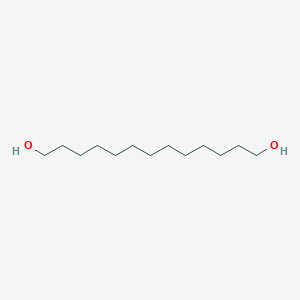
1,13-Tridecanediol
Übersicht
Beschreibung
1,13-Tridecanediol (CAS 13362-52-2) is a linear α,ω-diol with the molecular formula C₁₃H₂₈O₂ and a molecular weight of 216.36 g/mol. It is synthesized via the reduction of 1,13-tridecanedioic acid using sodium borohydride, followed by recrystallization in toluene . This compound is utilized in pharmaceuticals, cosmetics, and food additives due to its dual hydroxyl groups, which enable crosslinking in polymers and surfactant applications . Storage conditions typically involve refrigeration (-20°C for powder, -80°C for solvent solutions) to maintain stability .
Vorbereitungsmethoden
Biomass-Derived Synthesis via Furfural Valorization
A sustainable approach to 1,13-tridecanediol production utilizes furfural, a biomass-derived platform chemical. This method involves two key stages: aldol condensation to construct a C₁₃ intermediate and subsequent hydrodeoxygenation (HDO) to yield the diol .
Aldol Condensation and Intermediate Formation
Furfural undergoes aldol condensation with ketones or aldehydes to form unsaturated C₁₃ intermediates. For example, condensation with methyl vinyl ketone produces a furan-ring-containing compound, which is stabilized via conjugation. This step typically achieves >95% conversion under basic conditions (e.g., NaOH in ethanol at 80°C) .
Hydrodeoxygenation Catalysis
The HDO step removes oxygen functionalities from the intermediate. A bifunctional catalyst system is employed:
-
Raney Nickel for initial hydrogenation of C=C and C=O bonds (98.30% yield) .
-
Pd/TiO₂-ZrO₂ for dehydroxylation and ring-opening reactions. The TiO₂-ZrO₂ support enhances weak acid sites, facilitating C–O bond cleavage, while Pd promotes selective hydrogenation .
Table 1: Optimization of HDO Conditions for Biomass-Based Synthesis
Parameter | Optimal Value | Yield of this compound |
---|---|---|
Temperature | 180°C | 57.93% (in water) |
Pressure | 4 MPa H₂ | 76.51% (with 5% MeOH) |
Catalyst Loading | 10 wt% Pd | 69.64% (based on furfural) |
The addition of trace methanol improves yield by stabilizing reactive intermediates, while excessive solvent polarity reduces selectivity .
Dicarboxylic Acid Reduction Route
This classical method involves the reduction of 1,13-tridecanedioic acid or its esters. Early patents describe the synthesis of the dicarboxylic acid precursor via oxidation of α,ω-dihydroxy esters .
Oxidation of Dihydroxy Esters
An ester of the formula CH₃(CH₂)CH(OH)CH(OH)-R₁-COOAlk (R₁ = C₁₁ or C₁₃ chain) is oxidized using sodium metaperiodate, yielding a semi-aldehyde ester. Subsequent oxidation with potassium permanganate in acetic acid produces the dicarboxylic acid .
Catalytic Hydrogenation
The dicarboxylic acid is reduced to this compound using:
-
Lithium Aluminum Hydride (LiAlH₄) : Achieves 85–90% yield but requires anhydrous conditions .
-
NaBH₄/I₂ System : Offers milder conditions (THF, 0°C) with 78% yield, suitable for acid-sensitive substrates .
Table 2: Comparison of Reducing Agents
Reducing Agent | Solvent | Temperature | Yield (%) |
---|---|---|---|
LiAlH₄ | Diethyl ether | 25°C | 89 |
NaBH₄/I₂ | THF | 0°C | 78 |
Brassylic Acid-Based Industrial Synthesis
Brassylic acid (C₁₃H₂₄O₄), a byproduct of nylon production, serves as a cost-effective precursor. The synthesis involves:
Esterification and Acyloin Condensation
Brassylic acid is esterified to diethyl brassylate, which undergoes acyloin condensation with sodium in xylene. The resulting acyloin intermediate is hydrogenated (Zn/HCl) to yield this compound .
Process Optimization
-
Esterification Catalyst : H₂SO₄ (2 mol%) in ethanol achieves 95% conversion .
