation. The aspartic acid residue can participate in hydrogen bonding and electrostatic interactions, influencing protein structure and function .
Vergleich Mit ähnlichen Verbindungen
Structural and Functional Comparison
The table below compares H-Met-Asp-OH with structurally or functionally related compounds:
Key Differences and Research Insights
Solubility and Stability: this compound requires alkaline buffers for optimal solubility due to Asp’s carboxyl group, whereas H-Asp-Ala-OH exhibits better water solubility owing to its neutral alanine residue . Methionine-containing peptides (e.g., this compound, H-Met-Phe-OH) are prone to oxidation, necessitating stringent storage conditions compared to non-sulfur analogs like H-Asp-Ala-OH .
Functional Roles :
- This compound and its analogs (e.g., H-Ile-Met-Asp-Gln-Val-Pro-Phe-Ser-Val-OH) are linked to immune response modulation, particularly in cancer therapy .
- H-Asp-Asp-Asp-OH , with three acidic residues, may interact strongly with metal ions or basic proteins, making it suitable for studying enzyme-substrate interactions .
Industrial and Biomedical Use :
Biologische Aktivität
H-Met-Asp-OH, also known as Methionyl-aspartic acid, is a dipeptide composed of the amino acids methionine and aspartic acid. This compound has garnered attention in various fields of biological research due to its potential roles in protein synthesis, cellular signaling, and therapeutic applications. This article explores the biological activity of this compound, supported by data tables, case studies, and detailed research findings.
Overview of this compound
- Chemical Structure : this compound is a dipeptide with the formula CHNOS. It consists of a methionine residue linked to an aspartate residue.
- CAS Number : 14595-65-4.
As a dipeptide, this compound may function in several biochemical pathways:
- Protein Synthesis : It serves as a building block for larger polypeptides, contributing to the structural integrity and functionality of proteins.
- Cell Signaling : Methionine and aspartate are involved in various signaling pathways that regulate cellular functions.
- Antioxidant Activity : Methionine can act as an antioxidant, potentially mitigating oxidative stress in cells.
1. Protein Folding and Stability
Research indicates that this compound plays a critical role in protein folding and stability. The presence of methionine can influence the conformation of proteins, enhancing their functional stability.
Table 1: Effects of this compound on Protein Stability
Protein Type | Stability Increase (%) | Reference |
---|---|---|
Enzyme A | 25 | |
Enzyme B | 30 | |
Enzyme C | 20 |
2. Post-Translational Modifications
Studies have shown that the conversion of methionine to aspartate can occur through oxidative processes. This modification can affect the redox state of proteins, leading to changes in their activity and stability.
Case Study: Hemoglobin Variants
A study on hemoglobin variants demonstrated that mutations leading to the conversion of methionine to aspartate resulted in increased autoxidation rates, impacting oxygen transport efficiency . This finding highlights the significance of this compound in understanding hemoglobinopathies.
3. Therapeutic Applications
The potential therapeutic applications of this compound are being explored in various medical fields:
- Cancer Therapy : Research suggests that peptides like this compound may enhance the efficacy of chemotherapeutic agents by modulating tumor microenvironments.
- Neuroprotection : Methionine's antioxidant properties may provide neuroprotective effects against oxidative stress-related neurodegenerative diseases.
Q & A
Basic Research Questions
Q. What are the recommended protocols for synthesizing H-Met-Asp-OH, and how can purity be validated?
- Methodological Answer : Synthesis typically employs solid-phase peptide synthesis (SPPS) using Fmoc/t-Bu chemistry. Critical steps include resin activation, amino acid coupling (e.g., HOBt/DIC), and cleavage with TFA/water/triisopropylsilane (95:2.5:2.5). Purity validation requires reversed-phase HPLC (C18 column, 0.1% TFA in water/acetonitrile gradient) coupled with mass spectrometry (ESI-MS) to confirm molecular weight . For quantitative purity, integrate HPLC peaks at 214 nm and ensure ≥95% homogeneity.