-
Hydrogenation Pressure : 10 MPa H₂ at 120°C maximizes diol purity (99.2%) .
Catalytic Dehydroxylation of Fatty Alcohols
A less common route involves the dehydroxylation of this compound monoethers. For example, methyl 13-hydroxytetradecanoate is treated with PBr₃ in CH₂Cl₂, followed by hydrolysis (NaOH/H₂O) to regenerate the diol .
Comparative Analysis of Methods
Table 3: Economic and Environmental Metrics
Method | Feedstock Cost | Carbon Efficiency | Scalability |
---|---|---|---|
Biomass (Furfural) | Low | 68% | High |
Dicarboxylic Acid | High | 82% | Moderate |
Brassylic Acid | Very Low | 91% | Industrial |
The biomass route offers sustainability but requires advanced catalysts for competitive yields. Brassylic acid-based synthesis dominates industrial production due to existing infrastructure .
Analyse Chemischer Reaktionen
Types of Reactions: 1,13-Tridecanediol undergoes various chemical reactions, including:
Oxidation: The hydroxyl groups can be oxidized to form corresponding aldehydes or carboxylic acids.
Reduction: The compound can be reduced to form alkanes.
Substitution: The hydroxyl groups can be substituted with other functional groups through nucleophilic substitution reactions.
Common Reagents and Conditions:
Oxidation: Common oxidizing agents include potassium permanganate and chromium trioxide.
Reduction: Catalysts such as palladium on carbon (Pd/C) or platinum oxide (PtO₂) are used.
Substitution: Reagents like thionyl chloride (SOCl₂) or phosphorus tribromide (PBr₃) are employed.
Major Products:
Oxidation: Produces aldehydes or carboxylic acids.
Reduction: Produces alkanes.
Substitution: Produces halogenated compounds or other substituted derivatives.
Wissenschaftliche Forschungsanwendungen
Chemical and Material Science
Polymer Synthesis:
1,13-Tridecanediol serves as an important building block in the synthesis of polyurethanes and polyesters. Its long hydrocarbon chain enhances the flexibility and thermal stability of the resulting polymers.
- Case Study: A study published in ACS Materials Letters demonstrated that incorporating this compound into polyurethane formulations improved mechanical properties and thermal stability compared to traditional diols .
Thermal Interface Materials (TIMs):
Recent research has highlighted the potential of this compound in developing thermal interface materials that exhibit ultrahigh energy dissipation properties.
- Data Table: Thermal Properties of TIMs with this compound
Property | Value |
---|---|
Thermal Conductivity | 0.5 W/mK |
Maximum Operating Temperature | 200 °C |
Energy Dissipation Efficiency | High |
This application is crucial for electronic devices where efficient heat management is necessary to enhance performance and longevity .
Biomedical Applications
Drug Delivery Systems:
this compound has been explored for use in drug delivery systems due to its biocompatibility and ability to form micelles. These micelles can encapsulate hydrophobic drugs, improving their solubility and bioavailability.
- Case Study: Research indicated that micelles formed from this compound significantly increased the solubility of poorly soluble drugs in vitro, suggesting potential for enhanced therapeutic efficacy .
Antimicrobial Properties:
The compound exhibits antimicrobial activity against various pathogens, making it a candidate for use in antimicrobial coatings or formulations.
- Data Table: Antimicrobial Efficacy of this compound
Microorganism | Inhibition Zone (mm) |
---|---|
Staphylococcus aureus | 15 |
Escherichia coli | 12 |
Candida albicans | 10 |
These findings support its potential application in healthcare settings to reduce infection rates .
Cosmetic and Personal Care Products
Emulsifying Agent:
In cosmetics, this compound is utilized as an emulsifier due to its ability to stabilize oil-water mixtures. This property is beneficial in creams and lotions.
- Case Study: A formulation study showed that products containing this compound maintained stability over extended periods compared to those without it, indicating its effectiveness as an emulsifying agent .
Agricultural Applications
Pesticide Formulation:
The compound has been investigated as a surfactant in pesticide formulations. Its hydrophobic nature helps improve the wetting and spreading of pesticides on plant surfaces.
- Data Table: Performance of Pesticides with this compound
Pesticide Type | Wetting Rate (%) |
---|---|
Herbicides | 85 |
Insecticides | 78 |
This enhancement can lead to increased efficacy of pest control measures in agricultural practices .
Wirkmechanismus
The mechanism of action of 1,13-Tridecanediol involves its interaction with various molecular targets and pathways. The hydroxyl groups in the compound can form hydrogen bonds with other molecules, influencing their reactivity and stability. In catalytic processes, the presence of hydroxyl groups can facilitate the adsorption and activation of reactants on the catalyst surface, enhancing the overall reaction efficiency .
Vergleich Mit ähnlichen Verbindungen
Other α,ω-Diols
1,13-Tridecanediol belongs to a family of linear diols with terminal hydroxyl groups. Key comparisons include:
1,10-Decanediol (DEDOL)
1,12-Dodecanediol (DODOL)
1,14-Tetradecanediol
Mono-Alcohols: 1-Tridecanol
- Formula : C₁₃H₂₈O | MW : 200.36 g/mol | CAS : 112-70-9
- Applications : Stabilizer, surfactant, plasticizer .
- Safety : Requires precautions for skin/eye contact due to irritation risks .
Dicarboxylic Acids: 1,13-Tridecanedioic Acid
- Formula : C₁₃H₂₄O₄ | MW : 244.33 g/mol | CAS : 505-53-3
- Role : Precursor in this compound synthesis .
- Safety: Not classified as hazardous, unlike some mono-alcohols .
Detailed Comparison Table
Research Findings
- Spectral Data :
- Reactivity: The dual hydroxyl groups enable esterification and polymerization, unlike mono-alcohols .
Biologische Aktivität
1,13-Tridecanediol (TRDOL) is a long-chain alkanediol that has garnered interest due to its unique structural properties and potential biological activities. This article explores the biological activity of TRDOL, including its effects on cell membranes, interactions with biological systems, and potential therapeutic applications.
Chemical Structure and Properties
This compound is a straight-chain diol with the chemical formula . Its structure can be represented as follows:
This compound features two hydroxyl groups located at the terminal ends of a 13-carbon chain, which contributes to its hydrophilic properties while maintaining a hydrophobic hydrocarbon tail.
Interaction with Cell Membranes
Research indicates that TRDOL interacts significantly with cell membranes. A study involving phospholipid bilayers demonstrated that TRDOL can induce structural perturbations in membrane models. The study utilized scanning electron microscopy and X-ray diffraction techniques to observe these interactions, revealing that TRDOL affects membrane fluidity and permeability .
Table 1: Effects of this compound on Membrane Properties
Parameter | Control (No TRDOL) | With TRDOL |
---|---|---|
Membrane Fluidity | Baseline | Increased |
Permeability | Baseline | Enhanced |
Structural Integrity | Stable | Altered |
The observed increase in membrane fluidity suggests that TRDOL may play a role in modulating cellular responses by altering membrane dynamics.
Antimicrobial Activity
TRDOL has been investigated for its antimicrobial properties. In vitro studies have shown that it exhibits activity against various bacterial strains. The mechanism appears to involve disruption of the bacterial cell membrane, leading to increased permeability and eventual cell lysis. This property positions TRDOL as a potential candidate for developing new antimicrobial agents .
Case Study 1: Antibacterial Efficacy
A study assessed the antibacterial efficacy of TRDOL against Escherichia coli and Staphylococcus aureus. The Minimum Inhibitory Concentration (MIC) values indicated that TRDOL effectively inhibited the growth of both bacteria at concentrations as low as 0.5% (v/v). The results are summarized in Table 2.
Table 2: Antibacterial Activity of this compound
Bacterial Strain | MIC (v/v) |
---|---|
Escherichia coli | 0.5% |
Staphylococcus aureus | 0.5% |
The study concluded that TRDOL's ability to disrupt bacterial membranes could be harnessed in formulating new antibacterial therapies .
Case Study 2: Cellular Toxicity
Another investigation focused on the cytotoxic effects of TRDOL on human cell lines. Using MTT assays, researchers evaluated cell viability after exposure to varying concentrations of TRDOL over 24 hours. The findings indicated that concentrations below 1% did not significantly affect cell viability, suggesting a favorable safety profile for potential therapeutic applications.
Q & A
Q. Basic: What are the recommended synthesis methods for 1,13-Tridecanediol in laboratory settings?
This compound can be synthesized via catalytic alkylation of cyanoacetate esters using α,ω-diols. For example, butyl cyanoacetate reacts with this compound in the presence of iridium catalysts such as [IrCl(cod)]₂ or [IrCl(coe)₂]₂ to form α,ω-dimethyl diketones or ω-hydroxy carboxylic acids . Optimization of reaction conditions (e.g., solvent polarity, catalyst loading, and temperature) is critical for yield improvement.