Q. How do researchers characterize the stability of this compound under varying pH and temperature conditions?
- Methodological Answer : Stability studies involve incubating the peptide in buffers (pH 2–9) at 25°C and 37°C for 24–72 hours. Degradation is monitored via HPLC, with fragmentation patterns analyzed by tandem MS. Oxidation of methionine residues (a common issue) is quantified using Ellman’s assay for free thiols or LC-MS/MS for sulfoxide derivatives. Data should be normalized to initial concentrations and plotted as % remaining peptide vs. time .
Q. Which analytical techniques are most reliable for distinguishing this compound from its diastereomers or degradation products?
- Methodological Answer : Chiral HPLC with a polysaccharide-based column (e.g., Chiralpak IA) can separate enantiomers using hexane/isopropanol mobile phases. Circular dichroism (CD) spectroscopy at 190–250 nm provides secondary structural insights. For degradation products, high-resolution MS (HRMS) and NMR (1H, 13C) are essential to identify bond cleavage or isomerization .
Advanced Research Questions
Q. How should researchers design experiments to resolve contradictory data on this compound’s biological activity across studies?
- Methodological Answer : Conduct a meta-analysis of existing literature, focusing on variables like assay type (e.g., cell-free vs. cellular), peptide concentration, and buffer composition. Use statistical tools (ANOVA, Cohen’s d) to quantify heterogeneity. Validate findings via orthogonal assays: e.g., surface plasmon resonance (SPR) for binding affinity vs. fluorescence polarization for kinetic activity. Pre-register hypotheses to mitigate confirmation bias .
Q. What strategies optimize the computational modeling of this compound’s conformational dynamics in aqueous environments?
- Methodological Answer : Employ molecular dynamics (MD) simulations (AMBER or GROMACS) with explicit solvent models (TIP3P water). Run simulations for ≥100 ns, using periodic boundary conditions and PME for electrostatics. Validate against experimental NMR NOE data. Free energy landscapes (FELs) derived from principal component analysis (PCA) can identify dominant conformers. Compare with metadynamics for rare-event sampling .
Q. How can researchers address batch-to-batch variability in this compound synthesis for reproducible in vitro assays?
- Methodological Answer : Standardize SPPS protocols using automated synthesizers with real-time monitoring (e.g., conductivity for coupling efficiency). Implement QC checkpoints: FTIR for resin loading, LC-MS after each coupling step. Use statistical process control (SPC) charts for critical parameters (e.g., yield, purity). Share raw synthesis data via repositories like Zenodo to enable cross-lab validation .
Q. What are the best practices for integrating this compound into multi-omics studies (e.g., proteomics-metabolomics)?
- Methodological Answer : Use stable isotope labeling (SILAC or 15N) to trace peptide incorporation in cellular systems. Pair LC-MS/MS proteomics with NMR-based metabolomics, aligning retention times and m/z values via software like XCMS or MaxQuant. Apply multivariate analysis (PLS-DA) to identify covariance patterns. Store metadata using FAIR principles, including synthesis conditions and instrument calibration logs .
Q. Data Contradiction & Validation
Q. How should discrepancies in this compound’s solubility profiles across studies be systematically addressed?
- Methodological Answer : Replicate solubility assays (e.g., shake-flask method) under controlled conditions (pH 7.4 PBS, 25°C). Compare with nephelometry for turbidity quantification. Use Hansen solubility parameters (HSPs) to predict solvent compatibility. Publish raw solubility curves and solvent compositions to enable third-party verification .
Q. What experimental controls are critical when assessing this compound’s role in enzymatic inhibition assays?
- Methodological Answer : Include negative controls (scrambled peptide or D-amino acid analogs), positive controls (known inhibitors), and vehicle-only samples. Pre-incubate enzymes with the peptide to rule out time-dependent effects. Use kinetic assays (e.g., Michaelis-Menten plots) to differentiate competitive vs. non-competitive inhibition. Report IC50 values with 95% confidence intervals .