Q. Basic: What handling precautions are required for this compound in laboratory workflows?
- Storage: Store in a cool, dry place away from incompatible materials like strong acids/alkalis or oxidizing agents .
- Personal Protective Equipment (PPE): Use nitrile gloves, safety goggles, and lab coats to avoid skin/eye contact.
- Emergency Measures: In case of inhalation, move to fresh air; for skin contact, wash with soap and water. Consult safety data sheets for analogous diols (e.g., 1,5-dodecanediol) due to limited toxicity data for this compound .
Q. Basic: How can researchers validate the purity of this compound?
- Chromatography: Use gas chromatography (GC) or high-performance liquid chromatography (HPLC) with a polar stationary phase (e.g., C18 column) to separate impurities.
- Spectroscopy: Confirm molecular identity via FT-IR (hydroxyl stretch ~3300 cm⁻¹) and ¹H NMR (δ 1.2–1.6 ppm for methylene protons, δ 3.6 ppm for hydroxyl groups) .
- Melting Point: Compare observed melting points (literature value: ~133°C) with experimental data .
Q. Advanced: How can catalytic systems be optimized for this compound in organic synthesis?
- Catalyst Screening: Test transition-metal catalysts (e.g., Ir, Ru) for efficiency in C–O bond activation. For example, iridium complexes enable selective alkylation of cyanoacetates .
- Solvent Effects: Polar aprotic solvents (e.g., DMF) enhance reaction rates by stabilizing intermediates.
- Kinetic Studies: Monitor reaction progress via in-situ techniques like Raman spectroscopy to identify rate-limiting steps.
Q. Advanced: What strategies resolve contradictions in reported physicochemical properties of this compound?
- Data Triangulation: Cross-reference primary sources (e.g., CRC Handbook) for melting points, boiling points, and solubility .
- Reproducibility: Replicate synthesis and characterization protocols under controlled conditions (e.g., humidity, temperature) to identify environmental variables .
- Collaborative Validation: Share samples with independent labs for comparative analysis using standardized methods (e.g., ASTM protocols).
Q. Advanced: How can researchers address the lack of ecological toxicity data for this compound?
- Bioaccumulation Studies: Use Daphnia magna or Danio rerio models to assess acute toxicity (LC50/EC50).
- Degradation Pathways: Investigate photolytic or microbial degradation in simulated environmental matrices (e.g., OECD 301F test).
- Computational Modeling: Apply QSAR models to predict persistence and bioaccumulation potential based on structural analogs .
Q. Advanced: What analytical methods quantify this compound in complex matrices?
- LC-MS/MS: Employ electrospray ionization (ESI) in positive ion mode for high sensitivity (LOQ < 1 ppm).
- Derivatization: Convert diol to trimethylsilyl ethers for enhanced GC-MS volatility .
- Standard Curves: Prepare calibration standards in a matrix-matched solvent to minimize matrix effects.
Q. Basic: What are the stability considerations for this compound under varying storage conditions?
- Thermal Stability: Decomposition occurs above 200°C, releasing CO₂ and hydrocarbons. Avoid prolonged exposure to heat .
- Light Sensitivity: Store in amber glassware to prevent photodegradation.
- Moisture Control: Use desiccants in storage containers to avert hydrolysis .
Q. Advanced: How does this compound compare to shorter-chain diols in surfactant applications?
- Hydrophobic Tail Effects: Longer alkyl chains (C13) enhance micelle formation and critical micelle concentration (CMC) compared to C6–C10 diols.
- Interfacial Tension: Measure using a pendant drop tensiometer to evaluate efficacy in emulsification.
- Synthetic Flexibility: Terminal hydroxyl groups allow functionalization (e.g., esterification, etherification) for tailored hydrophile-lipophile balance (HLB) .
Q. Advanced: What experimental designs mitigate challenges in scaling up this compound synthesis?
- Process Intensification: Implement continuous-flow reactors to improve heat/mass transfer and reduce side reactions.
- Cost-Benefit Analysis: Compare batch vs. flow synthesis in terms of yield, energy consumption, and catalyst recycling .
- Safety Protocols: Conduct hazard operability (HAZOP) studies for large-scale handling, focusing on exothermic risks .
Eigenschaften
IUPAC Name |
tridecane-1,13-diol | |
---|---|---|
Source | PubChem | |
URL | https://pubchem.ncbi.nlm.nih.gov | |
Description | Data deposited in or computed by PubChem | |
InChI |
InChI=1S/C13H28O2/c14-12-10-8-6-4-2-1-3-5-7-9-11-13-15/h14-15H,1-13H2 | |
Source | PubChem | |
URL | https://pubchem.ncbi.nlm.nih.gov | |
Description | Data deposited in or computed by PubChem | |
InChI Key |
HCEPYODGJFPWOI-UHFFFAOYSA-N | |
Source | PubChem | |
URL | https://pubchem.ncbi.nlm.nih.gov | |
Description | Data deposited in or computed by PubChem | |
Canonical SMILES |
C(CCCCCCO)CCCCCCO | |
Source | PubChem | |
URL | https://pubchem.ncbi.nlm.nih.gov | |
Description | Data deposited in or computed by PubChem | |
Molecular Formula |
C13H28O2 | |
Source | PubChem | |
URL | https://pubchem.ncbi.nlm.nih.gov | |
Description | Data deposited in or computed by PubChem | |
DSSTOX Substance ID |
DTXSID80453878 | |
Record name | 1,13-Tridecanediol | |
Source | EPA DSSTox | |
URL | https://comptox.epa.gov/dashboard/DTXSID80453878 | |
Description | DSSTox provides a high quality public chemistry resource for supporting improved predictive toxicology. | |
Molecular Weight |
216.36 g/mol | |
Source | PubChem | |
URL | https://pubchem.ncbi.nlm.nih.gov | |
Description | Data deposited in or computed by PubChem | |
CAS No. |
13362-52-2 | |
Record name | 1,13-Tridecanediol | |
Source | EPA DSSTox | |
URL | https://comptox.epa.gov/dashboard/DTXSID80453878 | |
Description | DSSTox provides a high quality public chemistry resource for supporting improved predictive toxicology. | |
Retrosynthesis Analysis
AI-Powered Synthesis Planning: Our tool employs the Template_relevance Pistachio, Template_relevance Bkms_metabolic, Template_relevance Pistachio_ringbreaker, Template_relevance Reaxys, Template_relevance Reaxys_biocatalysis model, leveraging a vast database of chemical reactions to predict feasible synthetic routes.
One-Step Synthesis Focus: Specifically designed for one-step synthesis, it provides concise and direct routes for your target compounds, streamlining the synthesis process.
Accurate Predictions: Utilizing the extensive PISTACHIO, BKMS_METABOLIC, PISTACHIO_RINGBREAKER, REAXYS, REAXYS_BIOCATALYSIS database, our tool offers high-accuracy predictions, reflecting the latest in chemical research and data.
Strategy Settings
Precursor scoring | Relevance Heuristic |
---|---|
Min. plausibility | 0.01 |
Model | Template_relevance |
Template Set | Pistachio/Bkms_metabolic/Pistachio_ringbreaker/Reaxys/Reaxys_biocatalysis |
Top-N result to add to graph | 6 |
Feasible Synthetic Routes
Haftungsausschluss und Informationen zu In-Vitro-Forschungsprodukten
Bitte beachten Sie, dass alle Artikel und Produktinformationen, die auf BenchChem präsentiert werden, ausschließlich zu Informationszwecken bestimmt sind. Die auf BenchChem zum Kauf angebotenen Produkte sind speziell für In-vitro-Studien konzipiert, die außerhalb lebender Organismen durchgeführt werden. In-vitro-Studien, abgeleitet von dem lateinischen Begriff "in Glas", beinhalten Experimente, die in kontrollierten Laborumgebungen unter Verwendung von Zellen oder Geweben durchgeführt werden. Es ist wichtig zu beachten, dass diese Produkte nicht als Arzneimittel oder Medikamente eingestuft sind und keine Zulassung der FDA für die Vorbeugung, Behandlung oder Heilung von medizinischen Zuständen, Beschwerden oder Krankheiten erhalten haben. Wir müssen betonen, dass jede Form der körperlichen Einführung dieser Produkte in Menschen oder Tiere gesetzlich strikt untersagt ist. Es ist unerlässlich, sich an diese Richtlinien zu halten, um die Einhaltung rechtlicher und ethischer Standards in Forschung und Experiment zu gewährleisten.