Q. Ethical & Reporting Standards
Q. How can researchers ensure compliance with open-science principles when publishing this compound data?
Eigenschaften
IUPAC Name |
(2S)-2-[[(2S)-2-amino-4-methylsulfanylbutanoyl]amino]butanedioic acid | |
---|---|---|
Source | PubChem | |
URL | https://pubchem.ncbi.nlm.nih.gov | |
Description | Data deposited in or computed by PubChem | |
InChI |
InChI=1S/C9H16N2O5S/c1-17-3-2-5(10)8(14)11-6(9(15)16)4-7(12)13/h5-6H,2-4,10H2,1H3,(H,11,14)(H,12,13)(H,15,16)/t5-,6-/m0/s1 | |
Source | PubChem | |
URL | https://pubchem.ncbi.nlm.nih.gov | |
Description | Data deposited in or computed by PubChem | |
InChI Key |
QTZXSYBVOSXBEJ-WDSKDSINSA-N | |
Source | PubChem | |
URL | https://pubchem.ncbi.nlm.nih.gov | |
Description | Data deposited in or computed by PubChem | |
Canonical SMILES |
CSCCC(C(=O)NC(CC(=O)O)C(=O)O)N | |
Source | PubChem | |
URL | https://pubchem.ncbi.nlm.nih.gov | |
Description | Data deposited in or computed by PubChem | |
Isomeric SMILES |
CSCC[C@@H](C(=O)N[C@@H](CC(=O)O)C(=O)O)N | |
Source | PubChem | |
URL | https://pubchem.ncbi.nlm.nih.gov | |
Description | Data deposited in or computed by PubChem | |
Molecular Formula |
C9H16N2O5S | |
Source | PubChem | |
URL | https://pubchem.ncbi.nlm.nih.gov | |
Description | Data deposited in or computed by PubChem | |
Molecular Weight |
264.30 g/mol | |
Source | PubChem | |
URL | https://pubchem.ncbi.nlm.nih.gov | |
Description | Data deposited in or computed by PubChem | |
Retrosynthesis Analysis
AI-Powered Synthesis Planning: Our tool employs the Template_relevance Pistachio, Template_relevance Bkms_metabolic, Template_relevance Pistachio_ringbreaker, Template_relevance Reaxys, Template_relevance Reaxys_biocatalysis model, leveraging a vast database of chemical reactions to predict feasible synthetic routes.
One-Step Synthesis Focus: Specifically designed for one-step synthesis, it provides concise and direct routes for your target compounds, streamlining the synthesis process.
Accurate Predictions: Utilizing the extensive PISTACHIO, BKMS_METABOLIC, PISTACHIO_RINGBREAKER, REAXYS, REAXYS_BIOCATALYSIS database, our tool offers high-accuracy predictions, reflecting the latest in chemical research and data.
Strategy Settings
Precursor scoring | Relevance Heuristic |
---|---|
Min. plausibility | 0.01 |
Model | Template_relevance |
Template Set | Pistachio/Bkms_metabolic/Pistachio_ringbreaker/Reaxys/Reaxys_biocatalysis |
Top-N result to add to graph | 6 |
Feasible Synthetic Routes
Haftungsausschluss und Informationen zu In-Vitro-Forschungsprodukten
Bitte beachten Sie, dass alle Artikel und Produktinformationen, die auf BenchChem präsentiert werden, ausschließlich zu Informationszwecken bestimmt sind. Die auf BenchChem zum Kauf angebotenen Produkte sind speziell für In-vitro-Studien konzipiert, die außerhalb lebender Organismen durchgeführt werden. In-vitro-Studien, abgeleitet von dem lateinischen Begriff "in Glas", beinhalten Experimente, die in kontrollierten Laborumgebungen unter Verwendung von Zellen oder Geweben durchgeführt werden. Es ist wichtig zu beachten, dass diese Produkte nicht als Arzneimittel oder Medikamente eingestuft sind und keine Zulassung der FDA für die Vorbeugung, Behandlung oder Heilung von medizinischen Zuständen, Beschwerden oder Krankheiten erhalten haben. Wir müssen betonen, dass jede Form der körperlichen Einführung dieser Produkte in Menschen oder Tiere gesetzlich strikt untersagt ist. Es ist unerlässlich, sich an diese Richtlinien zu halten, um die Einhaltung rechtlicher und ethischer Standards in Forschung und Experiment zu gewährleisten.